Figures & data
Figure 1 Current field deployable treatments for leishmaniasis. Current field deployable therapies include chemotherapies which are the first-line treatment. Other mechanical therapies such as cryotherapy and thermotherapy can be operated in the field to treat skin lesions. Finally, future treatments include immunotherapies, nanocarriers and repurposing drugs to target the specific cellular niches of the parasite.
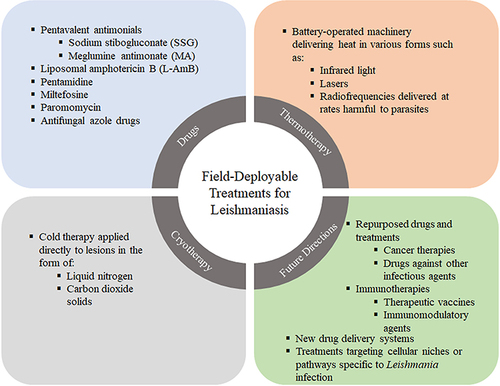
Table 1 Current Treatments Against Leishmaniasis. Administration routes, and therapeutic characteristics including use against which manifestation of leishmaniasis and other diseases, if repurposed
Table 2 Future Directions of Drugs for Leishmaniasis. Summary of Future Therapies That Could Be Repurposed for Their Use Against Leishmaniasis, the Disease for Which It Was Designed, Their State of Development and Testing Against Such Disease and Against Leishmaniasis, Mechanism of Action and Their Potential to Be Beneficial to Different Types of Leishmaniasis
Figure 2 Interplay of Leishmania and its cellular hosts: HSCs and monocytes. Hematopoietic stem cells (HSCs) respond to Leishmania infection. During this process, the microenvironment of the bone marrow (BM) changes, an inflammatory Th1 response usually prevents parasite expansion although infection can lead to local T-cell exhaustion, whereas an abundance of a regulatory environment, leaded by regulatory T cells (T regs) result in an elevated parasitic burden (PB) and is related to drug resistance. The nitric oxide (NO) and reactive oxygen species (ROS) production is also reduced and allows the generation of a permissive environment for the parasite. Although HSCs expand and differentiate to fight the infection, the resulting monocytes become the main host for the parasite, therefore resulting detrimental for the host. These newly differentiated monocytes express IL-10 and arginase (Arg)-1, and a reduction in iNOS and TNF-α, the characteristic phenotype that allows parasite survival. Finally, monocytes play a role by migrating to either the visceral organs in visceral leishmaniasis (VL) or to the skin lesion in cutaneous leishmaniasis (CL) with different roles for each manifestation. All this process can be targeted by the use of different therapies (bold), such as immunotherapies to reinstall the immune balance and favor an inflammatory response which allows parasite killing, also nanocarriers could be used to develop drugs directly to the infected cells. Other drugs limiting the expansion of HSCs could stop the generation of more cellular hosts as the monocytes, whereas migration of monocytes can also be driven into a favorable result for the patient.
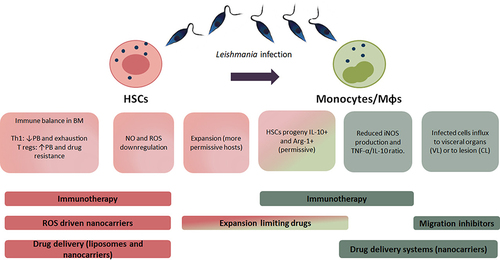
Figure 3 Regulatory pathways involved in ferroptosis. In ferroptosis, PUFAs are oxidized by free radicals due to lipid peroxidation. Hydroxyl facilitates lipid peroxidation, which causes stress-induced cell damage. Ferrous iron catalyzes these lipid hydroperoxides (LOOHs) into ROS molecules, followed by impairing cell membrane integrity. By turning damaging lipid hydroperoxides into lipid alcohols with the help of glutathione (GSH), GPX4 controls ferroptosis. Cystine is crucial for controlling lipid peroxidation by keeping GSH levels stable. SLC7A11 is a key regulator of SystemXc and is responsible for facilitating cystine/glutamate transport. Lipoperoxidation is inhibited by FSP-1, which is a NADPH-dependent coenzyme Q10 oxidoreductase located in cell membranes. Lipophilic radicals are contained within them, stopping the dissemination of lipid peroxide. This figure was created using Servier Medical Art Commons Attribution 3.0 Unported Licence (https://smart.servier.com/).
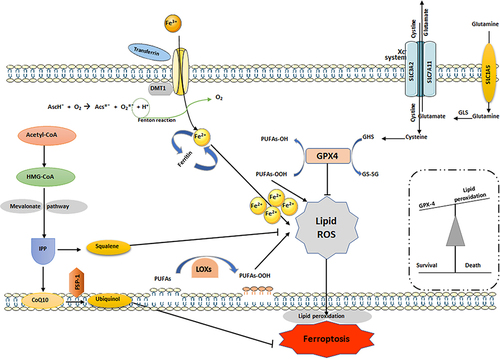