Figures & data
Figure 1 Partial pressure of inspired O2 (PIO2) is decreased in mountainous regions. Representative cities in major mountain ranges are shown. Notes: The map is courtesy of NASA/JPL-Caltech and adapted from NASA/JPL-Caltech. Aster Global Digital Elevation Map (GDEM) . Available at: https://asterweb.jpl.nasa.gov/images/GDEM-10km-colorized.png. Accessed February 28, 2021.Citation210
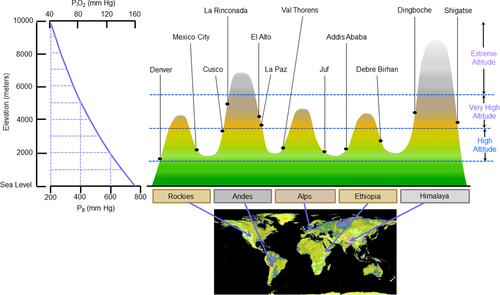
Figure 2 Changes of resting cardiovascular parameters when acutely exposed to high altitude and during acclimatization. Notes: Bbased on data reported in references Citation55 and Citation62–Citation65. From left to right.
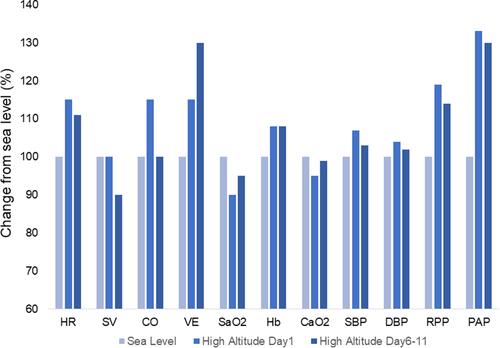
Figure 4 Hypobaric hypoxia induces cardioprotective gene expression. Hypoxia elicits cardioprotective adaptations by activating three gene programs: (A) β-adrenergic activation of cyclic nucleotide response element (CRE) binding protein (CREB) promotes transcription of genes encoding sarcoplasmic reticular Ca2+ ATPase (SERCA) and sarcolemmal Na+/Ca2+ exchanger (NCX), thereby improving Ca2+ homeostasis in the face of ischemia-reperfusion. (B) Intracellular hypoxia attenuates O2-dependent, prolyl hydroxylase (PHD) mediated degradation of the α subunit of hypoxia-inducible factor-1 (HIF-1), which translocates to the nucleus, binds HIF’s β subunit, and activates hypoxia-response elements (HRE) promoting expression of genes encoding hypoxia-adaptive proteins including erythropoietin, vascular endothelial growth factor (VEGF), nitric oxide (NO) synthase (NOS), endothelin-1, glucose transporters (GLUT) and glycolytic enzymes. Erythropoietin and NO suppress inflammation, VEGF promotes coronary collateral formation, endothelin-1 suppresses apoptosis, and GLUT and glycolytic enzymes support anaerobic ATP and phosphocreatine (PCr) production during ischemia. (C) Cellular hypoxia causes electron (e−) accumulation in the mitochondrial respiratory complexes. These electrons combine with residual O2 forming reactive oxygen species (ROS) which oxidize sulfhydryl moieties in Keap1, allowing Nrf2 to activate antioxidant response elements (ARE) in genes encoding antioxidant enzymes, thereby bolstering cellular defenses against ROS overproduction. ROS also augment HIF-1-activated gene expression by blunting HIF-1α degradation. Collectively, these mechanisms increase cardiomyocyte resistance to ischemia-reperfusion induced Ca2+ overload, inflammation, mitochondrial permeability transition (MPT), ATP depletion and oxidative stress.
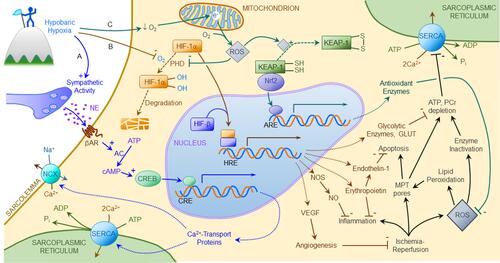