Figures & data
Figure 1. Schematic representation of the early secretory pathway. Nascent proteins are sorted into emerging COPII vesicles after clearing the quality control mechanisms in the endoplasmic reticulum (ER). COPII vesicles mediate anterograde transport via the ER-to-Golgi intermediate compartment (ERGIC) an eventually deliver their cargo at the cis-face of the Golgi apparatus. Here, proteins are either sorted to stay in the stack of membrane cisternae (called cis, medial and trans cisternae) and undergo further anterograde transport towards the trans-Golgi network (TGN), or they are marked to participate in retrograde transport via COPI vesicles. As shown by electron microscopy, many proteins feature non-uniform steady-state distributions along the secretory pathway, indicated here by overlapping color-coded distributions below the organelles. This Figure is reproduced in colour in the online version of Molecular Membrane Biology.
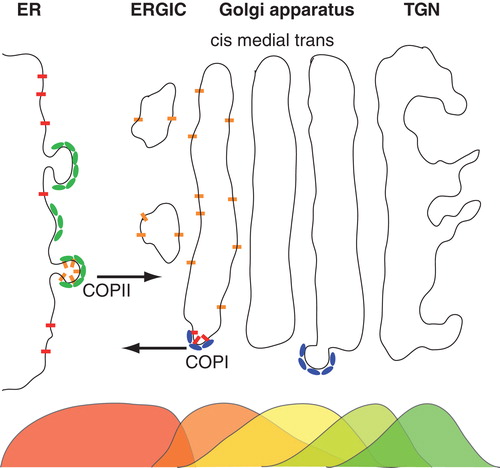
Figure 2. Mismatch-induced clustering of transmembrane proteins. (A) Embedding a transmembrane protein into a homogenous lipid bilayer leads to a local reduction or increase of the membrane thickness h(r); radial distances r from the protein are given in units of the size of a single lipid, r0. Insets show model proteins with a negative/positive hydrophobic mismatch (red and yellow indicate hydrophilic and hydrophobic residues, respectively). (B) A local minimum in the potential of mean force (PMF) between two proteins with a hydrophobic mismatch (given in units of thermal energy kBT) indicates a bound state for small distances. The minimum of the PMF becomes deeper for an increasing mismatch (black to red to blue). For large separations of the proteins no interaction is seen, i.e. the PMF is approximately constant. Inset: The mean first passage time τ to leave the bound state, i.e., to hop out of the minimum of the PMF, increases with the strength of the hydrophobic mismatch. Here, n indicates the length of the transmembrane domain with n <5 denoting a negative and n >5 denoting a positive mismatch. (C) Snapshot of a simulated membrane (grey) with mismatch-induced clusters of transmembrane proteins (red); water is not shown for better visibility. Figure adapted from (Schmidt et al. Citation2008). This Figure is reproduced in colour in the online version of Molecular Membrane Biology.
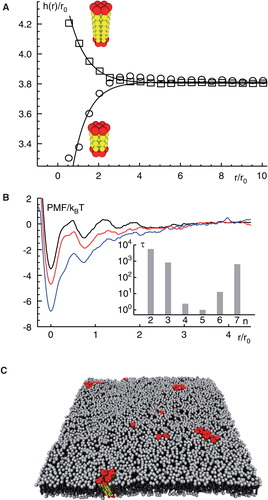
Figure 3. Structure formation of peripheral membrane proteins. (A) PMF for peripheral membrane proteins that reside in the same leaflet of a homogenous lipid bilayer. For large distances, proteins do not interact whereas for small distances a minimum in the PMF emerges that indicates a bound state. The binding strength increases for increasing penetration depths and radii of the proteins. (B) PMF for peripheral membrane proteins that reside in opposing leaflets of a homogenous lipid bilayer. In this case, proteins can form cross-leaflet dimers with a configuration and binding energy that depends again on the penetration depths and radii of the involved proteins. Figure adapted from (Morozova et al. Citation2011). This Figure is reproduced in colour in the online version of Molecular Membrane Biology.
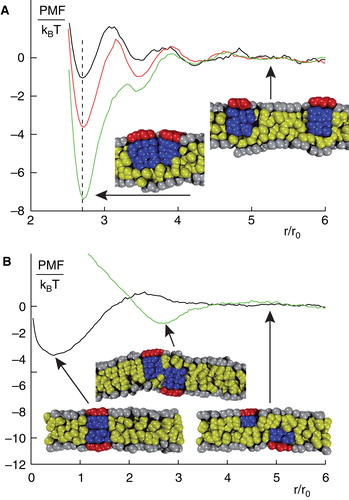
Figure 4. Model for sorting and trafficking facilitated by hydrophobic mismatching. If a membrane protein experiences a negative mismatch (lower left), e.g., in the ER, it will oligomerize and down-modulate the respective coat machinery, e.g., COPII. The emerging vesicle will transport the protein to a new compartment (middle right), e.g., the cis cisterna of the Golgi apparatus. Here, a slightly thicker membrane may extinguish cluster formation due to a lack of hydrophobic mismatching. As a consequence, proteins rarely leave this compartment (indicated by thinner arrows). If the protein experiences a positive mismatch (e.g., during a cisternal maturation process in the Golgi apparatus or after having left the best-matching bilayer by mistake), the protein will again start to form cluster that again will stabilize coat molecules at this locus (e.g., COPI at this time) which facilitates a retrieval to the better matching bilayer. This Figure is reproduced in colour in the online version of Molecular Membrane Biology.
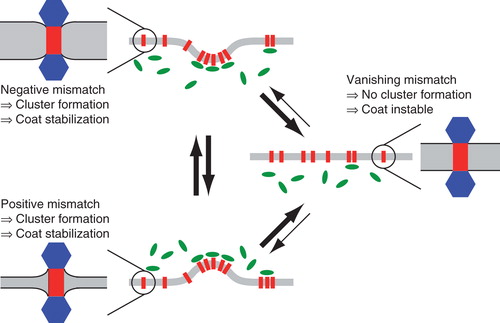