Figures & data
Figure 1. Figure shows the various modifications made in an aptamer to increase its stability and functionality. The common modifications made in the ribose residue of the sugar-phosphate backbone are incorporations of 2´-fluorine (F) and 2´-amino group (NH2), in the phosphate group of the sugar-phosphate backbone are incorporation of phosphorothioate and methyl phosphonate. The modification made in the nucleotide base includes incorporation of 5´- bromide (Br) and amino group (NH2) (Cheung et al., Citation2010). The 3´-capping of the aptamer sequence is also another form of modification, which helps in increasing the stability of aptamer and its prevention from nuclease degradation (Kasahara et al., Citation2010).
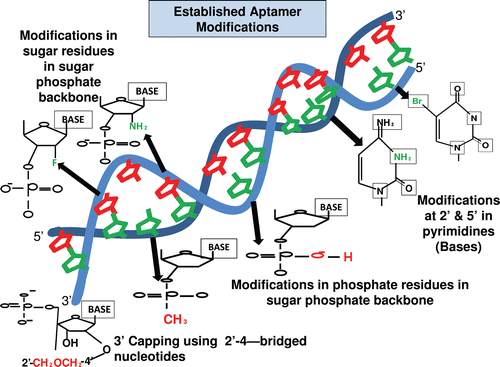
Figure 2. The structure of the locked nucleic acid molecule is shown in . The reason for its stability is due to the presence of an additional bond between the hydrogen on the 4th carbon and the oxygen atom on the 2nd carbon, which also gives the molecule a locked structure and makes it more resistant to thermal degradation. The incorporation of the LNA molecules into the aptamer is also shown which stabilizes the structure, and finally the LNA-incorporated aptamers are loaded onto a nanoparticle for target delivery (DI Primo et al., Citation2007; Lebars et al., Citation2007; Seferos et al., Citation2007; Barciszewski et al., Citation2009).
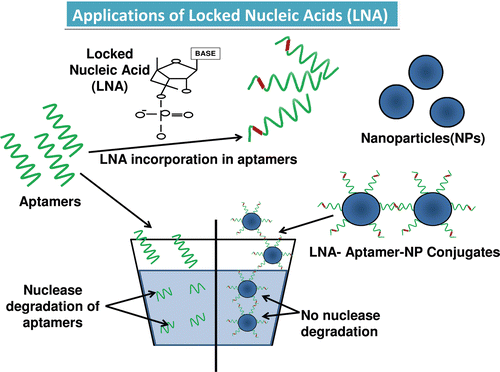
Table 1. Chimeric aptamers and their action.
Figure 3. Illustrations of the various types of chimeric forms of aptamers established, such as the aptamer–antibody (Ohk et al., Citation2010), aptamer–protein (Zhou & Rossi, Citation2010), aptamer–siRNA (Kawata et al., Citation2010) (Pastor et al., Citation2010), aptamer–miRNA (Lunse, Citation2010), and the aptamer–aptamer (Held et al., Citation2006) chimeras. The use of sticky bridges is also depicted which helps in binding of the aptamer moiety to the siRNA and the miRNA’s due to presence of complementary regions on the sticky loop for both siRNA/miRNA and the aptamer. The aptamer conjugates are then shown to conjugate with the nanoparticles binding to the target cells by the interaction of aptamer–receptor interaction, and finally the nanoparticles are internalized inside the target cells and results in release of siRNA, miRNA, protein, or the aptamer molecule, respectively (Lundberg et al., Citation2007; Farokhzad et al., Citation2004; Khaled et al., Citation2005; Alonso et al., Citation1994; Molpeceres et al., Citation1999).
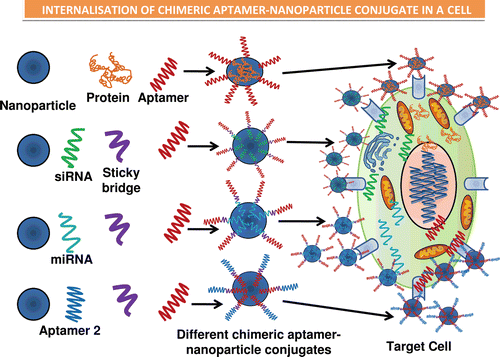
Table 2. Aptamer based detection systems.
Figure 4. Representation of future generation drug delivery by means of nanocapsules that are equipped with real-time imaging and wireless monitoring chips. These nanocapsules can be loaded with several drugs at the same time, and can be taken orally due to lack of any toxic effects. Once present in intestine the nanocapsules can be monitored by the controlling system to release the drugs by opening the valves. The drugs can act specifically on the respective target sites due to presence of various receptors and thus multiple diseases can be targeted at the same time and can be monitored as well in real time. These drugs can be monitored by means of satellite at different places simultaneously hence providing better diagnosis by the experts (Huertas et al., Citation2010; Shen et al., Citation2010).
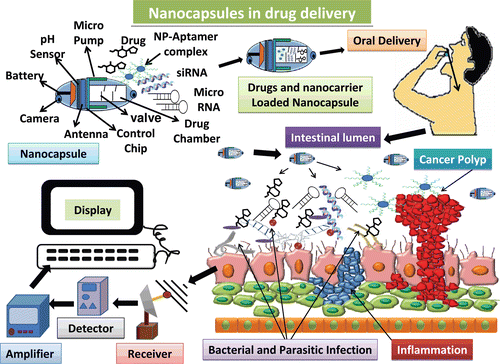
Figure 5. Cell cytotoxicity determined by LDH release assay and cell death (apoptosis) by TUNEL assay of (A) breast cancer cells and (B) colon cancer cells and compared with normal cells following treatment with 1600 μg/mL of iron saturated lactoferrin (Fe-bLf) and other control nanoformulations and control aptamers (nucleolin and EpCAM). Cells were treated for 24 h with different nanoformulations and stained by TUNEL analysis for apoptotic cells. Cell death is shown here in terms of apoptotic (% apoptosis) and LDH release (% cytotoxicity). All treatments were performed in triplicate and assay was repeated three times independently with similar results. The mean for representative experiment was calculated and presented as a mean ± SD values; ** indicates a highly significant p < 0.001 value from the normal control cell lines and with media only; * indicates a significant p < 0.05 value from the normal control cell lines and control with media only, (C) Biodistribution study of nanocarriers compared with Fe-bLf in oral administrations. Fluorescent signal of tissue extracts after 36 h of oral administration. Nanocarriers were labeled with coumarin (10mg/mL).
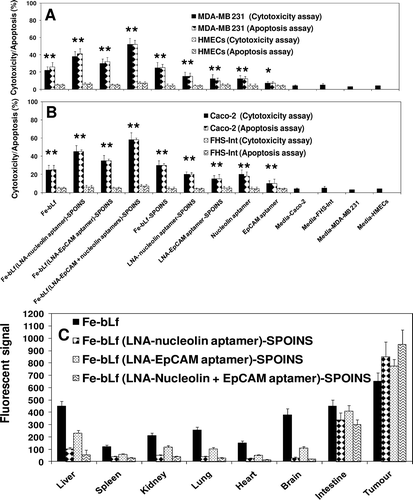