Figures & data
Figure 1. A: Schematic representation of EAF-PEGylation. B: Stability of thiolated albumin against scrambling of the internal disulfide bonds of albumin by thiol disulfide exchange reaction and against intermolecular cross-linking reaction to form oligomers through oxidation of thiols to generate inter-molecular disulfide bonds. A, HSA without IT; b, HSA (0.5 mm) with 5 mM IT incubated overnight (about 6 thiols in the final product); c, HSA (0.5 mM) with 10 mM IT incubated overnight at 4°C (about 12 thiol groups per mole) were subjected to SEC analysis using FPLC equipped with two analytical Superose 12 columns connected in series. C: PEGylation of HSA with 10 mM maleimidophenyl PEG3K in the presence of 5 mM 2-IT as a function of protein concentration ranging from 0.0625 to 1 mM as reflected by SDS-PAGE. D: Extent of PEGylation of HSA with 10 mM maleimidophenyl PEG5K in the presence of 5 mM 2-IT carried out in PBS pH 7.4 overnight at 4°C as a function of protein concentration ranging from 0.0625 (∼ 4 mg/ml) to 1 mM (∼ 66 mg/ml) as reflected by SDS-PAGE (Lane 1; marker, lane 2; control HSA, lane 3; 1 mM, lane 4; 0.5 mM, lane 5; 0.25 mM, lane 6; 0.125 mM and lane 7; 0.0625 mM HSA).
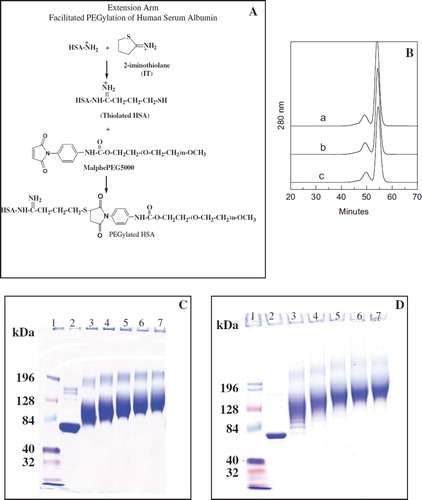
Table 1. PEGylation induced increase in the molecular volume of HSA as a function of number of chains conjugated using EAF-PEGylation with maleimido phenyl PEG (5K and 3K) as the PEGylating reagenta
Figure 2. Packing density of PEG within the PEG-shell as a function of number of PEG-chains conjugated to albumin. A: Increase in the molecular radius of albumin as a function of PEGylation, i.e., number of PEG chains conjugated to albumin. B: Packing density of PEG in the PEG-shell of EAF PEG albumin as a function of number of PEG chains conjugated; note that PEG-3K chains pack much more compactly than PEG5K chains. C: Packing density of PEG in the PEG shell of EAF PEG albumin as a function of total PEG mass conjugated to the protein.
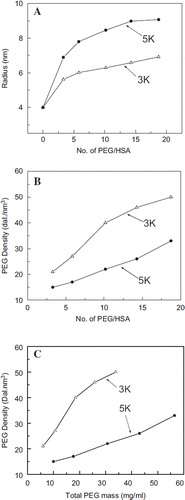
Figure 3. Correlation of the PEGylation induced solution properties (viscosity and Colloidal oncotic pressure) of PEG-albumin adducts as a function of PEGylation. PEG-chains of two different molecular sizes have been used for PEGylation. Viscosity (A) and COP (B) of PEG-albumin were measured at 4 gm % (protein). Inset shows correlation of viscosity (inset in A) and COP (inset in B) as a function of oxy ethylene units to define whether solution properties are just a function of PEG mass.
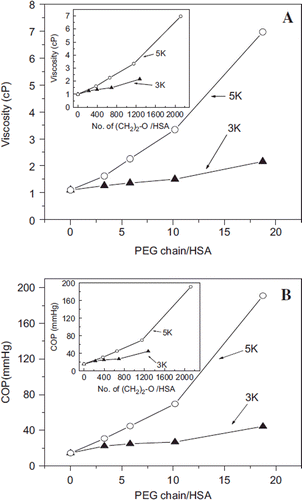
Figure 4. Influence of conjugated and free PEG5K chains on the Trp fluorescence of albumin. A: Fluorescence spectra of EAF PEGylated albumin. Curves a to d represent the fluorescence of HSA conjugated with 0, 3, 6, and 10 copies of PEG5K chains, respectively, in PBS at pH 7.4. The inset in A shows the % quenching (decrease) in the fluorescence of albumin as a function of number of PEG5K chains conjugated. B: Fluorescence spectra of albumin in the presence of free monomethoxy PEG 5K. Curves a, e to h in B represent the fluorescence of HSA in the presence of 0, 5, 10, 15, and 20 equivalents of free PEG5K chains per HSA in PBS at pH 7.4. Note that free PEG in solution increases the fluorescence of HSA, in a PEG concentration-dependent fashion, whereas the conjugated PEG-chains quench the fluorescence of albumin. The inset shows the % increase in the fluorescence of albumin.
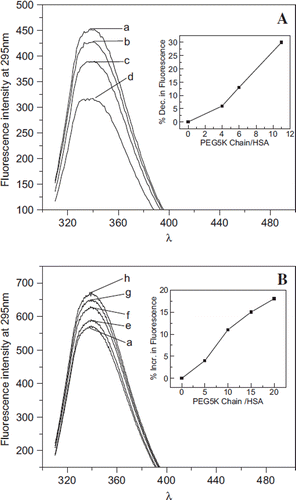
Table 2. Comparison of molecular and solution properties of hexaPEGylated HSA
Figure 5. Influence of extension arms on molecular, solution, and conformational properties of HexaPEGylated albumin. A: Hydrodynamic volume of hexaPEGylated albumin as reflected by SEC: a: HSA; b: EAF-P5K6- HSA; and c: (TCP-PEG5K)6-HSA. B: Apparent molecular mass of hexaPEGylated HSA as reflected by SDS-PAGE: a: HSA; b: EAF-P5K6-HSA; c: (TCP-PEG5K) 6-HSA. C: Influence of extension arm on the secondary structure (overall α-helical conformation) of hexaPEGylated HSA as reflected by Far UV circular dichroism. D: Influence of extension arm on the overall tertiary structure of hexaPEGylated albumin as reflected by tryptophyl fluorescence (a: HSA; b: EAF-P5K6-HSA; c: (TCP-PEG5K)6-HSA).
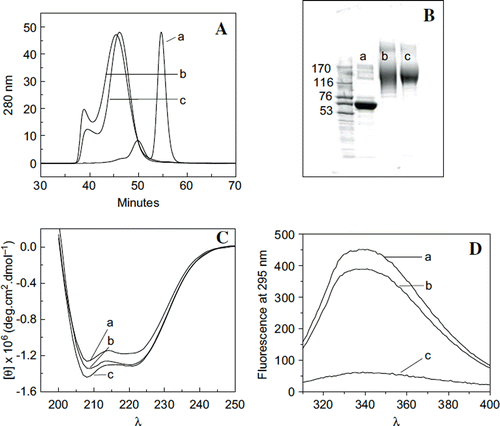
Figure 6. Influence of extension arm on the thermal transition of HexaPEGylated albumin. Scanning microcolorimetry has been used to generate the thermal transition curves. Deconvoluted curves for the experimentally generated transition curves are also shown. A: Control HSA; B: EAF-P5K6-HSA; and C: TCP P5K6-HSA.
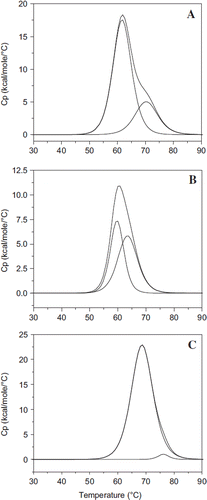
Table 3. Influence of extension arms on the thermal transitions of HexaPEGylated albumin
Figure 7. Viscosity (A) and colloidal osmotic pressure (B) of HSA hexaPEGylated with SP-PEG3K and SP-PEG5K as a function of protein concentration; viscosity (C) and colloidal osmotic pressure (D) of HSA hexaPEGylated with ITPPEG3K and ITPPEG5K as a function of protein concentration. Insets show increased viscosity and COP of the four hexaPEGylated albumins as function of PEG mass.
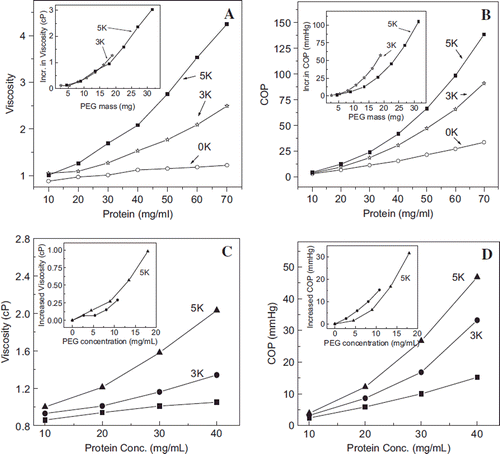
Figure 8. Comparison of the chemistry of conjugation of PEG chains between the EAF mode and the direct mode. The presence of extension arm pushes the PEG-chain away from the protein surface into the bulk water. Thus the interactions of the PEG-chains with the protein molecular surface should be lowered relative to direct PEGylation, and the interactions with the bulk solvent should be increased. Thus the extension arms may be expected to influence and/or modulate the influence of PEGylation on the structure and the functional properties of the PEGylated proteins.
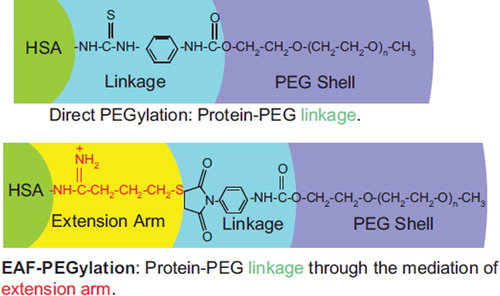