Figures & data
Figure 4 Three scans from viewpoint p1, p2, p3. Set Vi of cell i gives the indices of the viewpoints, whose scans cover cell i. Only one cell (boxed) is covered by all three scans.
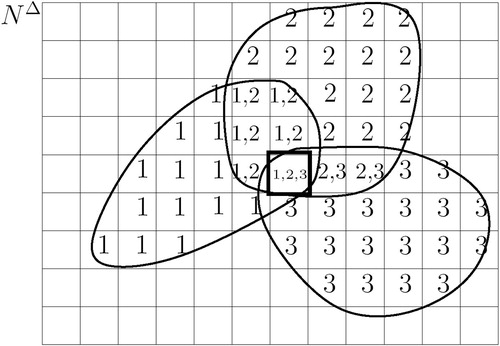
Figure 6 A joint of the FST (frontal view), with u=8, v=1. Each cylinder represents a link in one of 9 (uv+1) joint positions. Note that the center link corresponds to a straight joint position, with a 0° angle between two adjacent links.
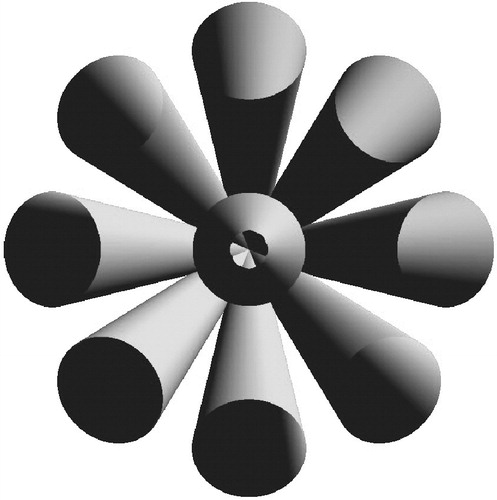
Figure 7 A joint of the FST, with u=4, v=3. Only the centerlines of the links, lying on the surface of concentric cones, are depicted. [Color version available online]
![Figure 7 A joint of the FST, with u=4, v=3. Only the centerlines of the links, lying on the surface of concentric cones, are depicted. [Color version available online]](/cms/asset/888a4abf-952f-463b-b383-66f0e9ac02e5/icsu_a_119017_f0007_b.jpg)
Figure 8 Empirical complexity of a catheter inserted into a brain artery. A fourth-order polynomial was fitted to the observed data from the FST algorithm. A linear fit was found for the data from the ∼FST algorithm. [Color version available online]
![Figure 8 Empirical complexity of a catheter inserted into a brain artery. A fourth-order polynomial was fitted to the observed data from the FST algorithm. A linear fit was found for the data from the ∼FST algorithm. [Color version available online]](/cms/asset/a7315bc0-e9da-41c6-b5f8-e52e529ae3af/icsu_a_119017_f0008_b.jpg)
Figure 10 Landmark-based registration. The insertion depth to the biopsy site can be given as an offset to the insertion depth to the main carina.
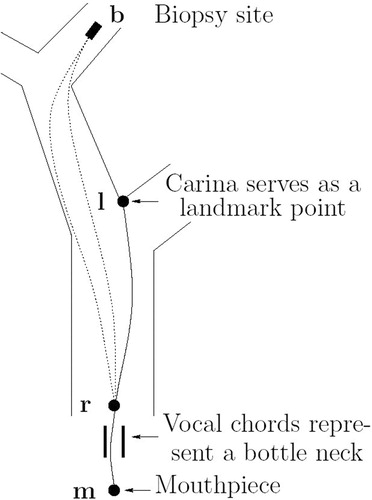
Figure 12 The TBNA protocol, including patient-non-specific instructions and patient-specific parameters (l, αi, βi, di).
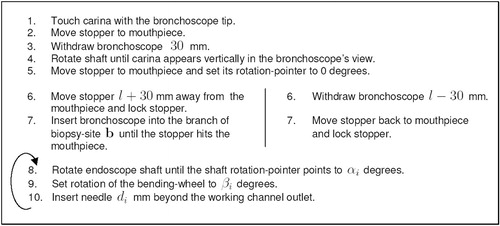
Figure 13 Experimental setup. Olympus GIF-100 with retro-reflective markers inserted into an “M”-shaped calibration path. The endoscope's shape (centerline) was measured in 3D space, using an optical stereo tracking system.
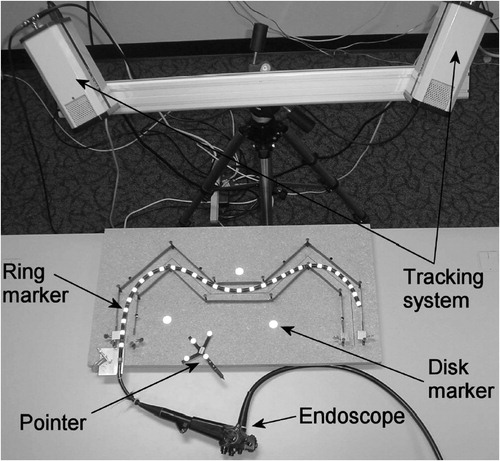
Figure 14 Endoscope model inserted into the calibration path model. Intermediate results (n=44, n′=16, k=2) after the first 13 segments were calculated. All “tentacles” are shown full length. [Color version available online]
![Figure 14 Endoscope model inserted into the calibration path model. Intermediate results (n=44, n′=16, k=2) after the first 13 segments were calculated. All “tentacles” are shown full length. [Color version available online]](/cms/asset/35da36ec-4fce-47f4-b3e1-669ffe82ee00/icsu_a_119017_f0014_b.jpg)
Figure 15 Final result (n=44, n′=20, k=4, p=7), showing only the first k=4 links of each tentacle. For the last segment the energy constraint has been relaxed by computing the p=7 smallest energies. The endoscope model largely matches the measured markers of the real endoscope. [Color version available online]
![Figure 15 Final result (n=44, n′=20, k=4, p=7), showing only the first k=4 links of each tentacle. For the last segment the energy constraint has been relaxed by computing the p=7 smallest energies. The endoscope model largely matches the measured markers of the real endoscope. [Color version available online]](/cms/asset/61703d74-bc38-4f7e-bd64-7484b2ad7d5f/icsu_a_119017_f0015_b.jpg)
Figure 16 Insertion simulation at 12 different stages: After insertion to a depth of 420 mm, the endoscope became stuck due to insufficient branch diameter. [Color version available online]
![Figure 16 Insertion simulation at 12 different stages: After insertion to a depth of 420 mm, the endoscope became stuck due to insufficient branch diameter. [Color version available online]](/cms/asset/9af85251-c5f6-4280-88ee-2aa4e6531825/icsu_a_119017_f0016_b.jpg)
Figure 17 Active bending simulation: How far can the Olympus GIF-100 reach into the upper right lobe of the lung phantom? [Color version available online]
![Figure 17 Active bending simulation: How far can the Olympus GIF-100 reach into the upper right lobe of the lung phantom? [Color version available online]](/cms/asset/13f41443-97ee-48ca-9225-a15b1bf58015/icsu_a_119017_f0017_b.jpg)
Figure 18 The deformation energy decreases from top left to bottom right. [Color version available online]
![Figure 18 The deformation energy decreases from top left to bottom right. [Color version available online]](/cms/asset/882c0d2c-3e93-4934-bfe4-b20927ed0a28/icsu_a_119017_f0018_b.jpg)
Figure 19 Experimental setup, showing the lung and head phantom and the endoscope with the biopsy needle.
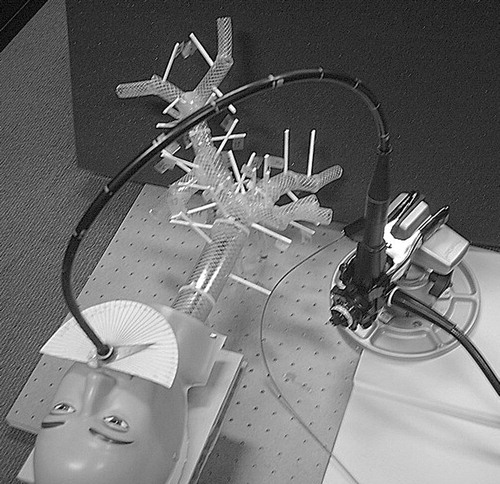
Figure 21 Experimental setup showing the Olympus GIF-100 with reflective markers inserted into the lung phantom.
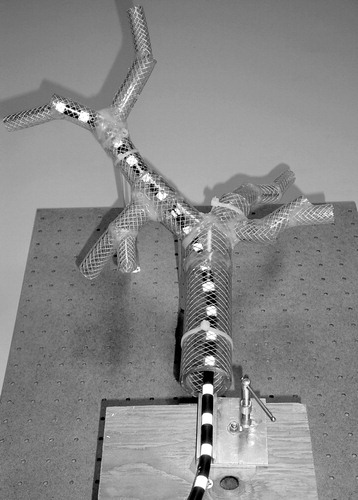
Figure 24 Endoscope model (workspace) inserted into a lung model. Target T is represented by an elliptical point cloud. [Color version available online]
![Figure 24 Endoscope model (workspace) inserted into a lung model. Target T is represented by an elliptical point cloud. [Color version available online]](/cms/asset/39cd9fe1-e65c-4d30-8b68-49af70f2a186/icsu_a_119017_f0024_b.jpg)
Figure 26 Ten scans of TΔ, rendered as lit convex hulls of the respective point clouds. [Color version available online]
![Figure 26 Ten scans of TΔ, rendered as lit convex hulls of the respective point clouds. [Color version available online]](/cms/asset/f6059006-7e09-4b88-bbb6-171ee3f19b05/icsu_a_119017_f0026_b.jpg)
Figure 27 Same scans as in , but each rendered with a 0.5 transparency value (alpha blending). Bending model: ffree. [Color version available online]
![Figure 27 Same scans as in Figure 26, but each rendered with a 0.5 transparency value (alpha blending). Bending model: ffree. [Color version available online]](/cms/asset/8cf4fe8d-1641-4eaf-8597-4bd148dc5ea8/icsu_a_119017_f0027_b.jpg)
Figure 30 Screen shots from the kCP_Greedy() Matlab simulation. Figures (b)–(g) correspond to needles 1–6 in . [Color version available online]
![Figure 30 Screen shots from the kCP_Greedy() Matlab simulation. Figures (b)–(g) correspond to needles 1–6 in Table I. [Color version available online]](/cms/asset/94131120-4d1d-4c2d-9b54-377d4ea9aebb/icsu_a_119017_f0030_b.jpg)
Table I. Probability of success for each needle placed.