Figures & data
Figure 1. (a) The C-arm imaging system: A host PC communicates with the the flat-panel detector (FPI) controller and the PowerMobil (via frame grabber and CANbus, respectively). The FPI controller in turn provides triggering of the X-ray generator in synchronization with FPI readout. Internal to the PowerMobil are a controller and microcomputer interfaced with the X-ray generator and orbital drive. (b) Operative set-up showing a knee specimen positioned near the isocenter of the C-arm with the assistance of back-pointing lasers mounted on the FPI. The magnified view shows one of the specimens following initial incision.
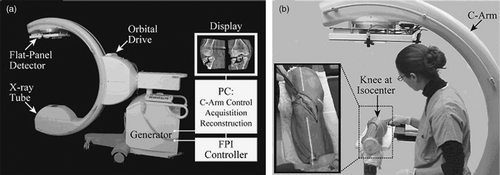
Figure 2. Fracture simulation set-up: Axial loading was applied to the extended knee on an MTS (Bionix 858 materials testing machine). Upon joint closure, confirmed by fluoroscopy, a lateral impact was applied to the specimen to create the tibial plateau fracture.
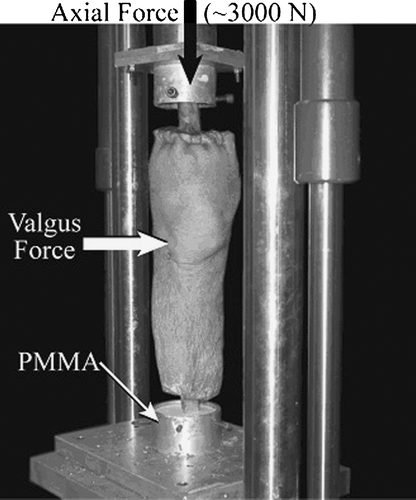
Table I. Details of the simulated tibial plateau fracture patterns.
Table II. Image quality assessment parameters examining acquisition speed and dose.
Figure 3. Case 1. (a) Example image illustrating uniform volumetric image quality and sub-millimetric spatial resolution across a fairly large field of view. (b) Example CBCT image (coronal view) demonstrating the fracture type before reduction. Note the amount of compression and the irregularity of the articular surface.
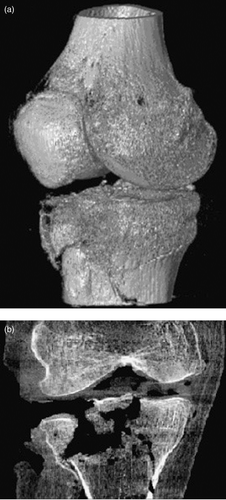
Figure 4. After the first attempt at reduction, orthogonal fluoroscopic views (a and b) were obtained, and the reduction was judged to be acceptable. However, an intraoperative CBCT scan (c) acquired at the same point in the procedure demonstrates a subtle articular fragment that could be reduced further. This fragment and the articular surface were not observed on the fluoroscopy images. (d) CBCT image (coronal view) illustrating improved reduction of the articular surface after further manipulation based on the intraoperative image in (c).
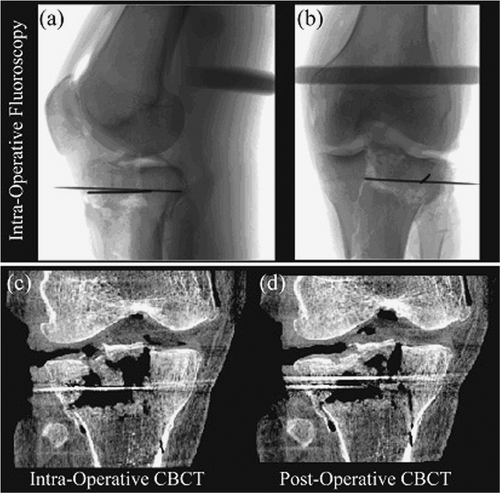
Figure 5. (a) Example coronal image obtained after fracture reduction and placement of a KW in case 2. (b) Localization of the small, displaced fragment was performed using an aluminum KW. The displaced fragment was localized antero-lateral to the KW tip, as shown in the example axial CBCT image. (c) The displaced fragment was manipulated in relation to the aluminum KW, and a near-perfect reduction of the articular surface was achieved under CBCT guidance.
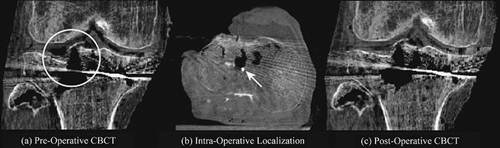
Figure 6. Each column illustrates a CBCT sagittal view, with a magnified portion in the region of the fracture shown in the bottom row. At the nominal dose level (4.7 mGy), excellent delineation of fine, high-contrast structures as well as soft tissues is observed. Below ∼0.5 mGy, image quality rapidly degrades due to increased image noise.
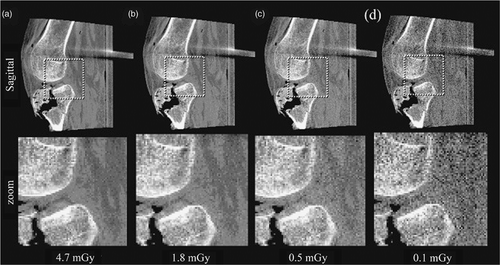
Figure 7. Example images acquired at various speeds and voxel sizes. (a) “Fast” acquisition (200 projections in 60 s) reconstructed at “Nominal” resolution (0.8 mm voxels). (b) “Fast” acquisition reconstructed at “High” resolution (0.4 mm voxels). (c) “Slow” acquisition (500 projections in Citation[1]50 s) reconstructed at “High” resolution. Fracture details are seen in both the Nominal and High resolution reconstructions; however, fine and subtle features are not well delineated in the former. Little appreciable difference is seen between the Fast and Slow acquisition images. Therefore, “Fast/High” (b) gives a reasonable choice of acquisition/reconstruction parameters for the given imaging task.
![Figure 7. Example images acquired at various speeds and voxel sizes. (a) “Fast” acquisition (200 projections in 60 s) reconstructed at “Nominal” resolution (0.8 mm voxels). (b) “Fast” acquisition reconstructed at “High” resolution (0.4 mm voxels). (c) “Slow” acquisition (500 projections in Citation[1]50 s) reconstructed at “High” resolution. Fracture details are seen in both the Nominal and High resolution reconstructions; however, fine and subtle features are not well delineated in the former. Little appreciable difference is seen between the Fast and Slow acquisition images. Therefore, “Fast/High” (b) gives a reasonable choice of acquisition/reconstruction parameters for the given imaging task.](/cms/asset/b484c636-1e94-4197-a7c7-32510d0e57d7/icsu_a_252552_f0007_b.gif)
Figure 8. Example images reconstructed using (a) “Smooth” and (b) “Sharp” reconstruction filters. Overall image quality is superior for the Sharp filter, particularly for identification of subtle fracture components and trabecular structure. The Smooth filter provides somewhat improved delineation of soft-tissue structures.
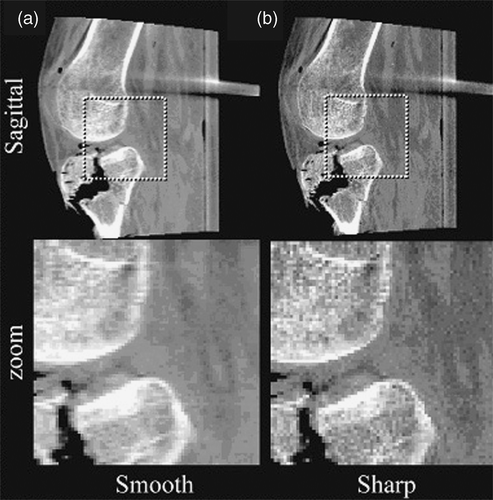
Figure 9. Comparison of image quality between a commercially available system based on an X-ray image intensifier (Iso-C3D) (a and b) and the experimental prototype incorporating a flat-panel detector for CBCT (c and d). Coronal and sagittal views are shown. X-ray technique was matched in terms of kVp, with imaging dose adjusted by variation of tube mA. For the Iso-C3D, the best possible image quality was obtained using a “Slow” acquisition (doubling the number of projections and the imaging dose); imaging dose was estimated at 5 mGy. Images for the experimental system correspond to “Fast” acquisition (fewer projections) and “Nominal” reconstruction (0.8 mm voxels), with an imaging dose of 4.7 mGy.
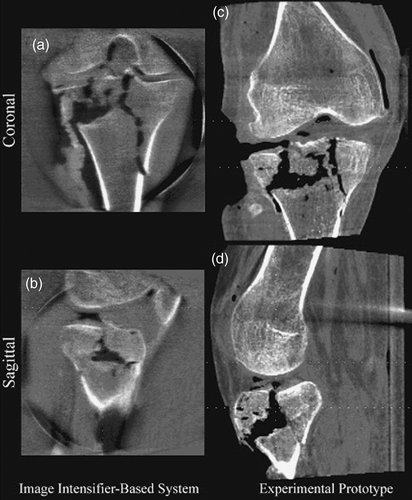