Figures & data
Table 1. The effect on strength of inhibition of altering the structure of isatin (1): a structure–activity study.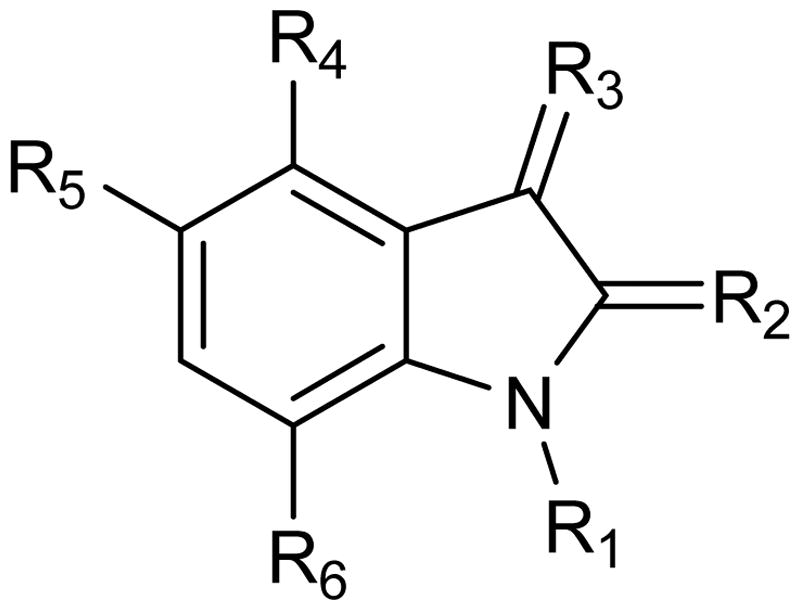
Table 2. The effect of modifications at N1, C2, C3, and the pyrrolidine ring of 8 in the absence of the two chlorines and the resulting Ki values against TDO and IDO1.
Table 3. The importance of the pyrrolidine ring in the inhibition potency of 8.
Figure 2. Stabilization forces between 36 and the active sites of (a) TDO and (b) IDO1 as these defined by LigPlot plus and electrostatic potential analyses. For LigPlot plus analyses (Top), hydrophobic contacts and hydrogen-bond (H-bond) interactions are illustrated as “eyelash” and dashed lines respectively. Compound 36 as well as the H-bond partners are shown as ball-and-stick models. The H-bond distances are in A°. For electrostatic potential analyses (Bottom), 36 and heme are shown as sticks. In both TDO and IDO1 active sites 36 is mainly stabilized by hydrophophic interactions.
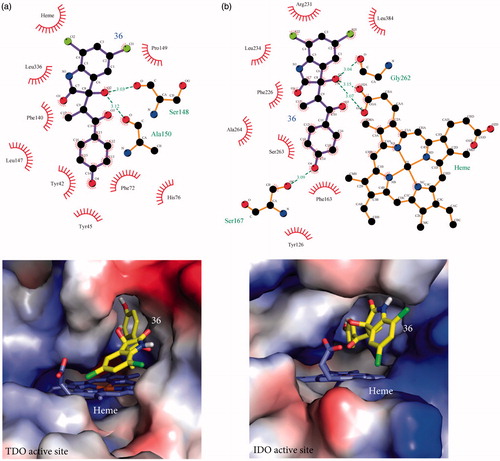
Figure 3. Stabilization forces between 40 and the active sites of (a) TDO and (b) IDO1 as these defined by LigPlot plus and electrostatic potential analyses. For LigPlot plus analyses (Top), hydrophobic contacts and hydrogen-bond (H-bond) interactions are illustrated as “eyelash” and dashed lines respectively. Compound 40 as well as the H-bond partners are shown as ball-and-stick models. The H-bond distances are in A®. For electrostatic potential analyses (Bottom), 40 and heme are shown as sticks. In both TDO and IDO1 active sites 40 is mainly stabilized by hydrophophic interactions.
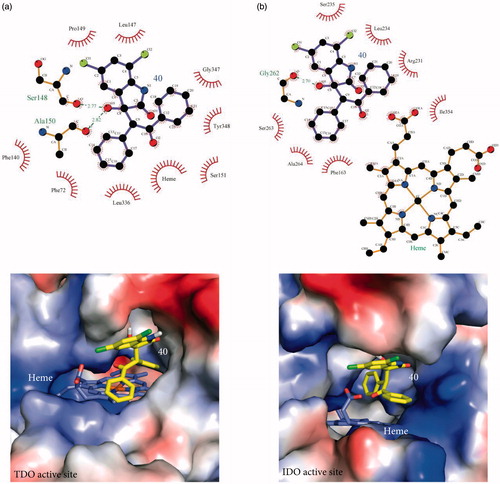
Table 4. Substitutions at C3 and their resulting Ki values against TDO and IDO1.
Table 5. Substitutions at the C3 position in the presence of chlorines and resulting Ki values against TDO and IDO1.
Figure 4. Docking of 5,7-dichloroindolin-2-one chemical moiety of 36 into the active site of hTDO. (a) Superposition of 36 with l-Trp in the docking model of hTDO and crystal structure of XcTDO respectively. The pose of 36 is the most favorable one produced by docking and has similar orientation to that of l-Trp in the crystal structure of XcTDO. (b) The most favorable pose of 36 results in clashes with active site residues of hTDO. Clashes were observed between the C5 chlorine atom of 36 and Leu 147 and C3 hydroxyl group of 36 and Ala 150.
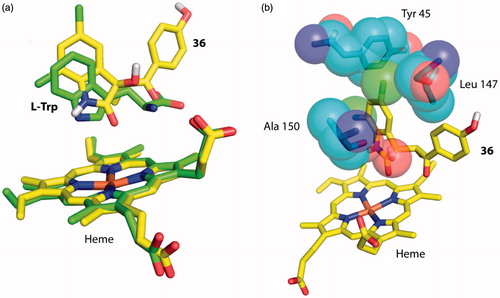