Figures & data
Figure 1. Schematic of the transmembrane structure of Cx32, highlighting the position of the peptides used to generate each of the antibodies assessed. Additional details are provided in .
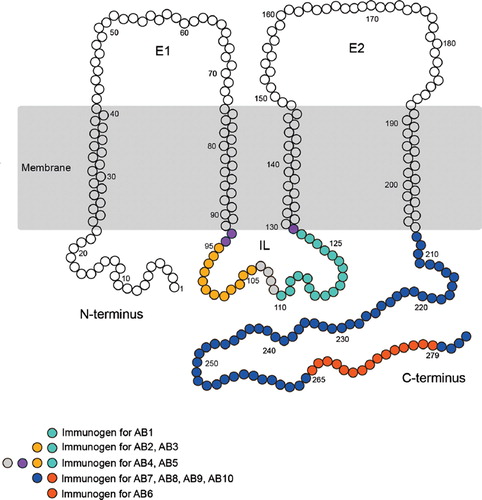
Table 1 Connexin antibodies
Table 2 Antigenic sites along the IL and C-terminus of the Cx32 protein
Figure 2. Four of the five Cx32 antibodies directed against the intracellular loop cross-react with a protein exhibiting the same mobility as Cx32 in Cx32Y/− brain but not Cx32Y/− liver under reducing/denaturing conditions. (A–E, left panel) Transmembrane schematics of the peptides for each Cx32 antibody, with the colored circles representing available information about each immunizing peptide sequence. Black brackets indicate primary sequence with the highest antigenic potential (see ). (A–E, right panel) Cx32 immunoblots were performed using 10 μg liver or 30 μg of human (Hu) and murine hippocampus or brain lysates. Lines indicate size markers. Black arrowheads point to Cx32 specifically detected by all antibodies in liver (A–E) and AB5 in brain (E). White arrowheads indicate isoform variations specific to human samples (D, E). Arrows indicate the cross-reactive protein(s) detected by AB1 to AB4 in both Cx32Y/+ brain tissue and the Cx32Y/− control (A–D). Note that this particular cross-reactive protein(s) migrates with the same mobility as Cx32 when separated on 12.5% Tris-HCl polyacrylamide gels.

Figure 3. Four of the five Cx32 antibodies directed against the C-terminal tail cross-react with a protein exhibiting the same mobility as Cx32 in Cx32Y/− brain but not Cx32Y/− liver under reducing/denaturing conditions. As in , lines indicate size markers. Closed arrowheads point to Cx32 specifically detected by all antibodies in liver (A–E) and AB10 in brain (E). White arrowheads indicate isoform variations specific to human samples (C–E). Arrows indicate the cross-reactive protein(s) detected by AB6-9 in both Cx32Y/+ brain tissue and the Cx32Y/− control (A–D). Note that this particular cross-reactive protein(s) migrates with the same mobility as Cx32 when separated on 12.5% Tris-HCl polyacrylamide gels. All other details are as in .
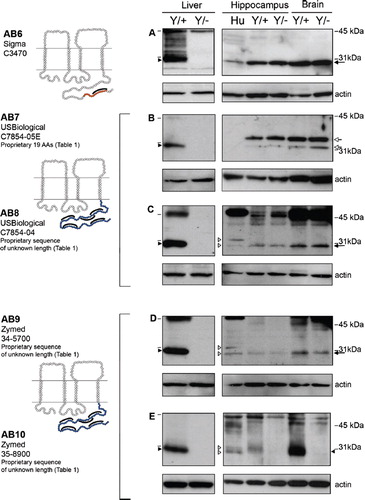
Figure 4. Specific immunofluorescent detection of Cx32 in both liver and brain using intracellular loop-directed antibodies. (A–E) All five antibodies directed against epitopes localizing to the Cx32 intracellular loop detected Cx32 at hepatocyte plasma membrane in Cx32Y/+ liver tissue (arrows, inset, Liver), with minimal to no background reactivity in the Cx32Y/− controls with the exception of some low level background standing of hepatocyte plasma membrane with AB5 (arrowhead, Liver). (A, B, D, E) Four antibodies detected fixed protein in situ in Cx32Y/+ mouse hippocampal sections with (D) AB4 providing the most robust signal. Hippocampal immunostaining was evident at the plasma membrane of cells with expected oligodendrocyte and/or oligodendrocyte precursor cell morphology (arrows, inset, Hippocampus). None of the antibodies exhibited significant cross-reactivity with Cx32Y/− brain tissue. GrDG, granule cell layer of the dentate gyrus; PMNL, polymorphonuclear layer of the dentate gyrus; CA3c, CA pyramidal cell field 3c of the hippocampus. Scale bars, 50 μm.
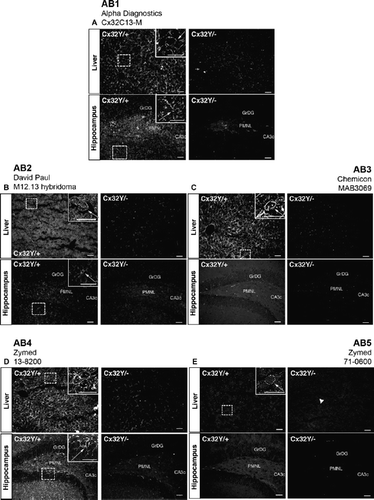
Figure 5. Specific immunofluorescent detection of Cx32 in liver but not brain using C-terminal tail-directed antibodies. (A–E) All five C-terminal antibodies detected Cx32 at hepatocyte plasma membrane in Cx32Y/+ liver tissue (arrows, inset, Liver), with minimal to no background reactivity in the Cx32Y/− controls. No specific immunosignal was seen in the Cx32Y/+ hippocampus under the specific fixation and processing conditions employed in this study. Abbreviations are as in . Scale bars, 50 μm.
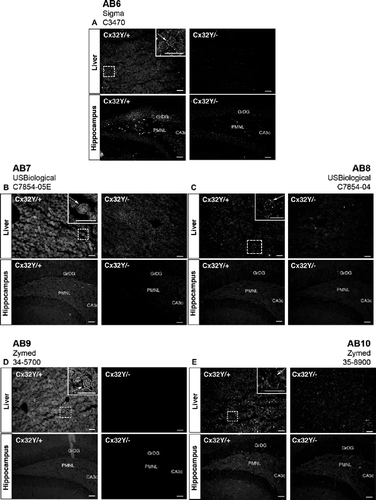
Figure 6. Brain-specific cross-reactivity is not observed following immunoprecipitation. (A) Cx32 lysates were purified from native RIPA lysates using monoclonal AB1, resolved under denaturing conditions by SDS-PAGE, and immunoblotted using polyclonal AB6. In the first two lanes WT (Cx32Y/×) and null-mutant (Cx32Y/−) liver lysates were subjected to Western analysis under reducing/denaturing conditions. The following three lanes represent human hippocampus and murine brain samples from WT (Cx32Y/+) and null-mutant (Cx32Y/−) controls immunoprecipitated with AB1 under native conditions before denaturing/reducing SDS-PAGE separation and immunoblotting with AB6. Specific immunoaffinity purification of Cx32 is observed under these conditions. (B) Standard Western analysis with aliquots of the same samples used in (A) reiterate the cross-reactivity observed when protein is first detected under reducing/denaturing conditions.
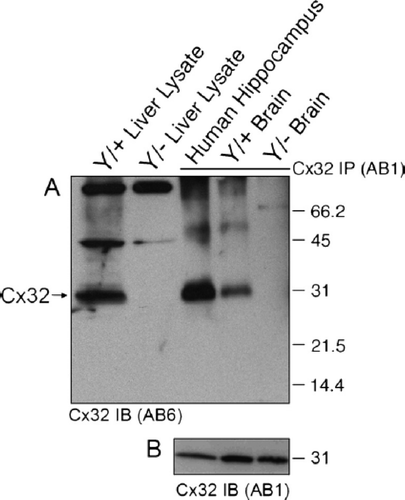
Figure 7. Sucrose gradient fractionation reveals that the brain-specific cross-reactive protein is approximately 4 kDa smaller than Cx32 and exhibits a distinct subcellular localization. (A–D) Total tissue lysates (T) or the Triton X-100–insoluble pellet (P) and (E) fractions 1 to 10 obtained through sucrose fractionation were immunoblotted under denaturing/reducing conditions using AB1. To achieve maximal separation, proteins were separated on 15% Tris-HCl polyacrylamide gels. Each lane contains 5 μg of protein. (A) Sucrose gradient fractions of Cx32Y/+ liver are compared to total tissue lysates of Cx32Y/+ liver or brain. Note the ∽4-kDa size difference between the species predominating in Cx32Y/+ liver compared to brain. (B) Sucrose gradient fractions of Cx32Y/− liver are compared to total tissue lysates of Cx32Y/+ liver or brain. No signal was detected in Cx32Y/− control lysates. (C) Sucrose gradient fractions of Cx32Y/+ brain are compared to total brain lysates prepared from Cx32Y/+ and Cx32Y/− mice (top panel). Exposure times were extended from that shown in A and B to enable detection of endogenous Cx32 in fractions 4 and 5 (arrow), migrating 4 kDa higher than the cross-reactive protein that was present at higher abundance and enriched in fractions 6 to 10. Lower panels characterize each fraction using organelle-specific markers: mitochondria (coxIV), lipid rafts (caveolin-1 and flotillin-1), plasma membrane (syntaxin-1), lysosomes (LAMP-1), and the trans-Golgi network (Golgin-97). The fractionation of liver tissue exhibited the same pattern of organelle-specific immunoreactivity (data not shown). (D) Only the lower molecular weight cross-reactive band was present in sucrose gradient fractions prepared from Cx32Y/− mouse brain. (E) Schematic of the sucrose fractionation showing the gradients enriched for Cx32 in both brain and liver.
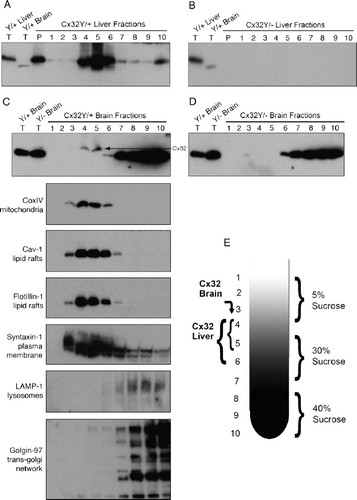
Figure 8. Cx32 antibodies do not cross-react with Cx26, Cx29, or Cx30 under denaturing/reducing conditions. (A) Liver and brain samples were prepared from wild-type (Cx32Y/×), Cx32Y/−, and Cx29−/−. Cx32Y/−/Cx29−/−, or Cx30−/− null-mutant mice, separated on 15% Tris-HCl polyacrylamide gels, and immunoblotted for Cx32 using monoclonal AB1. To distinguish between the cross-reactive protein(s) and endogenous Cx32 in brain samples, fraction 5 lysate from sucrose gradient separations (see ) of WT (Cx32Y/+) was included as a positive control. All brain samples, except WT brain fraction 5, exhibited the lower molecular weight cross-reactive band. Arrowheads indicate Cx32; arrows indicate the cross-reactive (CR) protein. (B) Immunoblotting of Cx30 in lysates prepared from human brain, wild-type mouse brain and liver, and Cx30−/− brain confirmed the presence of Cx30 in CNS tissue (arrowhead), absent from liver. Specificity was established using null-mutant controls. (C) Cx26 protein, present in Cx32Y/+ brain and liver (arrowhead), was below detection levels in Cx32Y/− tissue.
