Figures & data
Figure 1. Comparative graphic overview of the techniques used to resolve structural changes in the human genome. Left is shown the 3 x 109 bp haploid human genome; right is shown each technique together with a vertical bar representing its genome resolution capability. Top plus and minus represent the ability of each technique to provide orientational information. PFGE: pulsed-field gel electrophoresis; FISH: fluorescent in situ hybridization.
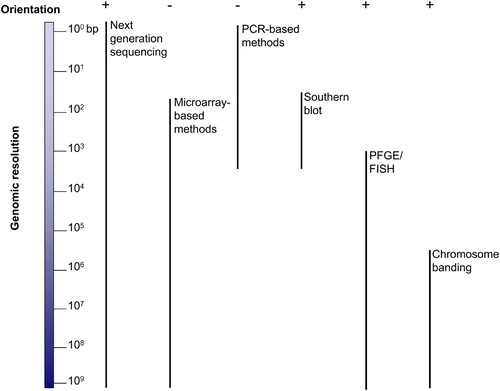
Figure 2. Representational diagram of three types of rearrangements causative for genomic disorders. Arrows (light and dark orange plus brown) represent low copy repeats (LCRs) and light blue rectangles represent a gene or genes spanning the region with altered copy number. Red horizontal bars: duplications (dup); green horizontal bars: deletion; dark blue horizontal bars triplications (trip). Recurrent rearrangements: have the same genomic size and content with breakpoints that cluster within LCRs flanking the altered region. Nonrecurrent rearrangements: are variable in size and genomic content and there is no breakpoint clustering. Nonrecurrent rearrangements with grouping: LCRs mapping close or at the breakpoints are frequently found to ‘group’ at one end. At least three different nonrecurrent rearrangements with grouping have been reported in the literature: i) associated with duplication formation; ii) associated with triplication embedded in duplications and, iii) associated with isodicentric chromosome formation. The two latter examples have been consistently associated with inverted repeats or palindromes [Carvalho et al. Citation2009; Lange et al. Citation2009].
![Figure 2. Representational diagram of three types of rearrangements causative for genomic disorders. Arrows (light and dark orange plus brown) represent low copy repeats (LCRs) and light blue rectangles represent a gene or genes spanning the region with altered copy number. Red horizontal bars: duplications (dup); green horizontal bars: deletion; dark blue horizontal bars triplications (trip). Recurrent rearrangements: have the same genomic size and content with breakpoints that cluster within LCRs flanking the altered region. Nonrecurrent rearrangements: are variable in size and genomic content and there is no breakpoint clustering. Nonrecurrent rearrangements with grouping: LCRs mapping close or at the breakpoints are frequently found to ‘group’ at one end. At least three different nonrecurrent rearrangements with grouping have been reported in the literature: i) associated with duplication formation; ii) associated with triplication embedded in duplications and, iii) associated with isodicentric chromosome formation. The two latter examples have been consistently associated with inverted repeats or palindromes [Carvalho et al. Citation2009; Lange et al. Citation2009].](/cms/asset/f914f037-5820-4183-a241-a6c8804fb6ea/iaan_a_527427_f0002_b.jpg)
Figure 3. Three main mechanisms that account for structural variations observed in association with human genomic disorders. (a) NAHR: non-allelic homologous recombination. This repair mechanism of double-strand breaks occurs by unequal crossing over using paralogous repeats (LCRs) as substrate rather than allelic homologous sequences. The resulting product will ultimately depend on the direction of the repeats involved as well as if the rearrangement occurs via an intrachromatidal, interchromatidal, or interchromosomal event (for an extensive review see [Stankiewicz and Lupski Citation2002]). This figure represents NAHR between two LCRs in the same orientation producing duplication (dup) and deletion (del) of the sequence between the repeats. Intrachromatid NAHR between inverted repeats will produce either inversion of the segment in between the LCRs or gene conversion; interchromatid NAHR (between sister chromatids) will produce either an isodicentric (idic) chromosome plus an acentric fragment or gene conversion [Lange et al. Citation2009]. (b) Non-homologous end joining (NHEJ) repair mechanism is used by eukaryotic cells to repair double-strand breaks. The NHEJ process includes detection of DSBs, molecular bridging of both broken DNA ends; modification of the ends to make them compatible and ligatable, and the final ligation step. If this latter step is not accurate it leaves an ‘information scar’ at the rejoining site as the pre-rejoining editing of the ends includes cleavage or addition of nucleotides [Gu et al. Citation2008; Hastings et al. Citation2009b]. Two rearrangements can be produced by NHEJ after two DSB events: an intrachromosomal deletion and an interchromosomal duplication. (c) Microhomology-mediated break-induced replication (MMBIR): the replication fork collapses because the fork encounters a nick on a template strand. The 5′ end of the broken molecule is recessed from the break, exposing a 3′ tail that may anneal to any single-strand DNA that shares microhomology resuming the replication by priming on another template (a nearby fork might provide the necessary ssDNA). The initial polymerase extension and replication is performed by a low processivity polymerase which may allow multiple rounds, or iterations, of disengagement and template switching until a fully processive replication is established to the end of the chromosome or replicon. As a result, complexities at the breakpoint junctions are predicted as well as rearrangements such as deletions, duplications, inversions, or chromosomal translocations depending on the genomic localization of the reinitiating fork. Absence of heterozygosity downstream of the event is predicted if the switching event occurred between homologous chromosomes rather than between sister chromatids [Hastings et al. Citation2009b].
![Figure 3. Three main mechanisms that account for structural variations observed in association with human genomic disorders. (a) NAHR: non-allelic homologous recombination. This repair mechanism of double-strand breaks occurs by unequal crossing over using paralogous repeats (LCRs) as substrate rather than allelic homologous sequences. The resulting product will ultimately depend on the direction of the repeats involved as well as if the rearrangement occurs via an intrachromatidal, interchromatidal, or interchromosomal event (for an extensive review see [Stankiewicz and Lupski Citation2002]). This figure represents NAHR between two LCRs in the same orientation producing duplication (dup) and deletion (del) of the sequence between the repeats. Intrachromatid NAHR between inverted repeats will produce either inversion of the segment in between the LCRs or gene conversion; interchromatid NAHR (between sister chromatids) will produce either an isodicentric (idic) chromosome plus an acentric fragment or gene conversion [Lange et al. Citation2009]. (b) Non-homologous end joining (NHEJ) repair mechanism is used by eukaryotic cells to repair double-strand breaks. The NHEJ process includes detection of DSBs, molecular bridging of both broken DNA ends; modification of the ends to make them compatible and ligatable, and the final ligation step. If this latter step is not accurate it leaves an ‘information scar’ at the rejoining site as the pre-rejoining editing of the ends includes cleavage or addition of nucleotides [Gu et al. Citation2008; Hastings et al. Citation2009b]. Two rearrangements can be produced by NHEJ after two DSB events: an intrachromosomal deletion and an interchromosomal duplication. (c) Microhomology-mediated break-induced replication (MMBIR): the replication fork collapses because the fork encounters a nick on a template strand. The 5′ end of the broken molecule is recessed from the break, exposing a 3′ tail that may anneal to any single-strand DNA that shares microhomology resuming the replication by priming on another template (a nearby fork might provide the necessary ssDNA). The initial polymerase extension and replication is performed by a low processivity polymerase which may allow multiple rounds, or iterations, of disengagement and template switching until a fully processive replication is established to the end of the chromosome or replicon. As a result, complexities at the breakpoint junctions are predicted as well as rearrangements such as deletions, duplications, inversions, or chromosomal translocations depending on the genomic localization of the reinitiating fork. Absence of heterozygosity downstream of the event is predicted if the switching event occurred between homologous chromosomes rather than between sister chromatids [Hastings et al. Citation2009b].](/cms/asset/b66985c6-5160-4898-ad1c-4edb8641709b/iaan_a_527427_f0003_b.jpg)
Figure 4. Schematic representation of the Y chromosome. Black horizontal line, male-specific region of the Y chromosome (MSY). The horizontal rectangle represents the Y chromosome with its short (Yp) and long (Yq) arms. In red, pseudoautosomal regions (PAR1 and PAR2); in black, heterochromatic regions; arrows in light blue represent palindromes or inverted repeats. (a) Major deletions observed in infertile males: AZFa, P5/proximal-P1 (AZFb), P5/distal-P1, P4/distal-P1, AZFc. The green horizontal lines (above) delimit the regions according to the reference genome (adapted from [Repping et al. Citation2002; Skaletsky et al. Citation2003]); the purple arrows on top of the green bars represent the orientation and location of the LCRs shown to mediate AZF deletions through NAHR. (b) Other structural variations involving the Y chromosome. In sharp blue, regions that show length variation within worldwide populations; in light purple (below), regions that show any other kind of variation such as inversions (inv), partial deletions(del), and duplications (dup) (adapted from [Repping et al. Citation2006]). Figure not to scale.
![Figure 4. Schematic representation of the Y chromosome. Black horizontal line, male-specific region of the Y chromosome (MSY). The horizontal rectangle represents the Y chromosome with its short (Yp) and long (Yq) arms. In red, pseudoautosomal regions (PAR1 and PAR2); in black, heterochromatic regions; arrows in light blue represent palindromes or inverted repeats. (a) Major deletions observed in infertile males: AZFa, P5/proximal-P1 (AZFb), P5/distal-P1, P4/distal-P1, AZFc. The green horizontal lines (above) delimit the regions according to the reference genome (adapted from [Repping et al. Citation2002; Skaletsky et al. Citation2003]); the purple arrows on top of the green bars represent the orientation and location of the LCRs shown to mediate AZF deletions through NAHR. (b) Other structural variations involving the Y chromosome. In sharp blue, regions that show length variation within worldwide populations; in light purple (below), regions that show any other kind of variation such as inversions (inv), partial deletions(del), and duplications (dup) (adapted from [Repping et al. Citation2006]). Figure not to scale.](/cms/asset/ad58280a-8554-4693-bb9a-646d5f2ebf6f/iaan_a_527427_f0004_b.jpg)