Figures & data
Figure 1. Levels of APP and LAMP-2 in RA-differentiated SH-SY5Y neuroblastoma cells stably transfected with vector, APPwt or APPswe. (A) Representative western blots for APP (using the 6E10 antibody), LAMP-2 and actin (loading control). (B) Quantification of APP and LAMP-2 band optical densities normalized against actin levels. The intracellular content of both APP and LAMP-2 was significantly increased in APPwt and APPswe cells as compared with vector controls (*p < 0.05; n = 3). (C) Confocal microscopy images of SH-SY5Y cells immunostained for APP (red fluorescence). Nuclei were stained by DAPI (blue fluorescence). APP immunoreactivity was apparently higher in APPwt and APPswe cells as compared with vector controls (n = 3). Bar, 20 μm.
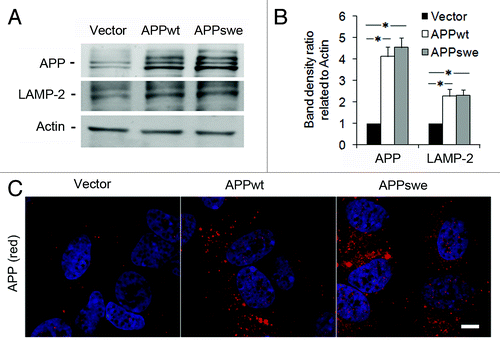
Figure 2. Increased amounts of intracellular APP and Aβ, and decreased amounts of secreted αAPP in RA- differentiated SH-SY5Y cells exposed to hyperoxia or normoxia (40% O2 vs. 8% O2) for 5 d. (A) Representative western blots for holoAPP (probes with 6E10 antibodies) and actin (loading control). (B) Quantification of APP bands normalized against actin levels. The content of APP significantly increased in APPwt and APPswe cells after exposure to hyperoxia (*p < 0.05; n = 4). (C) Representative western blot and quantification of αAPP (probed with 6E10 antibodies) in conditioned media from cell cultures (*p < 0.05; n = 4). (D) The ratio of secreted αAPP levels to holoAPP significantly decreased in APPwt and APPswe cells (*p < 0.05; n = 4), suggesting a decreased α-secretase activity after exposure to hyperoxia. (E and F) Confocal microscopy images of APPswe cells exposed to normoxia or hyperoxia and immunostained for (E) Aβ1–42 (red fluorescence) or (F) Aβ oligomers (using A11 antibody, red fluorescence). Nuclei were stained by DAPI (blue fluorescence). Note the increased immunoreactivity in hyperoxia-exposed cells for both Aβ1–42 and Aβ oligomers (n = 3). Bars, 30 μm.
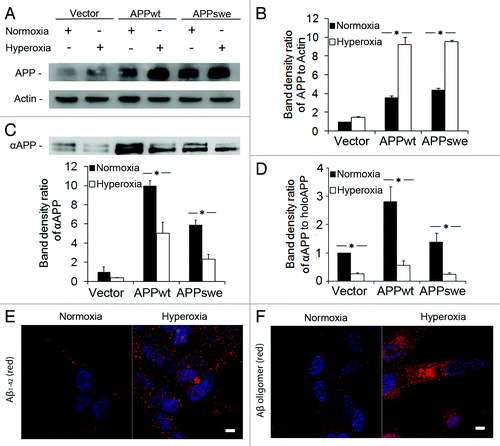
Figure 3. Inhibition of macroautophagy prevented apoptosis induced by APP overexpression and hyperoxia. RA-differentiated SH-SY5Y cells transfected with vector, APPwt and APPswe were investigated under hyperoxia and normoxia (40% O2 vs. 8% O2) for 5 d, with or without the autophagic sequestration inhibitor 3MA (1 mM). (A) Intracellular amounts of ROS were measured by DCF oxidation using flow cytometry. ROS levels increased after exposure to hyperoxia, most significantly in APPswe cells. (*p < 0.05; n = 4). (B) Percentage of cells with condensed or fragmented nuclei (assessed by DAPI staining) increased in all three cell lines after hyperoxia exposure for 5 d (*p < 0.05; n = 3), and were the highest in APPswe cells (Vector < APPwt < APPswe). This effect of hyperoxia was prevented by 3MA. (C) Images of DAPI-stained nuclei show normal and fragmented (apoptotic) nuclei in APPswe cells cultured in normoxia and hyperoxia, respectively. Arrow indicates nuclear fragmentation. Bar, 5 μm. (D) Transmission electron microscopy of APPswe cells shows nuclear fragmentation after exposure to hyperoxia. Bar, 2 μm. (E) Phase contrast images of vector-transfected, APPwt and APPswe cells cultured in hyperoxia, with or without 3MA for 7 d. Hyperoxia-exposed cultures show increased numbers of dead cells. This effect was inhibited by 3MA in all cell lines (compare with B). Experiments were performed 3 times. Bar, 100 μm.
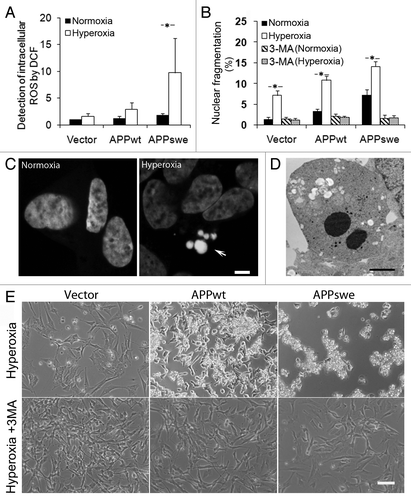
Figure 4. Enhancement of macroautophagy by overexpression of APP and hyperoxia. Vector-transfected, APPwt and APPswe SH-SY5Y cells were exposed to hyperoxia or normoxia (40% O2 vs. 8% O2) for 5 d. (A) Western blotting of LAMP-2 and actin (loading control). (B) Densitometry of LAMP-2 (normalized to actin levels) indicates significant upregulation of the lysosomal system in all cell lines exposed to hyperoxia, particularly in APPwt and APPswe cells (*p < 0.05; n = 3). (C) Western blotting of LC3 and actin (loading control). (D) Densitometry of LC3-II (vs. actin) showed significant activation of macroautophagy by hyperoxia in all cell lines, particularly in APPwt and APPswe cells (*p < 0.05; n = 5). (E) Western blotting of total and phosphorylated p70S6K (pThr389). Actin was used as loading control. (F) P-p70S6K to total p70S6K densitometry ratio was decreased in APPwt and APPswe cells in both normoxia and hyperoxia (*p < 0.05; n = 4).
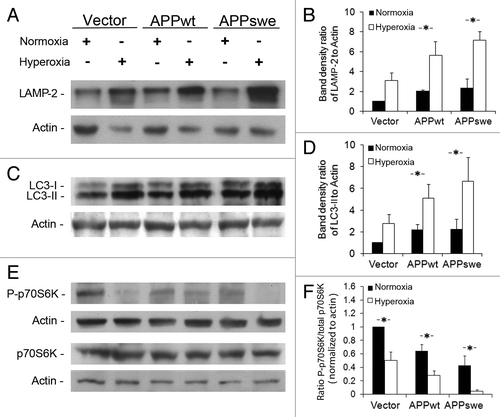
Figure 5. Autophagy flux measurements suggest enhancement of macroautophagy. (A) Western blotting of LC3 in cells cultured with or without lysosomal enzyme inhibitors E64d (10 μM) and Pepstatin A (Pep.A; 10 μg/ml) in normoxic conditions. (B) Increased LC3-II levels (normalized to actin) after lysosomal enzyme inhibition (*p < 0.05; n = 4). (C) Western blotting of LC3 in cells cultured with or without lysosomal enzyme inhibitors E64d (10 μM) and Pepstatin A (10 μg/ml) in hyperoxic conditions [same groups as in (A)]. (D) Increased LC3-II levels (normalized to actin) after lysosomal enzyme inhibition (*p < 0.05; n = 4). The elevated levels of LC3-II after lysosomal enzyme inhibition indicate an increased autophagic flux.
![Figure 5. Autophagy flux measurements suggest enhancement of macroautophagy. (A) Western blotting of LC3 in cells cultured with or without lysosomal enzyme inhibitors E64d (10 μM) and Pepstatin A (Pep.A; 10 μg/ml) in normoxic conditions. (B) Increased LC3-II levels (normalized to actin) after lysosomal enzyme inhibition (*p < 0.05; n = 4). (C) Western blotting of LC3 in cells cultured with or without lysosomal enzyme inhibitors E64d (10 μM) and Pepstatin A (10 μg/ml) in hyperoxic conditions [same groups as in (A)]. (D) Increased LC3-II levels (normalized to actin) after lysosomal enzyme inhibition (*p < 0.05; n = 4). The elevated levels of LC3-II after lysosomal enzyme inhibition indicate an increased autophagic flux.](/cms/asset/7efc5d45-1907-4e3e-a54d-d528d287d46d/kaup_a_10918051_f0005.gif)
Figure 6. Electron micrograghs of autophagic vacuoles in RA-differentiated SH-SY5Y cells. Vector-transfected (A, C and E) and APPswe (B, D and F) cells were cultured in normoxia (A and B), hyperoxia (C and D), or hyperoxia combined with 3MA exposure (E and F), respectively. Exposure to hyperoxia dramatically increased the number and size of autophagic vacuoles in all cell lines, especially in APPswe cells. This effect of hyperoxia was prevented by 3MA (1 mM). AV, autophagic vacuoles; Mt, mitochondria; N, nucleus. Bar, 1 μm. n = 3.
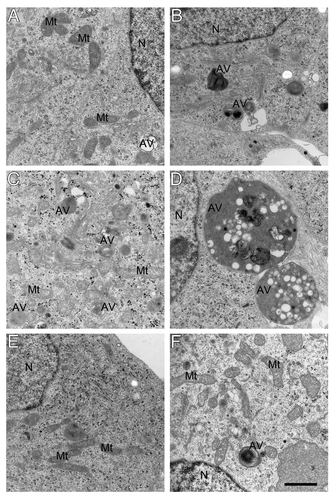
Figure 7. APP accumulation in autophagic-lysosomal compartment was enhanced by hyperoxia and prevented by 3MA (1 mM). (A) Confocal microscopy images of APPswe cells exposed to hyperoxia and double immunostained for APP (red fluorescence) and LC3 (green fluorescence). Nuclei were stained by DAPI (blue fluorescence). Arrows indicate colocalization of APP and autophagic vacuoles. Bar, 50 μm. (B) Confocal microscopy images of APPswe cells exposed to hyperoxia and double immunostained for APP (red fluorescence) and for cathepsin-D (green fluorescence). Nuclei were stained by DAPI (blue fluorescence). Arrows show colocalization of APP and lysosomes. Bar, 30 μm. (C) Quantification of cells with APP containing autophagic vacuoles in SH-SY5Y neuroblastoma cultures exposed to normoxia or hyperoxia, with or without 3MA. The percentage of cells with APP-containing autophagic vacuoles was higher in APP-overexpressing cells than in vector controls (Vector < APPwt < APPswe, p < 0.05; n = 4). Hyperoxia significantly increased the percentage of cells with APP containing autophagic vacuoles in all three cell lines, an effect that was prevented by 3MA treatment, (*p < 0.05; n = 4). (D) Quantification of cells with intralysosomal accumulation of APP. Cells overexpressing APP showed increased numbers of APP-positive lysosomes (Vector < APPwt < APPswe, *p < 0.05; n = 4) under normoxia or hyperoxia.
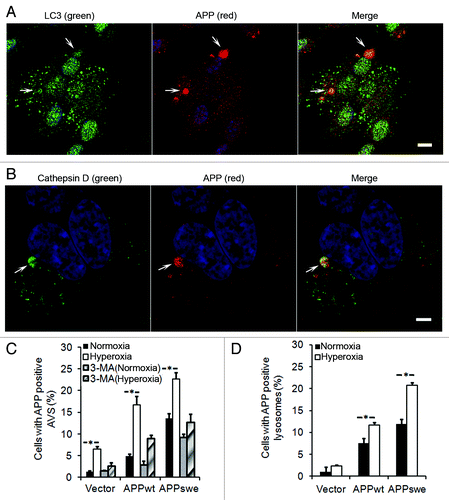
Figure 8. Intralysosomal accumulation of Aβ was enhanced by hyperoxia (5-d exposure) and prevented by 3MA (1 mM). (A) Confocal microscopy images of APPswe cells double immunostained for Aβ1–42 (red fluorescence) and LAMP-2 (green fluorescence). Nuclei were stained by DAPI (blue fluorescence). Arrows indicate the colocalization of Aβ1–42 and lysosomes. Bar, 50 μm. (B) Confocal microscopy images of APPswe cells double immunostained for Aβ oligomers (A11 antibody, red fluorescence) and LAMP-2 (green fluorescence). Nuclei were stained by DAPI (blue fluorescence). Arrows show the colocalization of Aβ oligomers and lysosomes. Bar, 20 μm. (C) Quantification of cells containing intralysosomal Aβ1–42 in cultures exposed to normoxia or hyperoxia, with or without 3MA. The percentage of cells with Aβ-containing lysosomes was higher in APP-overexpressing cells than in vector controls (Vector < APPwt < APPswe, p < 0.05). Hyperoxia significantly increases the percentage of cells with Aβ-positive lysosomes in all three cell lines and this increase was prevented by 3MA. (*p < 0.05; n = 4). (D) Quantification of cells with intralysosomal accumulation of Aβ oligomers. Cells overexpressing APP showed increased numbers of Aβ-positive lysosomes (Vector < APPwt < APPswe). Hyperoxia significantly increased the percentage of cells with lysosomes containing Aβ oligomers in all three cell lines and this increase was prevented by 3MA. (*p < 0.05; n = 4). (E) Quantification of cells containing intralysosomal Aβ1–42 in cultures exposed to normoxia or hyperoxia, with or without lysosomal inhibitors (E64d and Pepstatin A) (*p < 0.05; n = 3).
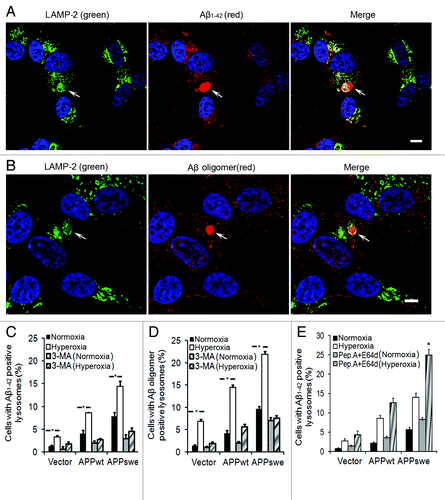
Figure 9. Downregulation of ATG5 by siRNA protected cells from apoptosis and decreased the intralysosomal accumulation of APP and Aβ. (A) Western blot of ATG5 in APPswe cells (control), cells with scramble RNA transfection (scRNA) or ATG5 siRNA transfection. (B) Single immunostaining of LC3 (green fluorescence) in APPswe cells (control), cells with scRNA, and with ATG5 siRNA after 3 d exposure to hyperoxia. Both control and scRNA cells showed brighter and more granular staining of LC3 compared with ATG5 siRNA cells. Bar, 40 μm. (n = 3). Nuclei were stained by DAPI (blue fluorescence). (C–F) Vector and APPswe cells (controls), cells with scRNA, or ATG5 siRNA were exposed to normoxia and hyperoxia for 3 d. The number of cells with: nuclear fragmentation (C) and with APP (D), Aβ1–42 (E), or Aβ oligomer (F) -positive lysosomes were counted. ATG5 siRNA protected cells from death (C), and decreased lysosomal accumulation of APP (D), Aβ1–42 (E), and Aβ oligomers (F) (*p < 0.05; n = 3).
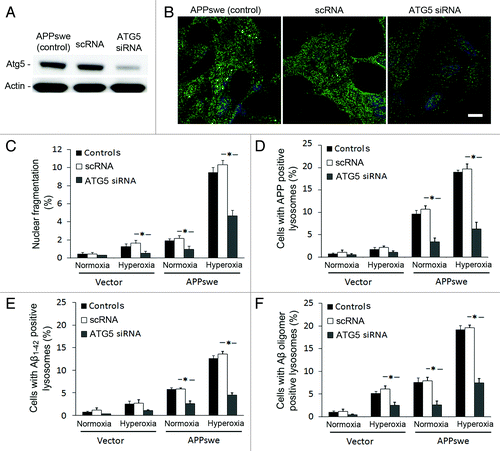
Figure 10 (See opposite page). Effects of γ-secretase inhibition by DAPT on intralysosomal accumulation of Aβ1–42. (A) double immunostaining of LAMP-2 (green) and Aβ1–42 (red) in APPswe cells after 3 d exposure to hyperoxia only (controls), 3 d exposure to hyperoxia and DAPT (500 nM) or 1 d DAPT pretreatment followed by 3 d exposure to hyperoxia and DAPT . Arrows show colocalization of Aβ1–42 with lysosomes while arrowheads indicate enlarged lysosomes without any Aβ1–42 accumulation. After DAPT treatment, the general amount of Aβ1–42 (cytosolic and lysosomal) and the size of Aβ1–42 containing lysosomes were decreased compared with controls. Bar, 30 μm. n = 3. Nuclei were stained by DAPI (blue fluorescence). (B). The percentage of cells with large Aβ1–42 positive lysosomes significantly decreased after 1 d of DAPT pretreatment followed by 3 d exposure to hyperoxia and DAPT, in comparison to controls (*p < 0.05; n = 3).
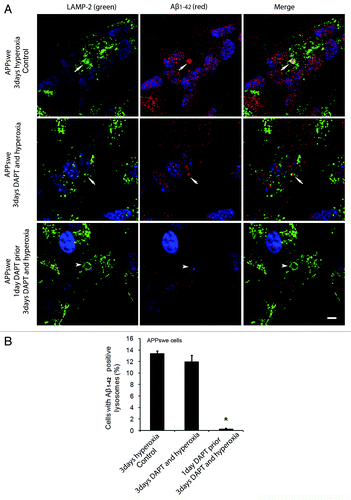
Figure 11. Effects of γ-secretase inhibition by DAPT on intralysosomal accumulation of Aβ oligomers. (A) Double immunostaining of LAMP-2 (green) and Aβ oligomers (red) in APPswe cells after 3 d exposure to hyperoxia (controls), 3 d exposure to hyperoxia and DAPT (500 nM) or 1 d DAPT pretreatment followed by 3 d exposure to hyperoxia and DAPT. Arrows show colocalization of Aβ oligomers with lysosomes while arrowheads indicate enlarged lysosomes without accumulation of Aβ oligomers. After DAPT treatment, the general amount of Aβ oligomers (cytosolic and intralysosomal) and the size of Aβ oligomer containing lysosomes were decreased compared with controls. Bar, 30 μm. n = 3. Nuclei were stained by DAPI (blue fluorescence). (B). The percentage of cells with large lysosomes containing Aβ oligomers significantly decreased after 1 d DAPT pretreatment followed by 3 d exposure to hyperoxia and DAPT, in comparison to controls (*p < 0.05; n = 3).
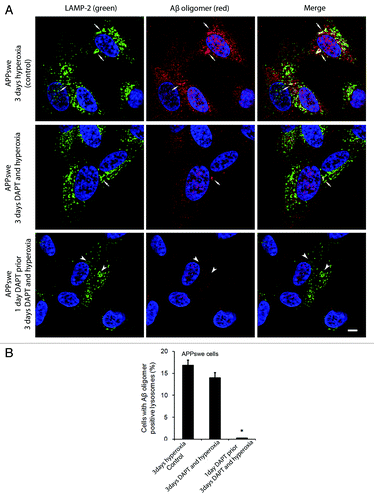
Figure 12. Hyperoxia enhanced endocytosis without inducing Aβ localization to endosomes. (A) Single immunostaining of Rab5 (green fluorescence) in vector, APPwt and APPswe cells under normoxia or hyperoxia. Under normoxia, Rab5 showed a preferentially diffuse (cytosolic) distribution pattern, while after exposure to hyperoxia it becomes more granular (membrane bound), suggesting an upregulation of early endosomes after oxidative stress. Bar, 60 μm. n = 3. Nuclei were stained by DAPI (blue fluorescence). (B and C) Double immunostaining showed no colocalization between Aβ1–42 (B, red fluorescence) or Aβ oligomers (C, red fluorescence) and Rab5 (B and C, green fluorescence) in APPswe cells after exposure to hyperoxia (arrow and magnified image), suggesting that most of intralysosomal Aβ was not delivered by endocytosis. Bar, 40 μm. n = 3. Nuclei were stained by DAPI (blue fluorescence).
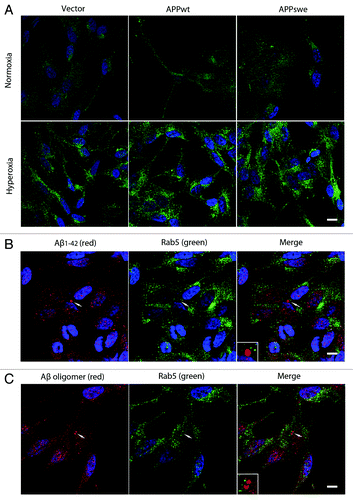