Figures & data
Figure 1. Some key features of ciliary kinesins. (A) An electron micrograph of a cross section through a cilium in zebrafish (left, from ref. Citation64) and a schematic representation of ciliary microtubules (right). Inside the ciliary shaft, microtubules form nine doublets that point their plus ends toward the tip of the cilium. These doublets frequently continue as single microtubules in the distal region, sometimes referred to as the “distal segment” of the cilium (d. s.). The portion of the cilium occupied by single microtubules is highly variable in different cells. (B and C) Kinesins are microtubule-dependent motors that transport cargo toward plus ends of microtubules. In all species examined so far, the heterotrimeric kinesin-2 (also known as kinesin-II) plays a major role in ciliary transport. It consists of two distinct motor subunits and an accessory subunit (KAP3). In C. elegans, two genes encode kinesin-II motor subunits (B). In vertebrates, on the other hand, these motor subunits are encoded by at least three loci (C). Their protein products are thought to assemble into complexes containing the Kif3a subunit, and either Kif3b or Kif3c. Thus at least two distinct Kinesin-II complexes appear to function in vertebrates. In addition to the heterotrimeric kinesin-2, the homodimeric kinesin-2, in vertebrates encoded by the kif-17 gene, contributes to ciliary transport. In C. elegans, this kinesin is frequently redundant with Kinesin-II and plays a major role in cilia formation. In at least one group of cells, the homodimeric kinesin-2, but not the heterotrimeric one, is necessary for the formation of the distal segment. In vertebrates, the homodimeric kinesin-2 appears to play a less significant role. It may transport unique cargos and/or kinesin-II cargos. There is no evidence that the formation of single microtubules in the distal portion of vertebrate cilia requires kinesins that differ from these that function in the formation of microtubule doubles. Kinesins of other families also contribute to ciliary transport (not shown). Verterbrate and C. elegans nomenclature is provided at the bottom of the figure.
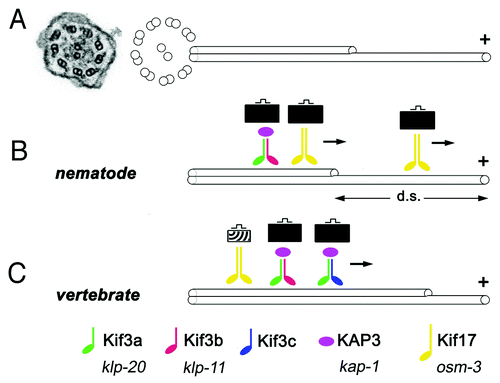
Figure 2. A subset of cilia differentiate in kif3bjj203 mutant embryos. In (A, A’, B and B’), shown are wild-type (A and B) and mutant (A’ and B’) embryos stained with anti-acetylated tubulin antibody at 3 (B and B’) and 7 (A and A’) dpf. (A and A’) Confocal images of a macula in the zebrafish ear. Cilia (arrows) are present in both wild type and mutant. (B and B’) Confocal images of a crista of the zebrafish ear. Cilia (arrows) are absent in the mutant. (C, C’ and D’) Electron micrographs of sections through wild-type (C and D) and mutant (C’ and D’) photoreceptor cells at 3.5 (C and C’) and 5 (D and D’) dpf. Photoreceptor outer segments [OS, asterisks in (C?E)] are initially absent in the mutant. (E) A schematic drawing of the vertebrate photoreceptor cell (after Kennedy and Malicki, 2009). The outer segment membrane is in red. Microtubules that support its structure are in blue. In this work, term “cilium” is used to mean the structure that includes the connecting cilium and the outer segment. The outer segment (OS) forms in the distal part of photoreceptor cilia, which differentiates membrane folds. The connecting cilium (CC), on the other hand, is the proximal region of photoreceptor cilia, and displays characteristics of the ciliary transition zone. OLM, outer limiting membrane.
![Figure 2. A subset of cilia differentiate in kif3bjj203 mutant embryos. In (A, A’, B and B’), shown are wild-type (A and B) and mutant (A’ and B’) embryos stained with anti-acetylated tubulin antibody at 3 (B and B’) and 7 (A and A’) dpf. (A and A’) Confocal images of a macula in the zebrafish ear. Cilia (arrows) are present in both wild type and mutant. (B and B’) Confocal images of a crista of the zebrafish ear. Cilia (arrows) are absent in the mutant. (C, C’ and D’) Electron micrographs of sections through wild-type (C and D) and mutant (C’ and D’) photoreceptor cells at 3.5 (C and C’) and 5 (D and D’) dpf. Photoreceptor outer segments [OS, asterisks in (C?E)] are initially absent in the mutant. (E) A schematic drawing of the vertebrate photoreceptor cell (after Kennedy and Malicki, 2009). The outer segment membrane is in red. Microtubules that support its structure are in blue. In this work, term “cilium” is used to mean the structure that includes the connecting cilium and the outer segment. The outer segment (OS) forms in the distal part of photoreceptor cilia, which differentiates membrane folds. The connecting cilium (CC), on the other hand, is the proximal region of photoreceptor cilia, and displays characteristics of the ciliary transition zone. OLM, outer limiting membrane.](/cms/asset/a5011ce7-96c6-4e2a-840e-23e80de7d87d/kbia_a_10921101_f0002.gif)
Figure 3. Current understanding of functional relationships between kinesin-2 family motors in vertebrate ciliogenesis. (A) In rod photoreceptors, kif3b is necessary for cilia differentiation and cell survival. In cone photoreceptors, on the other hand, kif3b is necessary for cilia formation at 3 dpf. Somewhat later, at 5 dpf, kif3b and kif3c function largely redundantly in cone cilia formation. kif3c does not, however, entirely compensate for the loss of kif3b function, and vice versa kif3b does not seem to entirely substitute for the loss of kif3c. (B) In the ear, two populations of mechanosensory hair cells display very different requirements for kinesin function: while kif3b is required for ciliogenesis in auditory maculae, kif3b and kif3c function redundantly in the cilia of cristae; either is sufficient to drive ciliogenesis in these cells. (C) In olfactory placodes, both kif3b and kif17 are necessary for normal ciliogenesis. The contribution of kif3b is much more significant, compared with kif17. Based on mouse knockout data, Kif3a is necessary for the differentiation of all cilia, except perhaps in photoreceptor cells, where yet another mechanism may be operational (dashed arrow).
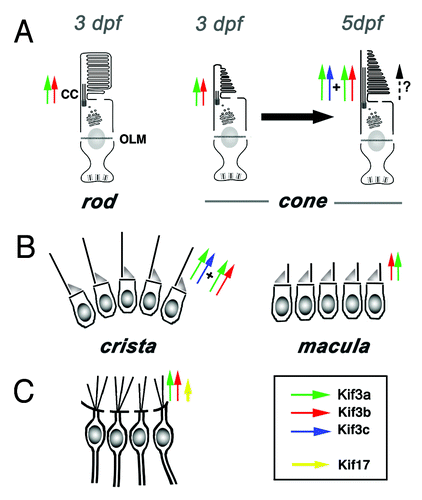