Figures & data
Figure 1. The growth of Oceanobacillus sp. PUMB02 on nutrient agar media supplemented with 2% NaCl (A). The hydrolysis zone on tributyrin plate (B) and olive oil plate (C).
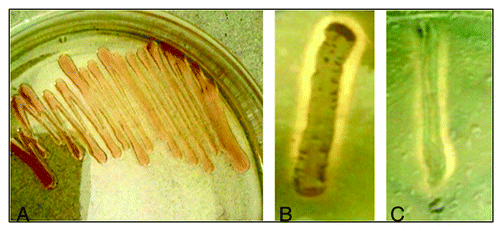
Figure 2. Maximum Parsimony bootstrapping phylogenetic tree of Oceanobacillus sp. PUMB02 and closest NCBI (Mega BLAST) relatives based on the 16S rRNA gene sequences. Phylogeny reconstruction was performed using Maximum Parsimony. Bootstrap values calculated from 1,000 re-samplings are shown at the respective nodes when the calculated values were 50% or greater.
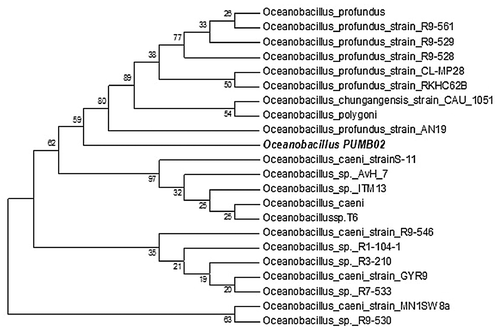
Figure 3. 3-D contour plots of relative enzyme activity with four variables (A: Olive oil, B: Sucrose, C: Potassium chromate, and D: NaCl). (A) Sucrose (g/L) and Olive oil (ml/L), (B) Olive oil (ml/L) and Potassium chromate (mg/L), (C) Olive oil ml/L and NaCl (% v/v), (D) Sucrose (g/L) and Potassium chromate (mg/L), (E) Sucrose (g/L) and NaCl (% v/v), (F) Potassium chromate (mg/L) and NaCl (% v/v).
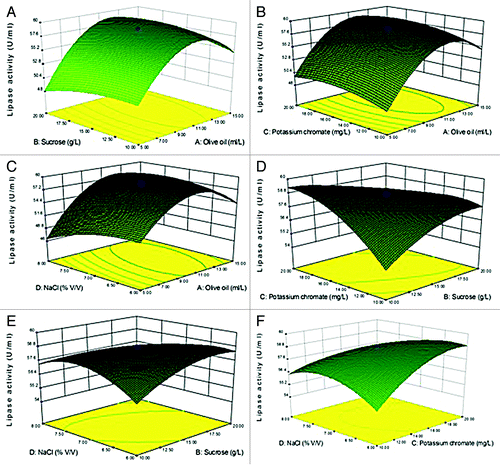
Figure 4. Purification of PUMB02 lipase. The dialyzed solution was purified with a fast-performance liquid chromatography (FPLC) system (BioRad) equipped with an anionic exchange (DEAE Sepharose) which was previously equilibrated with 50mM tris-HCl buffer (pH 7.4). The column was washed with 50 ml of the buffer and then eluted with a linear gradient of 0 to 1.0 M sodium chloride (NaCl) in the same buffer. Linear flow rate was 0.5 ml/min and fractions were collected every 2.0 min. The protease activity and protein concentration (A280) in each fraction were measured. (A) Butyl-sepharose anion exchange chromatography. (B) DEAE sephadex gel.
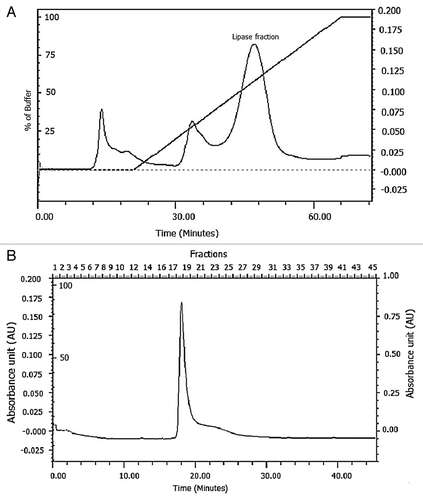
Table 1. Purification summary of lipase from Oceanobacillus PUMB02
Figure 5. MALDI-TOF analysis of the tryptic digest fingerprint of PUMB02 lipase predicting the peptide mass to be 31kDa. Lane a: zymogram of PUMB02 lipase; Lane b: crude protein; Lane c: purified protein after size-exclusion chromatography; Lane d: molecular weight markers.
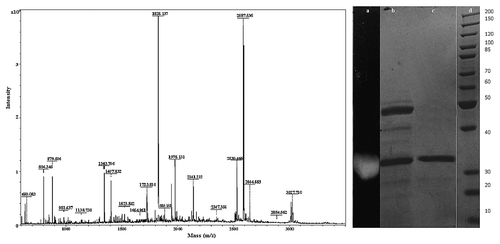
Figure 6. FT-IR analysis showing Broad Peak around 3600–3000 cm−1corresponds to the hydrogen bonded stretching vibration of - -N-H or O-H. The peak around 1700–1600 cm−1 due the overlapping of -C = O stretching and amide -N-H bending frequency and another peak at 1507 cm−1 N-H symmetric bending vibration.CH2- bending frequency observed at 1455 cm−1.The peak at 1404 cm−1corresponds to the amide -C-N stretching band. The strong and broad peak around 1100–900 cm−1 corresponds to the -C-O stretching vibration.
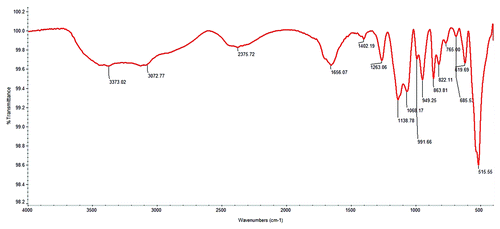
Table 2. Stability characterization of lipase from Oceanobacillus
Figure 7. Inhibition of pre-formed biofilms of E. coli, B. cereus, Pseudomonas sp., Vibrio sp., Serratia, and Listeria sp by lipases. (A) The BIC of Oceanobacillus PUMB02 lipase was found at the concentration 150µl for E. coli, B. cereus, Pseudomonas sp., Vibrio sp., S. typhi, and Listeria sp. (B) The BIC of Lpc53E1 lipase was 50 µl for E. coli followed by 100 µl B. cereus and S. typhi and 150 µl for Pseudomonas sp., Vibrio sp., and Listeria sp.
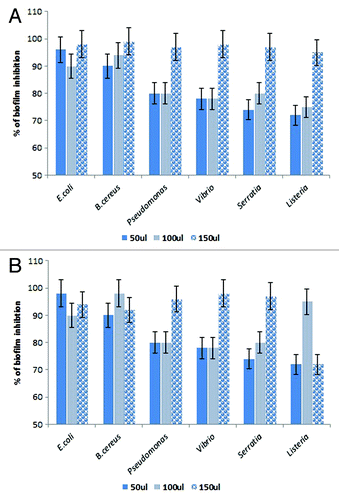
Figure 8. Phase contrast microscopic images showing antibiofilm effect of lipases against biofilm forming pathogens at their BIC.
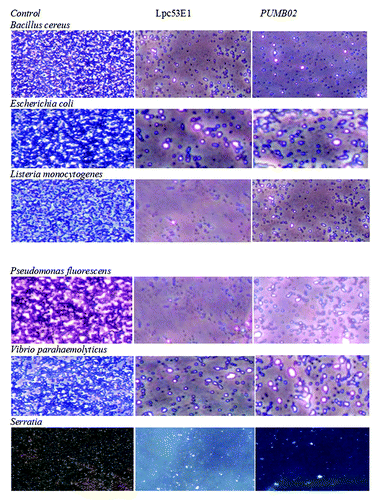