Figures & data
Figure 2. SEM micrographs of biodegradable drug-loaded porous structures derived from freeze-dried inverted emulsions: (A) cross section of a film, (B) composite mesh/matrix structure and (C) cross section of core/shell fiber. High magnification of the porous structure is shown in the circle.
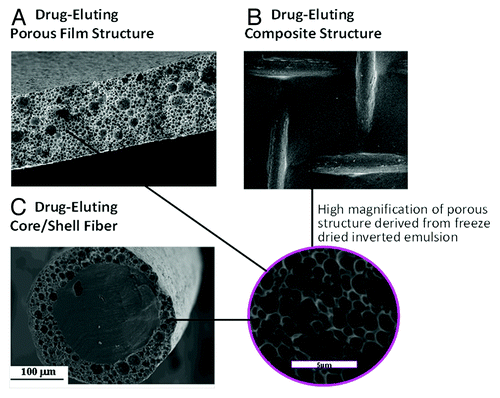
Table 1. Structural characteristics of the ceftazidime-loaded porous matrixCitation70
Figure 3. (A) Controlled release of the antibiotic drug ceftazidime from composite structures based on various formulations. ○, the reference formulation (formulation 1): 5% w/w ceftazidime and 15% w/v polymer (75/25 PDLGA, MW = 100 KDa), O:A = 6:1; △, formulation 2: increased O:A phase ratio (12:1); formulation 3: increased polymer MW (240KDa); ◇, formulation 4: increased polymer content in the organic phase (20%). (B–E) SEM fractographs showing the effect of a change in the emulsion’s formulation parameters on the microstructure of the binding matrix for formulations 1–4, respectively.Citation10
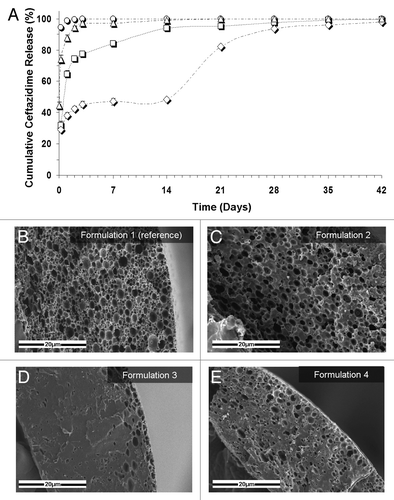
Figure 4. (A–C) SEM fractographs demonstrating the microstructure of wound dressings based on formulations BSA1, BSA2 and SPAN, respectively. (D) The controlled release of the antibiotic drug ceftazidime from the three studied wound dressings and (E) water vapor transmission rates, corresponding to each sample, together with these obtained from a dense (non-porous) PDLGA (50/50, MW 100 KDa) film () and from an uncovered surface.Citation70
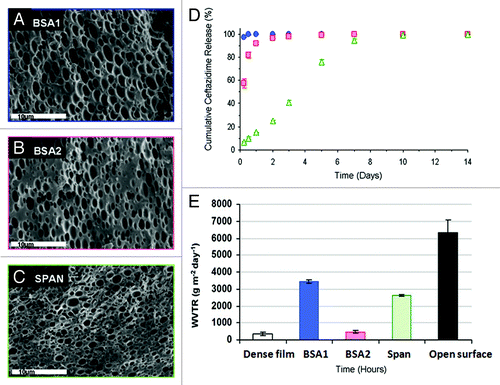
Table 2. Mechanical properties of various wound dressingsCitation70
Figure 5. (A) Tensile stress-strain curves for wound dressings immersed in water for 0 (–0–), 1 (–1–), 2 (–2–), and 3 (–3–) weeks. (B) Young’s modulus, (C) tensile strength and (D) maximal tensile strain as a function of immersion time. Comparison was made using ANOVA and significant differences are indicated (*).Citation70
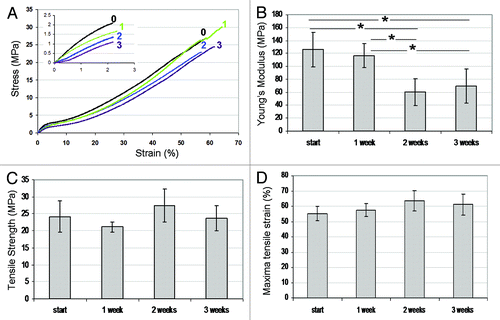
Table 3. Minimum inhibitory concentrations of antibioticsCitation69
Figure 6. Histograms showing the effect of drug release on corrected zone of inhibition (CZOI) around (1% w/v) BSA loaded wound dressings (n = 3) containing 5% (w/w), 10% (w/w) and 15% (w/w) drug, as a function of pre-incubation time in PBS. (A–C) gentamicin-loaded wound dressings, (D–F) ceftazidime-loaded dressings. The bacterial strain (P. aeruginosa, S. albus and S. aureus) is indicated.Citation69
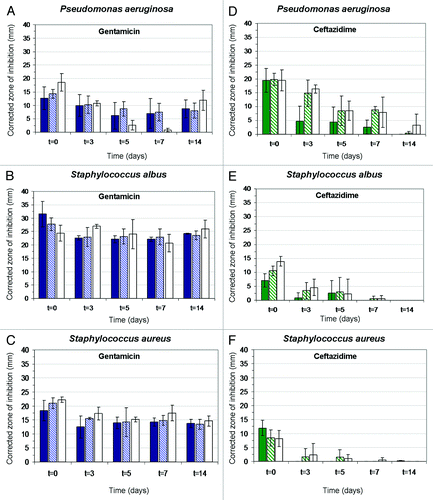
Figure 7. Histograms demonstrating changes in the viability of dermal fibroblast cultures (Alamar Blue assay) in the presence of wound dressing discs (D = 10 mm): (A) BSA-stabilized wound dressings (n = 3) containing 5% or 15% (w/w) gentamicin. (B) BSA and Span stabilized wound dressings containing 5% or 15% ceftazidime. Dressing materials devoid of antibiotics and pristine cell cultures served as control.Citation69
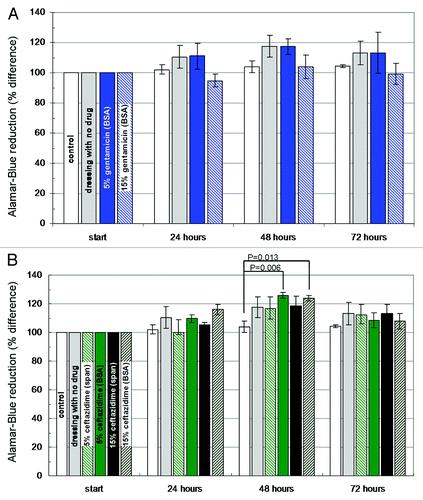
Figure 8. (A) cumulative release of gentamicin from wound dressings derived from emulsions with 10% drug contents, that were used in the animal study: (blue square) formulation based on 6:1 O:A phase ratio, stabilized with 1% (w/v) BSA (“fast release”). (Green circle) Formulation based on 12:1 O:A phase ratio, stabilized with 1% (w/v) Span 80 (“slow release”). (B) Representative photographs of wounds, ten and 14 d after treatment with the four types of wound dressings: Melolin® (group 1), “control” (group 2), “fast release” (group 3) and “slow release” (group 4).Citation111
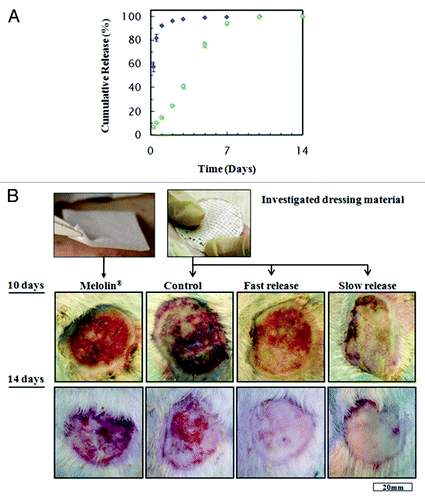
Figure 9. (A) Percentage of open wound measured at 10 and 14 d, with respect to the inflicted wound area (mean ± SEM), (B) Wound contraction as percentage of total wound area measured at 10 and 14 d, with respect to the inflicted wound area (mean ± SEM).Citation111
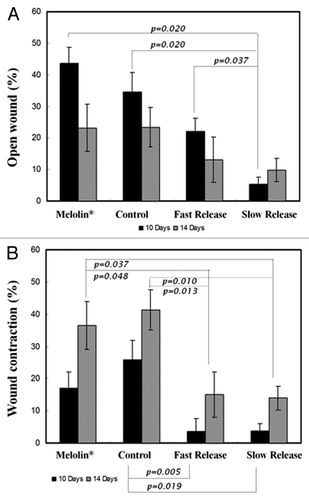
Table 4. The structural characteristics of the shell structures loaded with antiproliferative agents and their encapsulation efficiency valuesCitation133
Figure 10. The effect of the copolymer composition on the cumulative drug release profile from core/shell fiber structures (Green triangle, 50/50 PDLGA; blue circle, 75/25 PDLGA): (A) Paclitaxel release, (B) FTS release. Plots of dMt/dt vs. sqrt (1/t) for the first 5 weeks of release (in the small frames) indicate diffusion controlled region.Citation71,Citation72
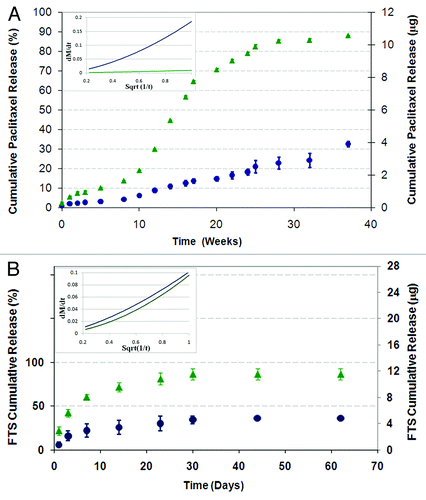
Figure 11. Degradation profile: (A) weight loss profile, (B) and water uptake, (C) green triangle, 50/50 PDLGA; blue circle, 75/25 PDLGA porous structures.Citation133
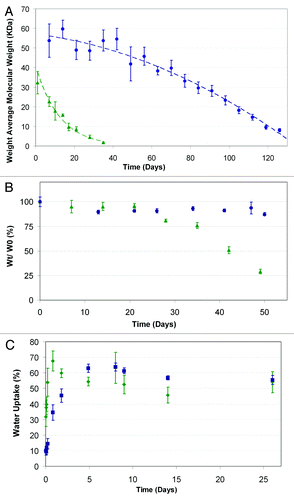
Figure 12. SEM fractographs of shell structures showing their microstructural changes with time: (A) 50/50 PDLGA at days 0, 7, 14, 28, (B) 75/25 PDLGA at days 0, 7, 14, 28, 56 and 126.Citation133
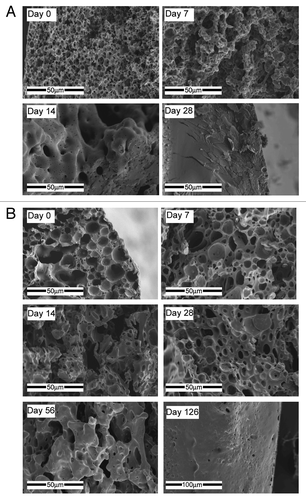
Table 5. Physical properties of the hydrophobic drugs Paclitaxel and FTSCitation135
Figure 13. The 3D structures of sirolimus and paclitaxel. The various atoms are presented by colors as follows: H, white; C, gray; O, red; N, blue; S, yellow.135
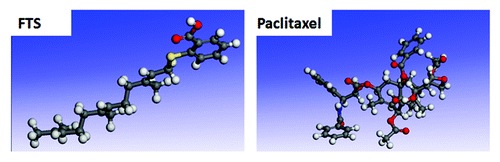
Figure 14. Both control paclitaxel and post-fabrication paclitaxel induce a significant decrease in the cell count. Cells (EJ, A549 or U87) were plated at a density of 10 × 103 cells/well in a 24-well plate in tetraplicate (n = 2). One day after plating, 0.1% DMSO (control), 50 μM control paclitaxel (black columns), or 50 μM post-fabrication paclitaxel (white columns) were added for 24 h. The cells were then counted using a hemocytometer (*p < 0.01 compared with the control well). Paclitaxel's effect on the above-mentioned cell lines is presented in terms of mean cell viability ± standard deviation.Citation133
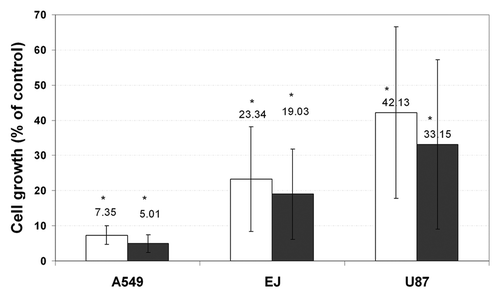
Figure 15. FTS loaded core/shell fiber structures inhibit growth or induce cell death of glioblastoma cells by a gradient effect and dose-dependent manner. U87 cells were plated at a density of 8x103 cells/well in a 24-well plate in tetraplicate (n = 2). One day after plating, control fibers (not loaded with FTS), slow or fast FTS release fibers were added to each well (2 fibers, each with a length of 1 cm, as described in the materials and methods). (A) Images taken after 5 d of incubation with FTS fibers, in locations which are near and distant to the fiber (magnification × 100). (B) A single well edge to edge panoramic view shows a gradiential increase in the cell concentration with the increase in the distance from a slow release fiber (magnification × 100, cells were counted using an image analysis software). (C) Images were taken after 7 d of incubation with FTS fibers; the control fiber well presents high cell viability while the well containing the fast FTS fiber exhibited cell death (magnification × 100). Note that the dark shape is the actual fiber.Citation133
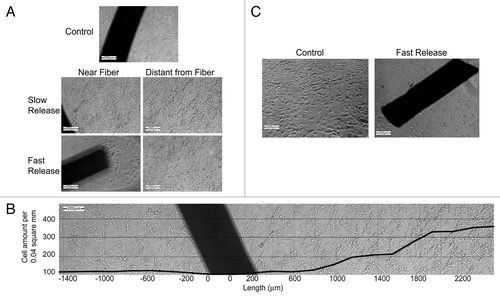
Figure 16. In vitro release of HRP from the porous scaffolds demonstrating the effect of a change in the emulsion's formulation parameters compared with the reference formulation: (A) effect of HRP content: green diamond, 2% w/w; blue square, 1% w/w; red triangle, 0.5% w/w. (B) Effect of polymer content: yellow circle, 15% w/v; blue square, 17.5 w/v; empty green triangle, 25% w/v. (C) effect of O:A phase ratio: white square, 2:1, empty blue diamond, 6:1, x - 8:1.Citation67
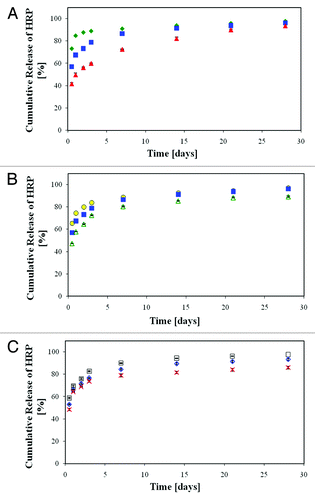
Figure 17. In vitro release of HRP from the porous scaffolds demonstrating the effect of a change in the host polymer compared with the reference sample: (A) effect of the copolymer composition: blue square, 50/50 PDLGA, red triangle, 75/25 PDLGA. (B) Effect of the initial molecular weight of the host polymer: green diamond, 50 KDa; blue square, 83 KDa; black circle, 185 KDa.Citation67
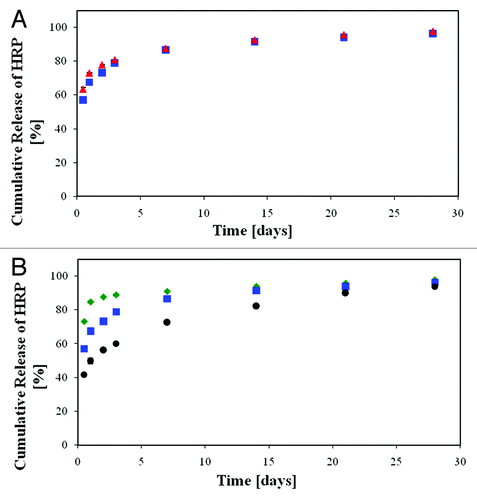
Table 6. Structural features of HRP-eluting films with a change in 2 or 3 parameters (compared with the reference sample)Citation67
Figure 18. In vitro release of HRP from the porous scaffolds demonstrating the effect of combined changes in the emulsion's formulation parameters compared with the reference formulation (blue square, 17.5% w/v polymer, i.v. = 83 KDa, 1% w/w HRP, O:A = 4:1). (A) Combined effect of a change in 2 parameters, 0.5% w/w HRP and: green diamond, 25% w/v polymer; black circle, 185 KDa; red triangle, O:A = 8:1. (B) White circle, combined effect of a change in three parameters (0.5% w/w HRP, 25% w/v polymer, O:A = 8:1). (C) X, composite film composed of a porous inner 50/50 PDLGA film (25% w/v polymer, MW = 83 KDa, O:A = 8:1 and 0.5% w/w HRP) and two external PDLLA layers (17.5% w/v polymer, MW = 80 KDa, O:A = 2:1, no HRP).Citation67
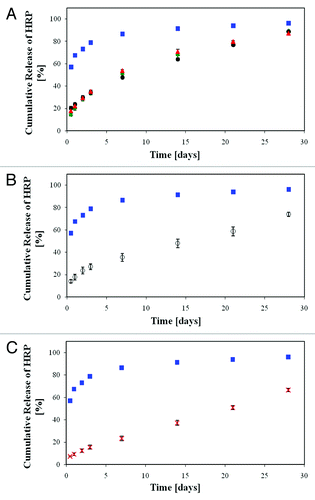
Figure 19. Schematic representation of a qualitative model describing the drug release mechanisms from the porous drug eluting structures derived from freeze-dried emulsions.
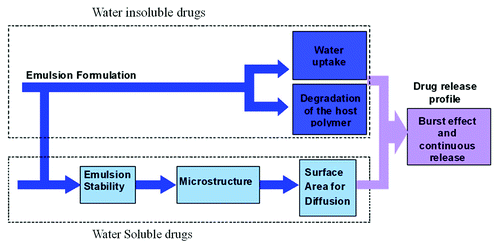