Figures & data
Figure 1. Structures of clinically relevant antifolates. Structures are shown for aminopterin (AMT), methotrexate (MTX), and pralatrexate (PDX), all dihydrofolate reductase (DHFR) inhibitors, and thymidylate synthase inhibitors raltitrexed (RTX) and pemetrexed (PMX).
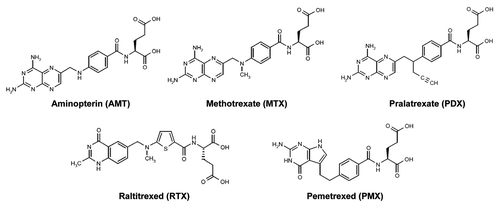
Figure 2. Folate transporters, folate metabolic pathways and intracellular enzyme targets of antifolates. Folate and antifolate transport across biological membranes is mediated by the reduced folate carrier (RFC), the proton-coupled folate transporter (PCFT) and folate receptors (FR). While RFC and PCFT are facilitative transporters, FRs mediate transport by a non-classical endocytosis involving formation of endosomes that migrate to the cytoplasm. Exit of the folate ligand from the endosome involves diffusion or a mediated process. PCFT has been proposed to facilitate endosomal exit,Citation38 however this does not appear to be obligatory.Citation16 Intracellular folates including tetrahydrofolate (THF), dihydrofolate (DHF), 10-formyl tetrahydrofolate (10-CHO-THF), 5, 10-methylene tetrahydrofolate (5,10-CH2-THF), 5,10-methenyl tetrahydrofolate (5,10-CH+-THF), and 5-methyl tetrahydrofolate (5-CH3-THF) participate in folate interconverting and biosynthetic reactions. Reactions 1–4 are in both the cytoplasmic and mitochodrial compartments. Reactions 1 and 9 are also present in the nucleus. Reaction 1 designates serine hydroxymethyltransferase. In the mitochondria, reactions 2 and 3 are catalyzed by bifunctional 5,10-CH2-THF dehydrogenase (MTHFD) 2 or MTHFD2L and 4 is catalyzed by monofunctional MTHFD1L. In the cytoplasm, reactions 4, 3 and 2 are catalyzed by the 10-CHO-THF synthetase, 5,10-CH+-THF cyclohydrolase and MTFD activities, respectively, of the trifunctional C1-THF synthase (MTHFD1). Other steps shown are catalyzed by β-glycinamide ribonucleotide formyltransferase (GARFTase; reaction 5), 5-amino-4-imidazolecarboxamide ribonucleotide formyltransferase (AICARFTase; reaction 6), thymidylate synthase (TS; reaction 7), dihydrofolate reductase (DHFR; reaction 8), 5,10-methyleneTHF reductase (MTHFR; reaction 9), and methionine synthase (MS; reaction 10). Antifolates inhibit folate biosynthetic reactions as shown and include aminopterin (AMT), methotrexate (MTX), pralatrexate (PDX), raltitrexed (RTX), lometrexol (LMX), pemetrexed (PMX), ONX-0801 (ONX), and compounds 3, 16 and 17.
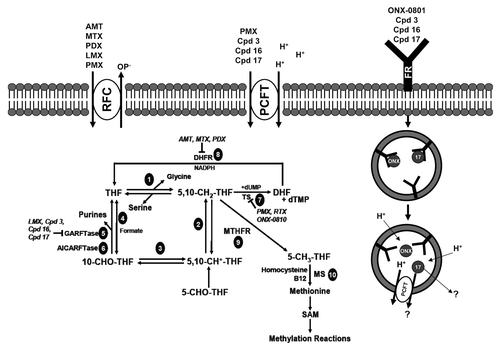
Figure 3. Schematic structure of PCFT topology. Structurally or functionally important amino acids, as determined from published mutagenesis studies, are shown as purple circles. Amino acids mutated in patients with hereditary folate malabsorption (HFM) are shown as red and blue circles. GxxxG putative oligomerization motifs are shown as yellow circles and glycosylated residues Asn58 and Asn68 are shown as green circles.
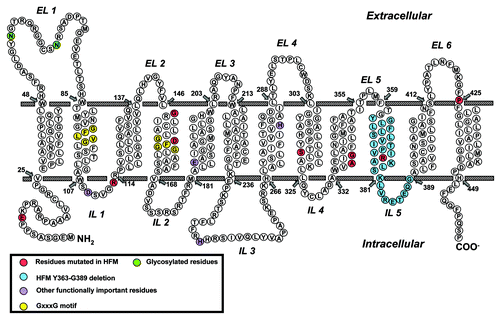
Figure 4. PCFT and RFC transcript expression in human normal tissues. PCFT transcripts were measured by real-time RT-PCR using an Origene cDNA array prepared from 48 pathologist-verified human normal tissues as previously described.Citation12 Transcript levels were normalized to GAPDH transcripts.
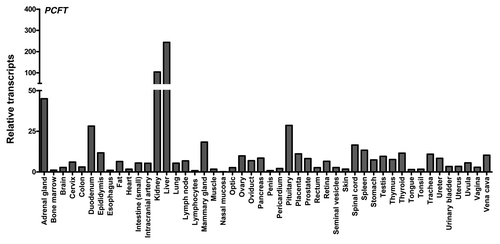
Figure 5. Proposed reaction scheme for hPCFT-mediated cellular uptake involving cooperative interactions between hPCFT monomers. Based on the “alternate access model” for secondary transporters such as Lac Y,Citation91 adapted from that of Unal et al. for monomeric PCFT,Citation82 an analogous reaction scheme is depicted for hPCFT-mediated transport which incorporates the functional impact of hPCFT oligomerization. The model starts from the outward-facing unloaded dimer, followed by the ordered binding of the co-transported protons (step 1) and (anti)folate substrates (step 2), which triggers a conformational change resulting in simultaneous transition of the two hPCFT monomers to an inward-facing state (step 3). This is followed by an ordered release of substrates (step 4) and then protons (step 5) into the cytoplasm. The unloaded homo-oligomeric unit then returns to the outward-facing state (step 6) to complete the transport cycle. In this model, the two hPCFT monomers are suggested to function cooperatively in facilitating substrate and proton binding, conformational changes, and substrate and proton release. From Hou et al.Citation87
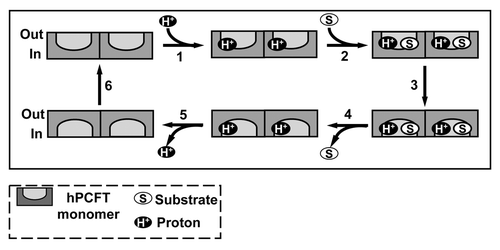
Figure 6. De novo purine nucleotide biosynthesis pathway. The de novo purine nucleotide biosynthetic pathway from phosphoribosyl pyrophosphate (PRPP) to IMP is shown. The numbered reactions are catalyzed by the following monofunctional enzymes: 1, glutamine phosphoribosylpyrophosphate amidotransferase (GPAT); 4, formylglycinamide ribonucleotide synthase (FGAM synthetase); and 8, adenylosuccinate lyase (ASL). Reactions 2, 3 and 5 are catalyzed by the trifunctional glycinamide ribonucleotide (GAR) formyltransferase (GARFTase) which contains GAR synthase (GARS; reaction 2), GAR formyltransferase (GARFTase; reaction 3) and 5-amino-4-imidazole ribonucleotide synthase (AIRS; reaction 5) activities. Reactions 6 and 7 are catalyzed by the bifunctional phosphoribosylaminoimidazole carboxylase/ phosphoribosylaminoimidazole succinocarboxamide synthetase (PAICS) enzyme, which contains carboxyaminoimidazole ribonucleotide synthase (CAIRS; reaction 6) and 5-aminoimidazole-4-(N-succinylocarboxamide) ribonucleotide synthase (SAICARS; reaction 7) activities. Reactions 9 and 10 are catalyzed by a bifunctional enzyme, 5-amino-4-imidazolecarboxamide ribonucleotide (AICAR) formyltransferase (AICARFTase)/IMP cyclohydrolase (ATIC) that sequentially catalyzes the last two steps in the pathway for de novo synthesis of IMP. Folate-dependent reactions (reactions 3 and 9) in which 10-CHO-THF serves as the one-carbon donor are catalyzed by GARFTase and AICARFTase. 5-Aminoimidazole-4-carboxamide (AICA) and AICAR can be metabolized to AICAR monophosphate (ZMP) by adenine phosphoribosyl transferase (APRT) and adenosine kinase (AK), respectively, thus circumventing the reaction catalyzed by GARFTase.
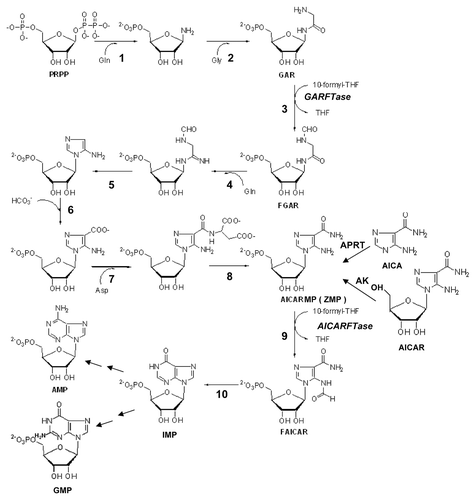
Figure 7. Structures of GARFTase inhibitors. Structures are shown for Lometrexol (LMX) and LY309887 and AG2034.
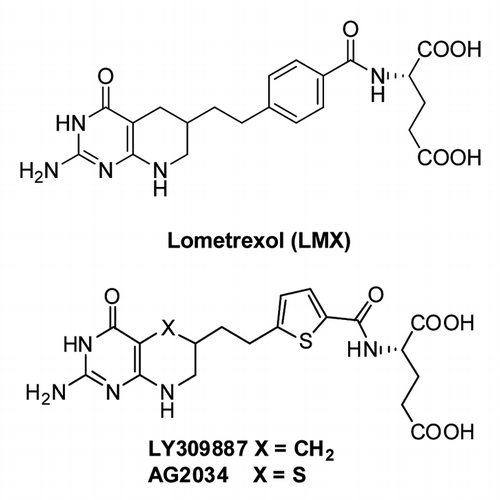
Figure 8. 6-Substituted pyrrolo- and thieno[2,3-d]pyrimidine antifolates with hPCFT and FR specificity over hRFC. Structures are shown for 6-substituted pyrrolo[2,3-d]pyrimidine benzoyl antifolates with carbon bridge length variations of 1- to 6-carbons (compounds 1–6, respectively), 6-substituted thieno[2,3-d]pyrimidine benzoyl antifolates with bridge length variations from 2–8 carbons (compounds 7–13, respectively), 6-substituted pyrrolo[2,3-d]pyrimidine antifolates with a thienoyl replacement for the benzoyl moiety and bridge length variations from 1–6 carbons (compounds 14–19, respectively), and 6-substituted pyrrolo[2,3-d]pyrimidine thienoyl regioisomers of compound 17 (compounds 20–24).
![Figure 8. 6-Substituted pyrrolo- and thieno[2,3-d]pyrimidine antifolates with hPCFT and FR specificity over hRFC. Structures are shown for 6-substituted pyrrolo[2,3-d]pyrimidine benzoyl antifolates with carbon bridge length variations of 1- to 6-carbons (compounds 1–6, respectively), 6-substituted thieno[2,3-d]pyrimidine benzoyl antifolates with bridge length variations from 2–8 carbons (compounds 7–13, respectively), 6-substituted pyrrolo[2,3-d]pyrimidine antifolates with a thienoyl replacement for the benzoyl moiety and bridge length variations from 1–6 carbons (compounds 14–19, respectively), and 6-substituted pyrrolo[2,3-d]pyrimidine thienoyl regioisomers of compound 17 (compounds 20–24).](/cms/asset/e36ad4fd-29ac-44ca-8418-d10223e01df7/kcbt_a_10922020_f0008.gif)
Figure 9. Characterization of compound 17 and PMX growth inhibition in R1–11 sublines differing in hPCFT and hRFC expression. Cell proliferation inhibition was measured for R1–11-PCFT4 (expresses hPCFT but not hRFC) and -RFC6 (expresses hRFC but not hPCFT) cells treated with PMX (A) or compound 17 (B) over a range of concentrations in complete folate-free RPMI1640 in the presence of 5-CHO-THF at 25 nM for 96 h. Cell densities were measured with CellTiter BlueTM fluorescence dye and a fluorescence plate reader. Results were normalized to cell densities in the absence of drug. From Kugel Desmoulin et al.Citation12
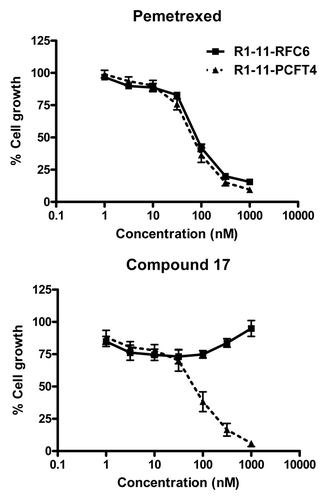