Figures & data
Figure 1. Strand-exchange by RECQ1 on synthetic forked duplexes lacking the leading strand. (A) Scheme of the assay. Strand exchange is a combination of both strand annealing and unwinding activities. Strand annealing of the homologous oligonucleotides by RECQ1 forming the stalled replication fork, which was eventually unwound after the addition of ATP. The lengths of individual arms are indicated in nucleotides (nt) or base pairs (bp). The homologous leading and lagging arms have a 5 nt heterology at the fork junction to prevent spontaneous strand exchange. The 3′ end of the lagging oligonucleotide is indicated by an arrow, and the position of the 5′-PCitation32 label is marked by an asterisk. (B) As indicated above, 1 nM PCitation32-labeled 30/60 mer duplex (RS1/2) was incubated with 1 nM 60 mer complementary oligonucleotide (RS3) in the presence of 20 nM RECQ1 to form the forked DNA structure. After 10 min, ATP was added to a final concentration of 5 mM. Ten μl aliquots were taken out at indicated time points and analyzed by non-denaturing PAGE. “C” represents the control substrate P32-labeled RS1/2; “M” represents the marker for strand exchange product, the 60mer bubble duplex; and “SS” represents the ssDNA unwound. (C) Similar strand exchange reactions were performed in the absence of RECQ1. (D) Quantification of the kinetics performed in the presence and absence of RECQ1. Relative concentration of the 60 mer duplex product was plotted vs. reaction time. The data points represent the average values from three independent experiments.
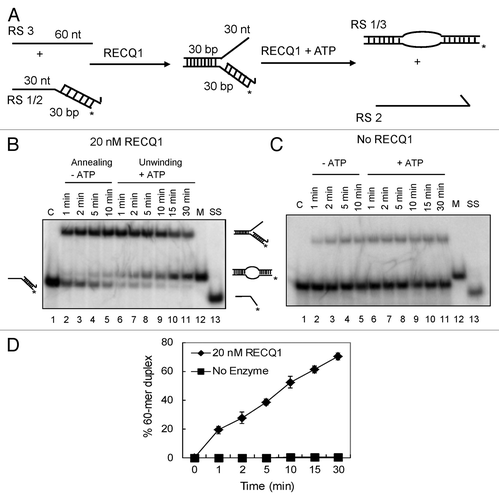
Figure 2. Strand-exchange by RECQ1 on synthetic stalled replication fork duplexes with a leading-strand gap. (A) Scheme of the assay. The oligonucleotides used are the same as in , except for an additional 20 mer representing the leading strand (RS4). (B) As indicated above, 1 nM P32-labeled 20/60 mer duplex (RS3/4) was incubated with 1 nM 30/60 mer duplex (RS1/2) in the presence of 20 nM RECQ1 to form the forked DNA structure. After 10 min, ATP was added to a final concentration of 5 mM. Ten μl aliquots were taken out at indicated time points and analyzed by non-denaturing PAGE. “C” represents the control substrate P32-labeled RS3/4; “M” represents the marker for strand exchange product and “SS” represents the ssDNA unwound. (C) Quantification of the kinetics performed in the presence of 20 nM RECQ1. Relative concentration of the subsequent leading/lagging strand duplex (RS2/4) was plotted vs. reaction time. The data points represent the average values from three independent experiments.
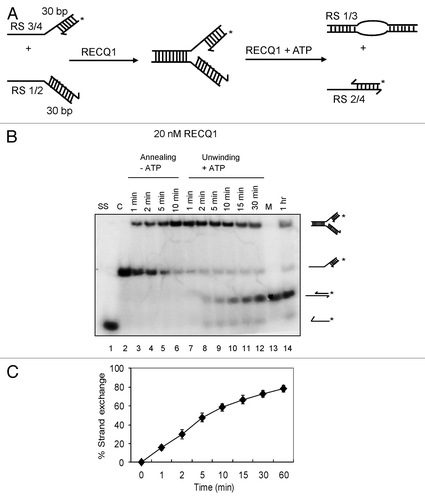
Figure 3. Effect of RPA on the strand exchange activity of human RecQ helicases. (A) Effect of RPA on RECQ1-mediated strand exchange on forked DNA duplex lacking the leading strand (B) and forked DNA duplex with 10 nt leading-strand gap. Effect of RPA on (C) 5 nM WRN, (D) 20 nM RECQ5β and (E) 50 nM RECQ4-mediated strand exchange on forked duplexes lacking the leading strand. Kinetic experiments were performed with 5 nM WRN, 20 nM RECQ5β and 50 nM RECQ4 in the presence or absence of 20 nM RPA. “DS” represents the control duplex substrate PCitation32 labeled RS1/2; “C” represents the control stalled replication fork-like structure; “M” represents the marker for strand exchange product; “SS” represents the ssDNA unwound.
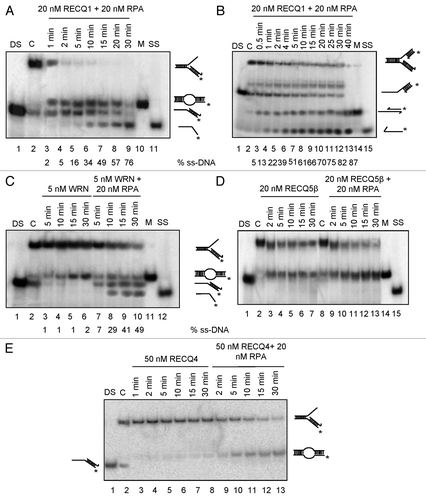
Figure 4. Both RECQ1 and WRN, but not RECQ4 or RECQ5β, can unwind the leading strand of the replication fork duplexes. Replication fork duplex lacking the lagging strand was generated by annealing the oligo nucleotides RS1 and RS5/6. Unwinding kinetics of the replication fork duplexes lacking the lagging strand using 20 nM RECQ1 in the absence (A) or in the presence of 20 nM RPA (B). Unwinding kinetics of the replication fork duplexes lacking the lagging strand using 5 nM WRN (C), 20 nM RECQ5β (D) in the presence and absence of 20 nM RPA. “DS” represents the control duplex substrate PCitation32 labeled RS5/6; “C” represents the control stalled replication fork-like structure; Δ represents the heat denatured substrate.
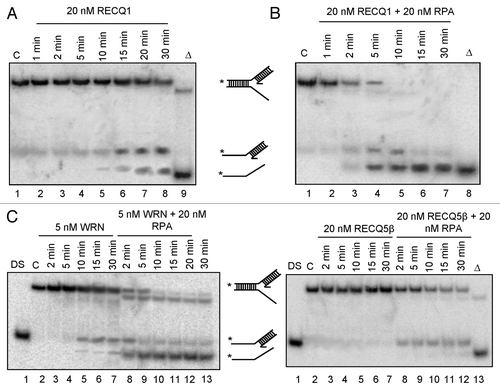
Figure 5. Generation and characterization of stable knockdown cells of RECQ1. (A) Stable depletion of RECQ1 was performed in HeLa cells by using the two independent and dose-dependent lentiviral shRNA, shRECQ1–1 and shRECQ1–2. (B) Growth assays were performed to assess the cell proliferation and cell growth for RECQ1 stable knockdown cells over a period for 5 d. MTT cell proliferation experiments were performed to evaluate the cell proliferation in the increasing concentrations of hydroxyurea (C), camptothecin (D) and 8-methoxypsoralen (E).
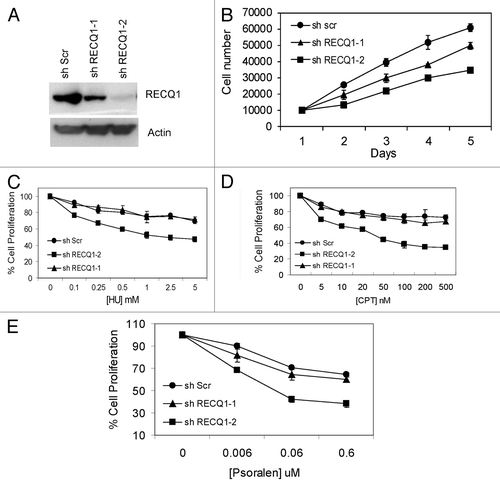
Figure 6. Characterization of stable knockdown cells of RECQ1. (A) FACS analysis or cell cycle distribution of shScrambled and shRECQ1–2-depleted HeLa cells. (B) Stable depletion of RECQ1 accumulates spontaneous replication-associated double-strand breaks (DSBs). Immunostaining of RECQ1-knockdown cells for accumulation of 53BP1 at BrdU sites. (C) Stable depletion of RECQ1 leads to hyper phosphorylation of RPA32. (D) Western analysis showing activation of DNA damage response proteins like p-ATM (1981) and activation of CHK1 (Ser 345) by stable depletion of RECQ1.
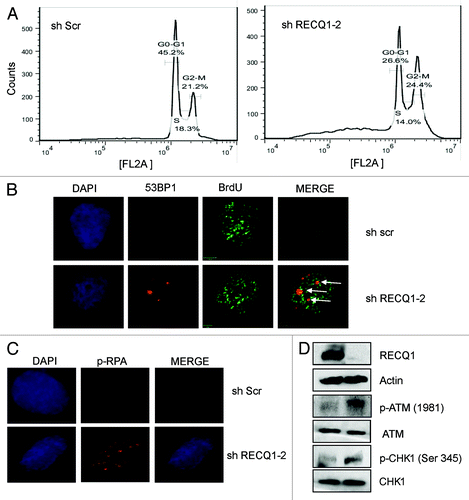
Figure 7. Stable depletion of RECQ1 leads to accumulation of under-condensed chromosomes. (A) Representative images of the metaphase spreads analyzed from RECQ1-depleted cells. The arrows indicate under-condensed chromosomes. (B) Quantification of metaphase spreads from shScrambled and shRECQ1–2-depleted HeLa cells. Results are an average of two independent experiments with a minimum of 50 metaphase spreads analyzed.
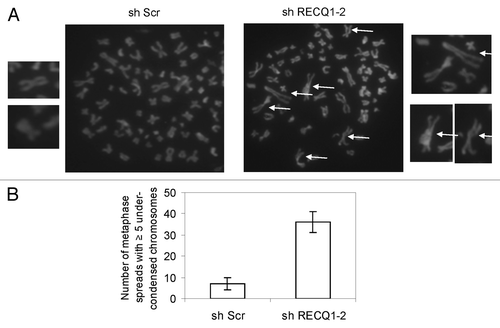
Figure 8. Model for the action of RECQ1 at stalled replication forks and its potential role in the maintenance of genomic stability. (A) The ability of RECQ1 to perform strand exchange at stalled replication fork structures may promote fork reversal, leading to fork stabilization. Red triangle represents a lesion encountered on the leading strand. (B) In vivo implication with a proposed biological role of RECQ1 in the maintenance of genomic stability. RECQ1 deficiency has biological consequences and induces defects in replication fork progression and activates DNA damage signaling.
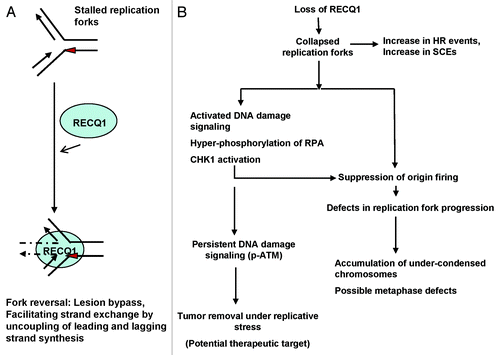