Figures & data
Figure 1. Crystal structure of the S. elongatus KaiC hexamer (PDB ID 3DVL).Citation15
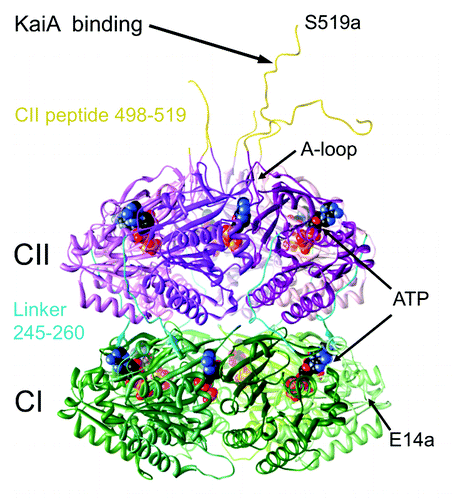
Figure 2. Electrostatic surface potentials (ESPs) of individual rings in S. elongatus KaiC (PDB ID 3DVL) and T. elongatus KaiB (PDB ID 2QKE).Citation19 ESP of (A) the CI ring, (B) the CII ring and (C) KaiB. KaiC rings are viewed from the N terminus, the side and the C terminus (from left to right). KaiB dimer is viewed along the normal to the non-crystallographic dyad (center) and rotated by −90° (left) and +90° (right) around the horizontal axis. The minimum and maximum values of the electrostatic potential are −5 and +5 kT/e, respectively. ESPs were calculated using default parameters of APBS.Citation40
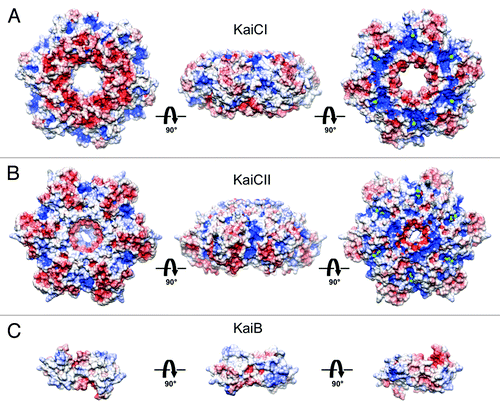
Figure 3. Negative stain EM analysis of S. elongatus KaiC in the presence of 5 nm Ni-NTA-Nanogold. Gallery of C-terminal His6-tagged KaiC particles bound to 5 nm Ni-NTA-gold. Side length of panels equals 240 Å.
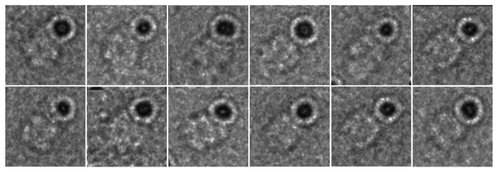
Figure 4. Negative stain EM analysis of the S. elongatus KaiBC complex with and without 1.8 nm Ni-NTA-Nanogold. Representative micrographs of KaiBC particles either (A) unbound or (B) bound to 1.8 nm Ni-NTA-Nanogold. Arrows point to diffuse density not seen in images of unbound complexes. Scale bars equal 50 nm. Representative class sums of the complex between KaiB and KaiC-His6 in the (C) presence and (D) absence of 1.8 nm Ni-NTA-Nanogold. The numbers of particles included in each class is shown in the bottom right corner. (E) Difference mapping between averages depicted in panels (C and D), consistent with the presence of gold density adjacent to KaiB dimers. (F) The difference shown in E filtered at 3σ threshold. (C–F) Side length of panels, 240 Å.
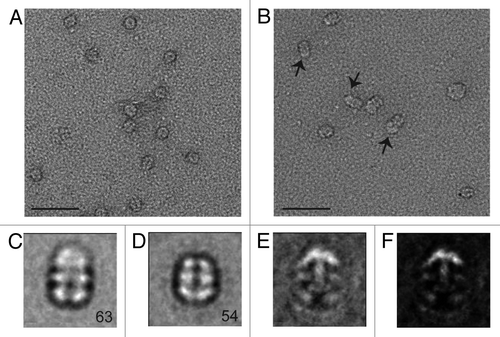
Figure 5. Analysis of S. elongatus KaiBC complex particles with and without 1.8 nm Ni-NTA-Nanogold. (A) Twenty class averages obtained by non-reference based alignment and classification of 875 unlabeled KaiBC particle images in negative stain. The average used for difference mapping (see ) is marked with an “*” in the upper right corner. (B) Twenty class averages obtained by non-reference based alignment and classification of 771 1.8 nm Ni-NTA-Nanogold labeled KaiBC particle images in negative stain. The average used for difference mapping (see ) is marked with an “*” in the upper right corner. (A and B) The number of particles in each projection average is shown in the lower right corner of each average. Side length of panels equals 240 Å.
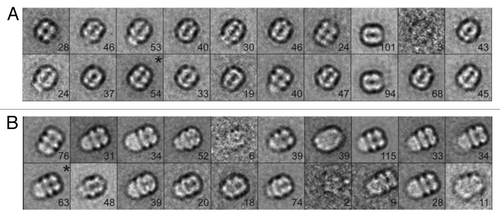
Figure 6. Models of KaiC and KaiBC complex derived from small angle X-ray scattering (SAXS).Citation25 (A) SAXS model of S. elongatus KaiC hexamer, exhibiting a protrusion that marks the location of C-terminal peptides jutting out from the CII ring. (B) SAXS model of the KaiBC complex, consistent with KaiB dimers co-locating with C-terminal peptides and thus sitting on top of the CII ring.
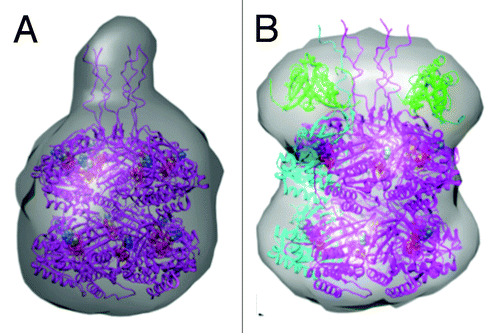
Figure 7. Changing protein-protein interactions over the daily cycle of the KaiABC PTO. KaiA dimer stimulates phosphorylation of KaiC in the hypo-phosphorylated T432/S431 (TS) state by interacting with a CII-terminal peptide via its C-terminal domains (C-KaiA). KaiC is first phosphorylated at T432 residues (pTS) and then also at S431 residues (pTpS = hyper-phosphorylated state). Unlike KaiA, KaiB shows no affinity for the KaiC C-terminal peptide and instead binds to the dome-shaped CII surface, triggering dephosphorylation first of pT432 (TpS) and then of pS431 residues via an ATP synthase mechanism, thus restoring the hypo-phosphorylated state. Dephosphorylation is accompanied by KaiC subunit exchange and the appearance of a ternary KaiABC complex that sequesters KaiA and therefore prevents it from stimulating KaiC phosphorylation. At this stage, KaiA lodges as a monomer on the side of the KaiBC complex, contacting it via its N-terminal (N-KaiA) domain. Each of the three proteins thus undergoes a change in quaternary structure over the 24-h period: KaiC hexamers exchange monomeric subunits, KaiB tetramer splits into dimers, and KaiA dimer splits into monomers.
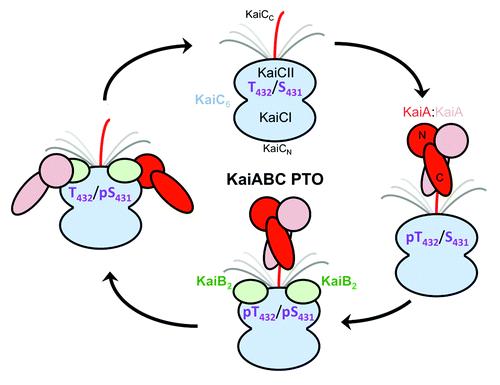