Figures & data
Figure 1. Key variables and system outputs explored in mathematical models. (A) The number of kinases present within the module: one kinase = one-Tiered (1T), 2 kinases = 2-tiered (2T), 3 kinases = 3-tiered (3T) and 4 kinases = 4-tiered (4T). (B) Two modes of activating kinases in the MAPK module: processive phosphorylation [P] (upper panel), where phosphorylation sites within the activation loops are phosphorylated during a single binding step: vs. distributive phosphorylation [D] (lower panel), where only one phosphorylation occurs during binding, and the kinases must re-bind for the second phosphorylation to occur. (C) The 4 different outputs that can be generated from MAPK modules. Classic Michaelis–Menten (MM) kineticsCitation72 where the system is initially linear but then becomes saturate; analog output, which transmit continuous information that is directly proportional to the input stimulusCitation17; switch-like output, which follows a sigmoidal dose-response curve where initially the system is relatively unresponsive and then responds rapidly to inputCitation10,Citation73; and digital output, where the system can only stably exists in one of 2 states, off or onCitation15 with a threshold that defines the switch.
![Figure 1. Key variables and system outputs explored in mathematical models. (A) The number of kinases present within the module: one kinase = one-Tiered (1T), 2 kinases = 2-tiered (2T), 3 kinases = 3-tiered (3T) and 4 kinases = 4-tiered (4T). (B) Two modes of activating kinases in the MAPK module: processive phosphorylation [P] (upper panel), where phosphorylation sites within the activation loops are phosphorylated during a single binding step: vs. distributive phosphorylation [D] (lower panel), where only one phosphorylation occurs during binding, and the kinases must re-bind for the second phosphorylation to occur. (C) The 4 different outputs that can be generated from MAPK modules. Classic Michaelis–Menten (MM) kineticsCitation72 where the system is initially linear but then becomes saturate; analog output, which transmit continuous information that is directly proportional to the input stimulusCitation17; switch-like output, which follows a sigmoidal dose-response curve where initially the system is relatively unresponsive and then responds rapidly to inputCitation10,Citation73; and digital output, where the system can only stably exists in one of 2 states, off or onCitation15 with a threshold that defines the switch.](/cms/asset/066332aa-8fc3-49e5-a6a4-42a802638288/kccy_a_10929349_f0001.gif)
Figure 2. How increasing the number of kinases within the module and processive vs. distributive activating phosphorylation mechanisms change signal output. Different modules that contained increasing number of kinases (1T = one-tiered kinase module, 2T = 2-tiered kinase module etc), with each kinase activated through either processive [P], or distributive [D] phosphorylation, were constructed and their signal outputs tested in silico. The Hill co-efficient [H] for each system was calculated and is displayed in the box within each panel. (A) One-tiered processive (solid black lines) and one-tiered distributive (blue dashed line). (B) Tow tiered processive-procecessive (P-P solid black line), processive-distributive (P-D dashed blue line) and 2 tiered distributive-distributive (DD solid green line). (C) Three tiered processive-processive-distributive (P-P-D solid black line) and processive-distributive-distributive (P-D-D solid green line). (D) Four tiered processive-processive-processive-distributive. (E) Four tiered processive-processive-distributive-distributive. (F) Four tiered processive-distributive-distributive-distributive.
![Figure 2. How increasing the number of kinases within the module and processive vs. distributive activating phosphorylation mechanisms change signal output. Different modules that contained increasing number of kinases (1T = one-tiered kinase module, 2T = 2-tiered kinase module etc), with each kinase activated through either processive [P], or distributive [D] phosphorylation, were constructed and their signal outputs tested in silico. The Hill co-efficient [H] for each system was calculated and is displayed in the box within each panel. (A) One-tiered processive (solid black lines) and one-tiered distributive (blue dashed line). (B) Tow tiered processive-procecessive (P-P solid black line), processive-distributive (P-D dashed blue line) and 2 tiered distributive-distributive (DD solid green line). (C) Three tiered processive-processive-distributive (P-P-D solid black line) and processive-distributive-distributive (P-D-D solid green line). (D) Four tiered processive-processive-processive-distributive. (E) Four tiered processive-processive-distributive-distributive. (F) Four tiered processive-distributive-distributive-distributive.](/cms/asset/b231ff7e-407b-488c-aa47-011fed80c025/kccy_a_10929349_f0002.gif)
Figure 3. Testing module robustness in silico. We used the Hill coefficient to evaluate the robustness of the MAPK module, because the Hill coefficient can be used to define the system output. Robustness was assessed in silico by determining how the Hill co-efficient was maintained in the face of random perturbations to the system. Here we display the box plot of the system outputs (i.e., the value of the Hill co-efficient) in the face of random perturbations.
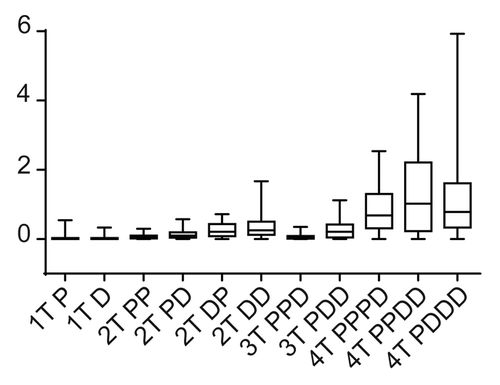
Figure 4. Generating a digital output from different MAPK module topologies using a positive feedback loop. Shown are the system outputs of the 4 most robust module configurations in the presence of a positive feedback loop. The blue solid curve shows the system being activated, with the upward pointing arrow indicating “switching on”. The red dashed line indicates the system becoming inactive after the input is removed, with a downward pointing arrow indicating 'switching off'. The double-headed arrow between the curves shows the presence of hysteresis, which combined with the sharp transition between “off” and “on” states indicates a bistable system output. The module configuration is indicated above each panel with a heading in bold.
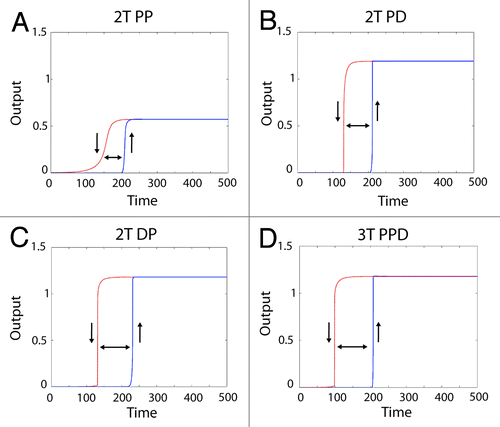
Figure 5. Generating an analog output from the MAPK module using feedback control and a scaffold protein. (A) The 2T P-D system (left panels) and the 3T P-P-D system (right panels) (B) in the presence of a negative feedback loop. The blue line shows the output generated in silico, the red dashed lines shows the output of an ideal Michaelis–Menten system, revealing that both the 2T P-D and 3T P-P-D system outputs closely approximate a Michaelis–Menten system in the presence of a negative feedback loop. (C) The signal output of the 2T processive-distributive module modified using a scaffold plus negative feedback loop (solid-blue-line; scaffold concentration = 1.0: dash-green-line; scaffold concentration = 1.2: dash-dot-red-line; scaffold concentration = 1.4). (D) The signal output of the 3T P-P-D module (solid-blue-line; scaffold concentration = 1.0: dash-green-line; scaffold concentration = 1.2: dash-dot-red-line; scaffold concentration = 1.4).
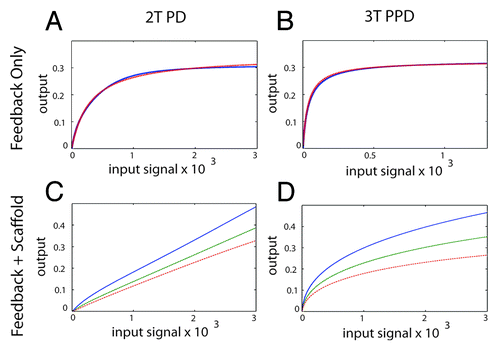
Figure 7. The 3-tiered MAPK module structure in mammals. Mammals have 4 established MAP kinase modules, the canonical ERK MAPK module, the p38, and JNK stress-activated modules, and the ERK5 module. All of the MAPK cascades follow a 3-tiered module configuration, with the proximal MAP Kinase Kinase Kinase (M3K), a central MAP Kinase (M2K) and a terminal MAP Kinase (MK). In general, each module regulates distinct cell phenotypes, with the ERK module controlling cellular proliferation and differentiation, the p38 and JNK modules controlling cellular response to various forms of stress, and the ERK5 module controlling both stress response and cell proliferation. However, MAPK module functions are often overlapping and opposing, and individual MAPK modules can also regulate non-canonical cell phenotypes under certain conditions. The proximal M3K consists of a group of evolutionarily diverse, promiscuous input kinases that link the evolutionarily conserved, non-promiscuous M2Ks to a variety of external stimuli. The M2Ks are tightly linked to their cognate, evolutionarily conserved MAPKs. Once phosphorylated the MAPKs dissociate from their M2K partners and function as the output node of the module, phosphorylating a diverse array of substrates that include transcription factors, phosphatases and other kinases. For detailed reviews of the form and function of mammalian MAPK modules the readers are referred to.Citation6,Citation29
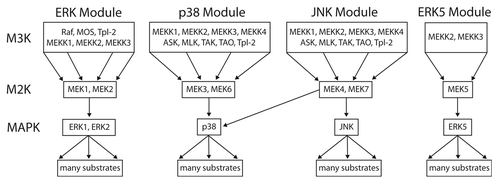
Figure 6. Evolutionary analysis of MAP kinase modules from yeast and man. We restricted our evolutionary analyses to kinases that have been experimentally verified to belong to MAP Kinase modules in humans (Hs) and baker's yeast Saccharomyces cerevisiae (Sc) (reviewed in refs. Citation5, Citation6, Citation27, and Citation28). We conducted a Maximum Likelihood evolutionary analysis on the aligned sequences using Mr Bayes,Citation70 using the standard options available in the Geneious software plugin.Citation71 The Epithelial Growth Factor Receptor (EGFR) from human, fruit fly (Drosophila melanogaster DM) and worm (let-23 Caenorhabditis elegans Ce) were used as out-groups to root the tree (shown in black). The M3K tier of the module is shown in green, the M2K tier shown in blue, and the M1K tier shown in red. The kinase family groups are indicated by lines and named on the right, the kinase groups are shown on the left.
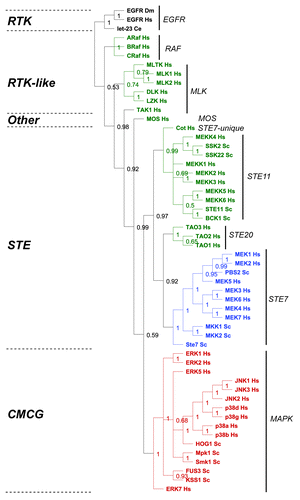