Figures & data
Figure 1 Aging-related increase in PPARγ2 expression in bone marrow stromal cells. (A) Bone marrow stromal cells were collected from adult (6 months old) and old (24 months old) mice and expanded. PPARγ2 expression was measured by real-time PCR. PPARγ2 expression was enhanced in cells from old mice compared to those from adult mice. (B) RNA was extracted from tibia of young (3 mo), adult (6 mo) and old (24 mo) mice. PPARγ2 expression was measured by real-time PCR.
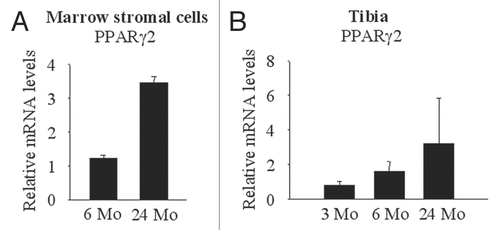
Figure 2 Aging- and rosiglitazone-dependent increase of marrow adiposity and decrease in bone mineral density (BMD). (A) Adipocyte number in the bone marrow was counted in young (3 months), adult (6 months) and old (24 months) mice. Marrow adipocyte number was increased with age, which was further enhanced by the treatment with rosiglitazone (R). (B) Whole body areal bone mineral density (BMD) was measured in young (3 months), adult (6 months) and old (24 months) mice.
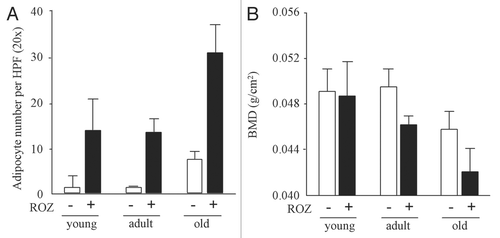
Figure 3 High bone mass and reduced marrow adiposity in Noc-/- mice. (A) Trabecular bone micro-architecture of the distal femur was analyzed by microCT at 16 weeks of animal's age. (B) Marrow adiposity was evaluated in HE-stained femurs of the same animals.
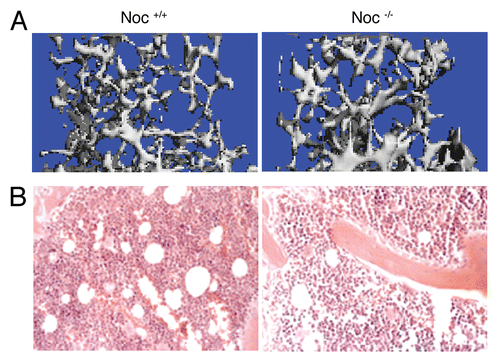
Figure 4 Aging-associated decrease in Igf1 expression in the bone. RNA was collected from tibia of adult (6 months) and old (24 months) mice and Igf1 expression was analyzed by real-time PCR.
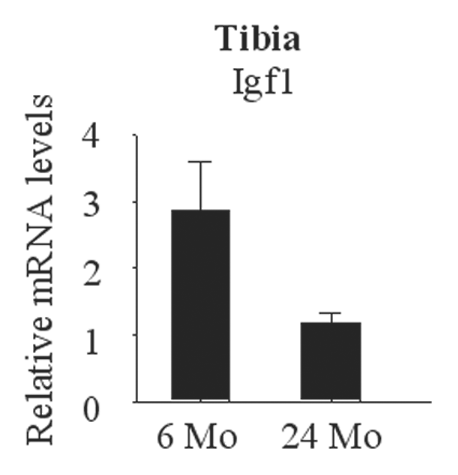
Figure 5 Upregulation of Noc in response to PPAR γ2 activation. U-33 cells stably expressing PPAR γ2 expression vector (U-33/γ2 cells) were treated with Rosiglitazone (ROZ) for 3 days and RNA was collected. Expression of Noc was analyzed by real-time PCR.
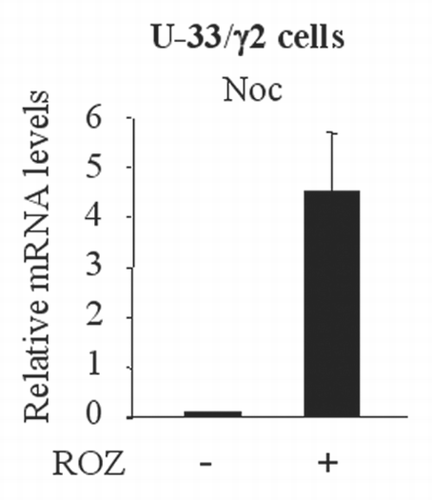
Figure 6 Anti-phase circadian expression pattern between Noc and Igf1 in the femur. RNA was collected from femur at the several time points of the day and expression of Noc and long-form Igf1 were analyzed by real-time PCR. White bar and black bar represent daytime (ZT 0–14) and night time (ZT 14–24), respectively.
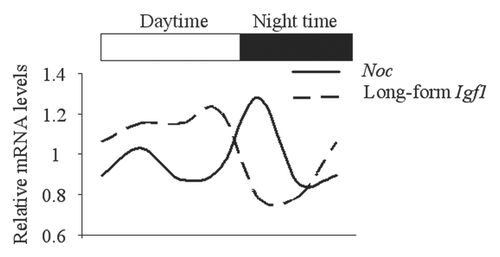
Figure 7 Noc expression was unchanged during the process of aging in the bone marrow stromal cells. Bone marrow stromal cells were collected from adult (6 months old) and old (24 months old) mice and expanded. Noc expression was measured by real-time PCR.
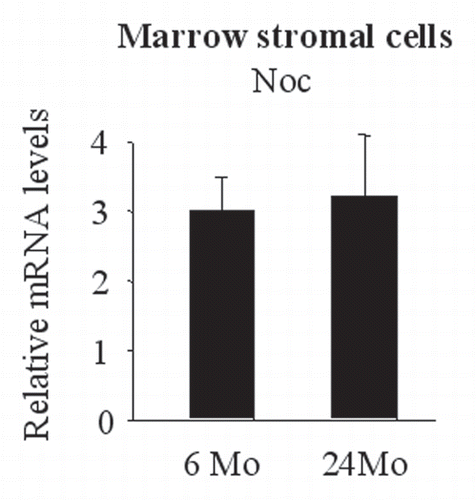
Figure 8 Schematic model of Noc, PPARγ and Igf1 network in the bone marrow. The fate of mesenchymal stem cells (MSCs) is regulated by a number of transcription factors. PPARγ and C/EBPs regulates the specification of MSCs toward the adipogenic lineage, while Runx2, Osterix and Dlx5 favors osteoblastogenesis. Circadian-regulated gene, Nocturnin (Noc), increases PPARγ activity in part by stimulating its nuclear translocation and enhances adipogenesis. In contrast, Noc is a negative regulator for osteoblastogenesis. Noc downregulates Igf1 expression probably through targeting the long-form 3′ UTR of Igf1 transcripts, resulting in the decrease in IGF-I protein levels in the skeletal microenvironment. Because IGF-I is a pivotal factor for skeletal metabolism, Nocinduced bone loss may be in part explained by its activity to decrease IGF-I levels. Importantly, Noc is induced by external stimuli such as high-fat diet and rosiglitazone, thus proposing the possibility that Noc is a circadian factor linking external stimuli and cellular metabolic outputs. PPARγ, Peroxisome proliferator-activated receptor-gamma; C/EBP, CCAAT enhancer binding protein; Runx2, Runt-related transcription factor 2; Dlx5, Distal-less homebox homolog 5; Msx2, Muscle segment homeobox homolog of 2.
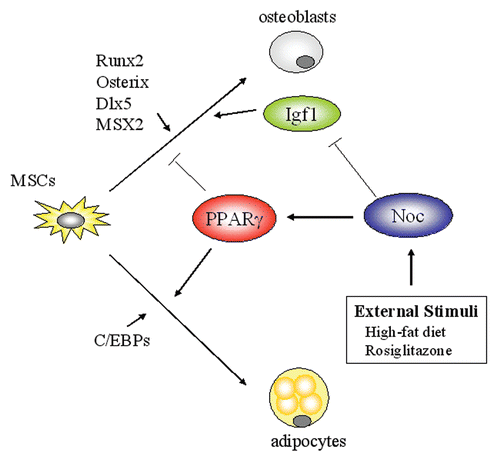