Figures & data
Figure 1 Model potential energy diagrams and Arrhenius plots for the rate or kinetics of microbial “social” decision making. (A) Under preferential attachment rules, decisions to switch from one social strategy to another of less fitness may require “energy absorption” analogous to endothermic chemical reactions. The initial fitter strategy or reactant (Rs) maintains a lower potential energy. Forward transition to a less fit strategy or product (Ps) of higher potential energy may proceed over the reaction barrier via an intermediate or transition state (Ts) or through the reaction barrier without a transition state. The former case is a classical reaction needing activation energy (Ea) and a change in the system's heat (ΔH). The latter case is a quantum reaction, tunneling through the reaction barrier. Arrhenius kinetics (inset) of the classical reaction (black) form a linear relationship between the rate of strategy switching (k) and the inverse of the system's temperature (1/T), whereas the quantum reaction (grey) produces a nonlinear function between the same variables. (B) Social decision making by microbes may also require “energy dissipation” analogous to exothermic chemical reactions. Here the initial less fit strategy (Rs) of higher potential energy transitions to a more fit strategy (Ps) of lower potential energy using the classical or quantum principles described for (A). The Arrhenius kinetics (inset) of exothermic reactions give plots identical to that of endothermic reactions.
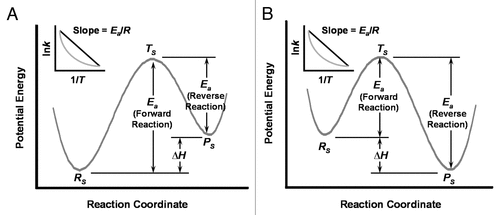
Figure 2 Arrhenius plots for learned “courting” decisions made by Spirostomum ambiguum during simulated “social” trials. (A) S. ambiguum that respond with initial low (top panel), medium (middle panel), or high (bottom panel) responsiveness learn to encourage or discourage mating interactions by emitting serial contraction strategies of differential fitness. When the rate or kinetics of strategy switching are dominated by quantum Bose-Einstein (BE, grey diamond) or Fermi-Dirac (FD, white diamond) statistical distributions, Arrhenius plots show pronounced nonlinearity consistent with quantum-like tunneling in decision making processes. When a classical Maxwell-Boltzmann (MB, black diamond) statistical distribution dominates social decision making by S. ambiguum, plots yield a pronounced linear function consistent with classical, thermodynamic-sensitive strategy switching. (B) S. ambiguum that respond with initial medium (middle panel) or high (bottom panel) responsiveness also learn to encourage or discourage mating interactions by emitting serial ciliary reversal strategies of differential fitness. Rates of strategy switching are dominated by quantum FD (white diamond) and BE (black diamond) statistical distributions with pronounced nonlinear Arrhenius kinetics consistent with quantum-like tunneling. For (A) and (B), temperature T was substituted for the “critical tunneling field strength”, Γ, an annealing parameter directly related to the critical condensation temperature TC by ΓC = (TCkB)/[n/ζ(3/2)]2/3, where kB is Boltzmann constant, ζ is the Riemann zeta function, and n is the “particle” density.
![Figure 2 Arrhenius plots for learned “courting” decisions made by Spirostomum ambiguum during simulated “social” trials. (A) S. ambiguum that respond with initial low (top panel), medium (middle panel), or high (bottom panel) responsiveness learn to encourage or discourage mating interactions by emitting serial contraction strategies of differential fitness. When the rate or kinetics of strategy switching are dominated by quantum Bose-Einstein (BE, grey diamond) or Fermi-Dirac (FD, white diamond) statistical distributions, Arrhenius plots show pronounced nonlinearity consistent with quantum-like tunneling in decision making processes. When a classical Maxwell-Boltzmann (MB, black diamond) statistical distribution dominates social decision making by S. ambiguum, plots yield a pronounced linear function consistent with classical, thermodynamic-sensitive strategy switching. (B) S. ambiguum that respond with initial medium (middle panel) or high (bottom panel) responsiveness also learn to encourage or discourage mating interactions by emitting serial ciliary reversal strategies of differential fitness. Rates of strategy switching are dominated by quantum FD (white diamond) and BE (black diamond) statistical distributions with pronounced nonlinear Arrhenius kinetics consistent with quantum-like tunneling. For (A) and (B), temperature T was substituted for the “critical tunneling field strength”, Γ, an annealing parameter directly related to the critical condensation temperature TC by ΓC = (TCkB)/[n/ζ(3/2)]2/3, where kB is Boltzmann constant, ζ is the Riemann zeta function, and n is the “particle” density.](/cms/asset/5f28cd3d-1101-411d-b4c6-cf41a34a26ca/kcib_a_10912842_f0002.gif)