Figures & data
Figure 1 Subcellular compartmentalization of a mouse sperm cell. (A) Schematic diagram of a murine sperm as an example of spermatozoon compartmentalization: The sperm head, with the acrosomal vesicle and the condensed nucleus and the flagellum with the mitochondrial-enriched mid-piece region, the principal piece and end piece segment are shown. The red dotted line demonstrates the separation between head and flagellum. (B) Hypothetical working model illustrating capacitation-dependent reorganization of lipid rafts in the plasma membrane of the acrosomal region. The two schematic drawings of the murine sperm head (upper and lower left part) show the distribution and size of raft plasma membrane platforms in the whole acrosomal region. In freshly ejaculated spermatozoa (upper parts) lipid rafts, enriched in sphingolipids (green) and cholesterol (blue), are uniformly distributed in the plasma membrane (pm) overlaying the whole acrosomal cap. In contrast, following capacitation (lower parts), rafts are more concentrated in a crescent shaped region of the sperm head. Following the size and location of rafts at higher magnification (right parts), it becomes obvious that rafts in the anterior region of the acrosomal cap in capacitated sperm aggregate upon maturation, thus leading to large-scale and stable raft clusters in the plasma membrane. Since lipid rafts in sperm recruit ZP-binding receptors as well as components of the SNARE-fusion machinery, this area may present the preferential site of hybrid vesicle formation during acrosomal secretion. This structural organization resembles the one at the active zone of the presynaptic terminal in neurons where neurotransmitter vesicle fusion occurs and thus may be termed “acrosomal synapse”. The cholesterol efflux from the plasma membrane during capacitation has been suggested to occur from the non-raft fraction and is illustrated by far less cholesterol molecules in the lower right part compared to the upper one. Possible lipid rafts in the outer acrosomal membrane (oam), and the tighter association between the two membranes, which has been suggested to occur upon capacitation, are not shown.
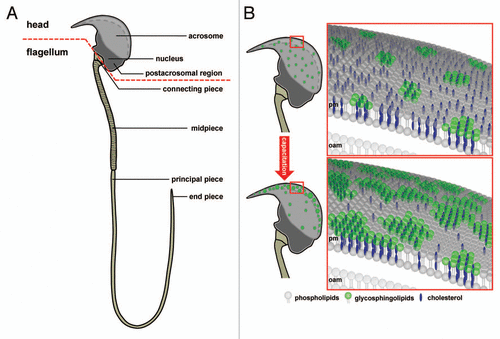
Figure 2 Subcellular localization and possible function of MUPP1 in spermatozoa. (A) Confocal laser scanning micrographs showing the subcellular localization of MUPP1 in spermatozoa of different mammalian species. Isolated spermatozoa from different mammalian species (mouse, rat, bull and man) were fixed and incubated with a specific antibody recognizing MUPP1. Antibody binding was visualized using a fluorescein-conjugated anti-rabbit IgG (green). Sperm nuclei were counter-stained with propidium iodide (PI, red). Note that MUPP1 expression in all analyzed species does not overlap with the propidium iodide labeling of the nucleus but is restricted to the acrosomal region of the sperm head and the equatorial segment (bull, man). The inserts indicate a region shown at higher magnification in the lower parts. Photomicrographs show overlays of the green and red channel and the transmitted light channel. Scale bars for the upper parts: mouse, rat: 20 µm; bull, man: 10 µm. Scale bars for the lower parts: mouse, rat and bull: 5 µm; man: 2 µm. (B) working model illustrating a possible functional role of a MUPP1/CaMKIIα complex in preventing spontaneous acrosome reaction during the sequential fusion steps of SNARE-promoted acrosomal exocytosis. The model summarizes the molecular rearrangements and modifications of proteins and lipids involved in the acrosomal fusion process, first depicting a pre-fusion state (upper part) and second at the time after fusion of the outer acrosomal membrane and the plasma membrane (lower part). After capacitation, the acrosomal vesicle has been suggested to be in a pre-fusion stage which is reflected by pre-assembled trans-SNARE complexes which force the outer acrosomal membrane (oam, grey) and the adjacent plasma membrane (pm, dark grey) to close proximity (see also insert on the left). At this stage, a catalytically active CaMKIIα, recruited by the PDZ domains 10 and/or 11 of MUPP1, is “freezing” the acrosomal exocytotic process in this intermediate fusion state, thereby preventing “accidental” spontaneous acrosomal secretion. whether this CaMKIIα/MUPP1 mediated fusion clamp is the result of a CaMKII-catalyzed phosphorylation of proteins participating in the sequential Ca2+-controlled secretion pathway, like SNARE-components and/or ion channels, is currently not known. Furthermore, it is not clear whether the observed co-localization of CaMKIIα and MUPP1 in detergent resistant membrane platforms (green) is necessary to position the kinase and possibly also its “interlocking” target/s to a defined space between the outer acrosomal membrane and the plasma membrane. Upon sperm/egg interaction, Zona pellucida ligand/s bind to complementary receptors on the capacitated sperm plasma membrane (ZP-R), thus inducing an initial transient influx of Ca2+ mediated by voltage-gated channels (Cav) and a subsequent sustained increase in Ca2+ concentration mediated by the interplay of inositol-1,4,5-trisphosphate receptors (IP3-R) on the outer acrosomal membrane and transient receptor potential channels (TRP) in the plasma membrane (bottom part). A local increase in Ca2+ (blue spots) and the formation of Ca2+ occupied calmodulin (CaM) might then be able to release the kinase from the PDZ scaffolding protein, thereby unfreezing the CaMKIIα/MUPP1 clamp. At this point, Ca2+ also triggers the final steps of membrane fusion, coinciding with the formation of hybrid vesicles at multiple sites of the membrane (see insert on the left), controlled by the calcium sensor protein synaptotagmin (synt). For the sake of simplicity, not all known molecules, which are functionally operative in the acrosome reaction (e.g., cAMP, Enkurin, EPAC, NSF, PLCδ, PI3K, Rab3A) are depicted. Localization of Q- and R-SNAREs is plotted according to reference Citation125. Cav, voltage-activated Ca2+ channel; CaMKIIα, Ca2+/calmodulin-dependent kinase IIα; IP3-R, inositol 1,4,5-trisphosphate receptor; MUPP1, Multi PDZ domain Protein 1; TRP, transient receptor potential; VAMP, vesicle-associated membrane protein/synaptobrevin; ZP-R, ZP-receptor.
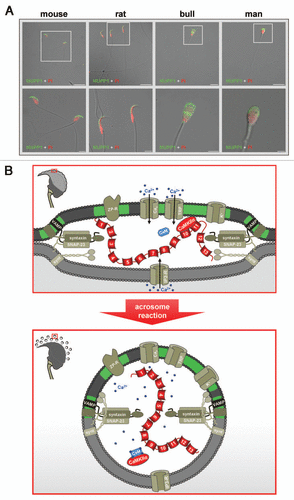