Figures & data
Figure 1. Aberrant expression of LINE-1 retrotransposons in breast cancer cells. (A) The LINE-1-encoded ORF1 protein was detected by western blotting of whole-cell lysates from normal and breast cancer cells. NTera.2D1 human embryonic carcinoma cells were used as positive controls. For protein normalization, mouse α-tubulin was used as a loading control. (B) The LINE-1 transcripts (L1 mRNAs) derived from the LINE-1 ORFs were detected via qRT-PCR with primers specific for the LINE-1 ORF1 and ORF2 sequences. The data are shown as the fold change compared with the HMECs after normalization to the HPRT1 housekeeping gene. Each point represents the average from four independent experiments. Unpaired t-test, p = 0.002. Error bars indicate s.d. (n = 4).
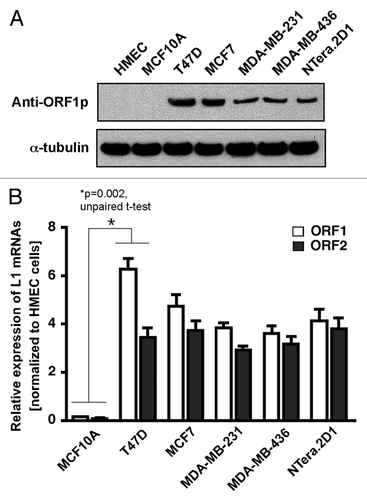
Figure 2. The LINE-1 retrotransposition cassette. (A) Schematic diagrams of the pRP99-L1-EGFP expression cassettes used for LINE-1 retrotransposition assays. LINE-1 transcription is driven by its own 5′UTR, which harbors an internal promoter. This LINE-1 retrotransposon contains an intron-interrupted EGFP reporter in the 3′UTR region with its own CMV promoter and polyadenylation signal. The EGFP indicator cassette is in an antisense orientation relative to LINE-1. Only when EGFP is transcribed from the LINE-1 promoter, spliced, reverse transcribed and integrated into the genome does a cell become EGFP positive. Arrows depict the location of the geno-5 (left) and geno-3 (right) primers used in the PCR assay shown below. SD, splice donor; SA, splice acceptor. (B) Detection of sustained retrotransposition events in breast cancer cells. The number of EGFP-positive cells was plotted over time for pRP99-L1-EGFP transfected cells. Each time point represents cell populations from three independent experiments. Sampling and analysis were performed at 1, 3, 5, 8, 12, and 15 d after the plating of transfected cells. The X-axis indicates the number of EGFP-positive cells per 105 cells analyzed. The error bars indicate s.d (C) PCR analysis of retrotransposed cells. The geno-5 and geno-3 primers, flanking the intron in EGFP, were used for PCR amplification of genomic DNA, and the obtained products were analyzed on a 1.2% agarose gel. PCR products of 1.49 kb (corresponding to the intron-containing vector) and 530 bp (corresponding to the retrotransposed insertion that lacks the 909 bp intron) are shown. As a negative control, genomic DNA from the parental cells (P) was used. The symbols + and – represent the active LINE-1 (pRP99-L1-EGFP) and the inactive LINE-1 (pRP-ΔL1-EGFP) transfected cells, respectively. Vector, 1 ng plasmid DNA; Marker, 1 kb-plus DNA marker.
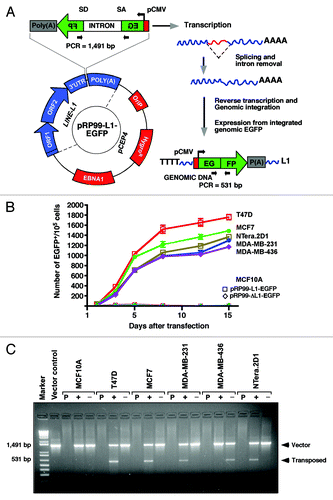
Figure 3. Depletion of Dicer1 and Ago2 activates LINE-1 expression. (A) Quantitative real-time RT-PCR analysis of endogenous Dicer1 and Ago2 mRNAs in normal MCF10A cells that were stably transfected with shRNAs targeting the Dicer1 and Ago2 genes. An shRNA targeting the luciferase gene was used as a negative control. The data are shown as the relative fold changes of Dicer1 and Ago2 mRNAs with respect to the control HPRT1. (B) western blot of endogenous Dicer1 and Ago2 proteins knocked down in MCF10A cells. α-tubulin was used as an internal control. (C) Dicer1- and Ago2-depleted MCF10A cells were transiently transfected with the pRP99-L1-EGFP expression cassette. The analyses of the parental MCF10A cells and cells transfected with control shRNA were performed in parallel. EGFP-expressing cells were readily detected 3 d after transfection. The EGFP signal was digitally overlaid on the corresponding phase-contrast image. (D) The time course of LINE-1 retrotransposition events in Dicer1- and Ago2-knockdown cells. Number of gated EGFP-positive cells recorded at various time points. Each time point represents cell populations from three independent transfections. The error bars indicate s.d. (n = 3). (E) Confirmation of retrotransposition events using PCR, as revealed by a 531 bp band observed in MCF10A cells in which the Dicer1 and Ago2 genes were depleted, but not in cells treated with control shRNA or parental MCF10A cells. The 1,491 bp band represents the intron-containing pRP99-L1-EGFP vector.
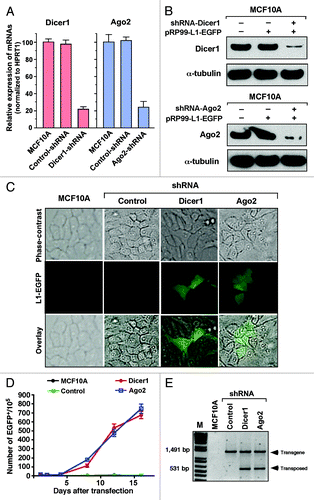
Figure 4. Comparison of LINE-1-specific endo-siRNA abundance in MCF10A and T47D cells. (A) Read counts of endo-siRNAs and their binding sites on LINE-1 (L1) retrotransposons are shown. (B) Structure of the LINE-1Hs 5′-UTR showing the sense (SP) and antisense (ASP) promoters within the ~906 nucleotides of the promoter. Transcription start sites are given, numbered according to the sense strand. Known transcription start sites of ASP involved in the regulation of LINE-1 are denoted by closed dot symbols. Grey arrows indicate the bidirectional transcripts of the LINE-1 5′-UTR. Sequences of two differentially expressed endo-siRNAs, read counts, and their binding positions on the LINE-1 5′–UTR sequences are shown.
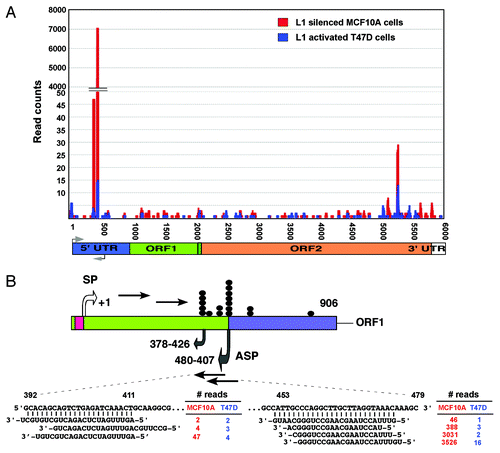
Figure 5. Endo-siRNA mediates LINE-1 silencing. (A) northern blot analysis of endo-siRNAs. Low MW total RNAs were probed with the sense strand of endo-siRNA sequences, and the resulting signals were detected after exposure to X-film for 3 d. As a small RNA loading control, hsa-miR-16 was used. Labeled Decade RNA markers were used as size markers. (B) Differential expression of endo-siRNAs in various types of breast cancer cells determined using real-time qRT-PCR. The fold changes were determined by comparing the ∆CT value of each endo-siRNA after normalization to the control Sno37A small RNA. The data are shown as the ratio of the mean signal in cancer cells/mean signal in normal cells. A negative value indicates reduced expression of endo-siRNAs in cancer cells compared with MCF10A cells. (C) A schematic of the construct used for the dual luciferase report assay is shown in the top panel. The synthetic siRNAs used in this study are shown at the bottom. Luciferase reporter assay detecting expression from in HEK293T cells transfected with scrambled, endo453 and endo392 siRNAs. Cells transfected with firefly luciferase (under control of the LINE-1Hs 5′-UTR) and the Renilla luciferase reporter plasmid without any siRNA sequences were used as a negative control. The firefly luciferase activity was normalized to the Renilla luciferase activity. The data are shown as the relative luciferase activity of endo-siRNA-treated cells with respect to the control cells. Unpaired t-test, p = 0.005. Error bars indicate s.d. (n = 9). (D) Real-time RT-PCR analysis of the transcript abundance of LINE-1 (L1) mRNAs (ORF1 and ORF2) in normal MCF10A cells and various types of breast cancer cells after being stably transfected with shRNA constructs that encode the endo453 and endo392 sequences. Negative controls containing control shRNA or no shRNA were performed in parallel. The relative LINE-1 mRNA levels were determined after normalizing the data to the control HPRT1 gene. Genomic DNA isolated from HMEC tissue was used as an additional control. Lane 1, parental cells; lane 2, control shRNAs; lane 3, shRNAs encoding endo453; and lane 4, shRNAs encoding endo392. Error bars indicate s.d.
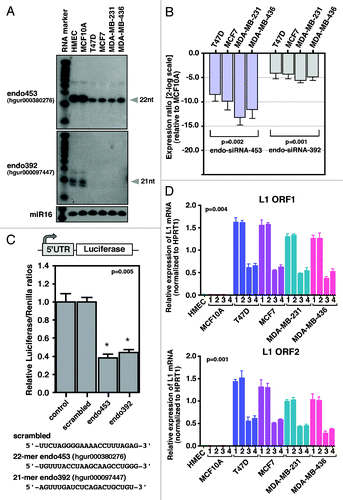
Figure 6. DNA methylation analysis. (A) Schematic representation of the CpG dinucleotide distribution within the 5′UTR of the LINE-1Hs promoter from +1 to +906 bp (relative to the transcription start site). Vertical lines above indicate the position and numbering of CpG sites; the box represents the CpG island in the LINE-1 promoter (ending at +560 bp). The positions of the primer sets used for methylated DNA immunoprecipitation (MeDIP) and bisulfite sequencing are shown as arrows. (B) MeDIP analysis of LINE-1 5′-UTR methylation patterns. The data are shown as the percentage of methylated DNA relative to the total input for primer sets I and II. Each point represents averages from three experiments. p values were calculated by a one-way analysis of variance. Genomic DNA from HMEC tissue was used as a positive control. The parental cells and cells transfected with control shRNA were analyzed in parallel. Lane 1, parental cells; lane 2, control shRNAs; lane 3, shRNAs encoding endo453; and lane 4, shRNAs encoding endo392. (C) Bisulfite sequence analysis of the LINE-1Hs 5′-UTR region. A region of 560 bp containing 31 CpG sites was sequenced, and the methylation status of individual CpG sites was analyzed within the LINE-1 CpG island. Open and closed circles denote unmethylated and methylated CpG-sites, respectively. Gray represents the unresolved CpG sites. The corresponding percentage of overall CpG methylation is illustrated below, as determined by BDPC analysis.
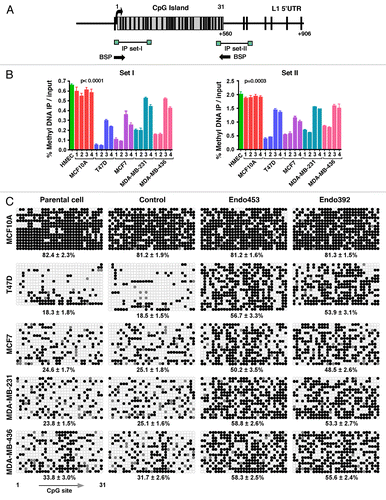