Figures & data
Figure 1. Time lapse studies monitoring lipid droplet formation in dispersed islet cells. Phase contrast digital images of dispersed islet cells treated with 25 mM glucose and 500 μM FFAs (oleate:palmitate = 1:1v/v) were acquired at 30 min intervals for 40 hours using a 20× objective. Representative images at the indicated time (h) are shown. An enlarged image indicated by the white arrow (far right panel) demonstrates that lipid droplets were uniform in size. Images were rescaled using Adobe Photoshop software. Results are representative of three independent experiments.
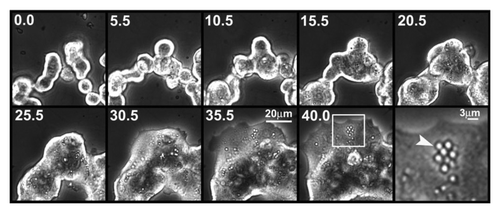
Figure 2. (A) Time course of lipid droplet formation in dispersed rat β-cells. Dispersed rat islet cells (105) were treated for the indicated time periods with 25 mM glucose and 500 μM FFAs. After treatment, cells were processed for immunostaining. Insulin (red), nuclei (blue), and Nile red staining (green) are shown. (B) The bar graph shows the average Nile red intensity for a total of ~80 clusters of insulin-positive cells per time point from three independent experiments is shown. (C) Dose-dependent effects of glucose and FFAs on lipid droplet formation. Dispersed rat islet cells (105) were treated for 4 days as indicated. After treatment, cells were processed for immunostaining. Insulin (red), nuclei (blue), and Nile red staining (green) are shown. 5.6G and 240FFA denote 5.6 mM glucose and 240 μM FFAs, respectively. (D) The bar graph shows the average Nile red intensity for a total of ~80 clusters of insulin-positive cells per condition from three independent experiments.
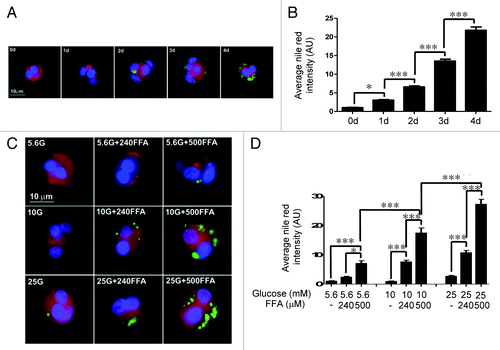
Figure 3. Nutrient-mediated activation of mTORC1. Rat islets (100) were treated for 4 days as indicated. Samples were processed for Western blotting for phosphorylated S6, stripped, and reblotted for β-actin. Band intensities were quantitated by Biorad ChemiDoc XRS Image Lab Software. The ratio of p-S6/β-actin was obtained as described in the Methods section. Data are the means ± SEM of n = 3 experiments.
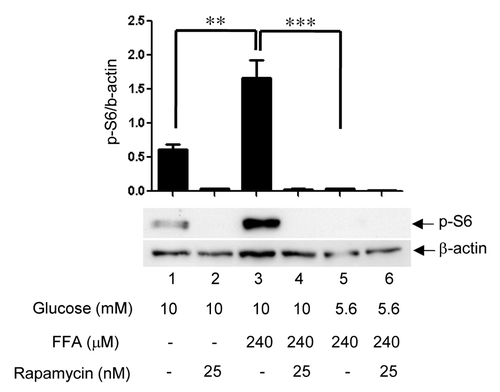
Figure 4.(A) mTORC1-dependent TG accumulation. Rat islets (150) were cultured for 4 days as indicated. After treatment, lipid extraction by chloroform:methanol was performed and TG quantitated. Data are the means ± SEM of n = 3 experiments with duplicate samples in each experiment. (B) mTORC1 mediates lipid droplet formation exclusively in β-cells, sparing α-cells. Dispersed rat islet cells (105) were cultured for 4 days in cCMRL-1066 containing 5.6 mM glucose, 25 mM glucose + 500 μM FFAs, or 25 mM glucose + 500μM FFAs+25 nM rapamcyin. After treatment, cells were processed for immunostaining. Representative images of β-cells (panels a, b and c) and α-cells (panels d, e and f) under the three conditions [insulin and glucagon (red), nuclei (blue), and Nile red staining (green)]. (C) The bar graph shows the average Nile red intensity of a total of ~50 β- or α-cells per condition from three independent experiments is shown.
![Figure 4.(A) mTORC1-dependent TG accumulation. Rat islets (150) were cultured for 4 days as indicated. After treatment, lipid extraction by chloroform:methanol was performed and TG quantitated. Data are the means ± SEM of n = 3 experiments with duplicate samples in each experiment. (B) mTORC1 mediates lipid droplet formation exclusively in β-cells, sparing α-cells. Dispersed rat islet cells (105) were cultured for 4 days in cCMRL-1066 containing 5.6 mM glucose, 25 mM glucose + 500 μM FFAs, or 25 mM glucose + 500μM FFAs+25 nM rapamcyin. After treatment, cells were processed for immunostaining. Representative images of β-cells (panels a, b and c) and α-cells (panels d, e and f) under the three conditions [insulin and glucagon (red), nuclei (blue), and Nile red staining (green)]. (C) The bar graph shows the average Nile red intensity of a total of ~50 β- or α-cells per condition from three independent experiments is shown.](/cms/asset/77343559-911f-4275-966f-c29b8fd00d0e/kisl_a_10922720_f0004.gif)
Figure 5.(A) Rat islets (50) were cultured for 4 days in cCMRL containing 5.6 mM glucose, 25 mM glucose + 500 μM FFAs, or 25 mM glucose + 500 μM FFAs + 25 nM rapamycin. Frozen sections (5 μm) of islets were processed for immunostaining [insulin (green), nuclei (blue), and Nile red (red)] as described in the Methods section. Images were rescaled using Adobe Photoshop software. (B) The bar graph shows the average intensities of Nile red for lipid accumulation and DyLight 647 for insulin, respectively, of 15–20 islet sections per condition from three different experiments.
![Figure 5.(A) Rat islets (50) were cultured for 4 days in cCMRL containing 5.6 mM glucose, 25 mM glucose + 500 μM FFAs, or 25 mM glucose + 500 μM FFAs + 25 nM rapamycin. Frozen sections (5 μm) of islets were processed for immunostaining [insulin (green), nuclei (blue), and Nile red (red)] as described in the Methods section. Images were rescaled using Adobe Photoshop software. (B) The bar graph shows the average intensities of Nile red for lipid accumulation and DyLight 647 for insulin, respectively, of 15–20 islet sections per condition from three different experiments.](/cms/asset/06bf8ca6-c7da-47d4-9766-ae1100ec0a94/kisl_a_10922720_f0005.gif)
Figure 6.(A) Dose-dependent effects of nutrients on ADRP expression. Rat islets (100) were cultured for 4 days in cCMRL-1066 containing 5.6 mM glucose, 10 mM glucose + 240μM FFAs, or 25 mM glucose + 500 μM FFAs. Samples were processed for Western blotting and quantitated by densitometry. β-actin was used as a protein loading control. Data are the means ± SEM of n = 3 experiments. (B) Glucose and FFAs synergize in increasing mTORC1-mediated ADRP expression. Rat islets (100) were cultured for 4 days in cCMRL-1066 under various conditions as indicated. Samples were processed for western blotting and quantitated by densitometry. β-actin was used as a protein loading control. Data are the means ± SEM of n = 3 experiments. (C) ADRP expression and insulin content in single β-cells determined by immunohistochemistry. Dispersed rat islet cells (105) were cultured for 4 days in cCMRL-1066 containing 5.6 mM glucose, 25 mM glucose + 500 μM FFAs, or 25 mM glucose + 500μM FFAs + 25 nM rapamcyin. After treatment, cells were processed for immunostaining. Representative images of β-cells under the three conditions are shown [insulin (green), nuclei (blue), and ADRP (red)]. (D) The bar graph shows the average intensities of DyLight 647 for insulin and Dylight 488 for ADRP, respectively, of a total of ~80 β-cells per condition from three independent experiments.
![Figure 6.(A) Dose-dependent effects of nutrients on ADRP expression. Rat islets (100) were cultured for 4 days in cCMRL-1066 containing 5.6 mM glucose, 10 mM glucose + 240μM FFAs, or 25 mM glucose + 500 μM FFAs. Samples were processed for Western blotting and quantitated by densitometry. β-actin was used as a protein loading control. Data are the means ± SEM of n = 3 experiments. (B) Glucose and FFAs synergize in increasing mTORC1-mediated ADRP expression. Rat islets (100) were cultured for 4 days in cCMRL-1066 under various conditions as indicated. Samples were processed for western blotting and quantitated by densitometry. β-actin was used as a protein loading control. Data are the means ± SEM of n = 3 experiments. (C) ADRP expression and insulin content in single β-cells determined by immunohistochemistry. Dispersed rat islet cells (105) were cultured for 4 days in cCMRL-1066 containing 5.6 mM glucose, 25 mM glucose + 500 μM FFAs, or 25 mM glucose + 500μM FFAs + 25 nM rapamcyin. After treatment, cells were processed for immunostaining. Representative images of β-cells under the three conditions are shown [insulin (green), nuclei (blue), and ADRP (red)]. (D) The bar graph shows the average intensities of DyLight 647 for insulin and Dylight 488 for ADRP, respectively, of a total of ~80 β-cells per condition from three independent experiments.](/cms/asset/abc18697-3651-4530-b34d-8a93ce7093fa/kisl_a_10922720_f0006.gif)
Figure 7.(A) mTORC1-dependent increase in rat islet size under nutrient overload. Phase contrast images of rat islets (40) before and after the 4 days incubation period under various conditions as indicated were acquired using a 20× objective. The diameter of each islet was computed by converting pixels to μm using the Metamorph image analysis software. The white and the black bars indicate the sizes of the islets before and after the treatment, respectively. Data are the means ± SEM of n = 3 experiments. (B) mTORC1-dependent new DNA synthesis in rat islets under nutrient overload. Rat islets (100) were cultured for 4 days under various conditions as indicated. [3H]thymidine was added to each dish 24 h prior to the end or the 4-day period. Data are the means ± SEM of n = 3 experiments. dpm, disintegrations per minute. (C) Islet cell expansion under nutrient overload determined by immunohistochemistry. Rat islets (50) were cultured for 4 days in cCMRL containing 5.6 mM glucose, 25 mM glucose + 500 μM FFAs, or 25 mM glucose + 500 μM FFAs+25 nM rapamycin. Frozen sections (10 μm) of islets were processed for immunostaining (insulin [red], nuclei [blue], and phalloidin [green]). Images were rescaled using Adobe Photoshop software. (D) The bar graph shows the average area of intra-islet cells determined using the Metamorph image analysis software. Data are the means ± SEM of ~250 cells obtained from 10–15 islet sections per condition.
![Figure 7.(A) mTORC1-dependent increase in rat islet size under nutrient overload. Phase contrast images of rat islets (40) before and after the 4 days incubation period under various conditions as indicated were acquired using a 20× objective. The diameter of each islet was computed by converting pixels to μm using the Metamorph image analysis software. The white and the black bars indicate the sizes of the islets before and after the treatment, respectively. Data are the means ± SEM of n = 3 experiments. (B) mTORC1-dependent new DNA synthesis in rat islets under nutrient overload. Rat islets (100) were cultured for 4 days under various conditions as indicated. [3H]thymidine was added to each dish 24 h prior to the end or the 4-day period. Data are the means ± SEM of n = 3 experiments. dpm, disintegrations per minute. (C) Islet cell expansion under nutrient overload determined by immunohistochemistry. Rat islets (50) were cultured for 4 days in cCMRL containing 5.6 mM glucose, 25 mM glucose + 500 μM FFAs, or 25 mM glucose + 500 μM FFAs+25 nM rapamycin. Frozen sections (10 μm) of islets were processed for immunostaining (insulin [red], nuclei [blue], and phalloidin [green]). Images were rescaled using Adobe Photoshop software. (D) The bar graph shows the average area of intra-islet cells determined using the Metamorph image analysis software. Data are the means ± SEM of ~250 cells obtained from 10–15 islet sections per condition.](/cms/asset/7bf6926c-90b8-4f3b-910a-688f06ab8c11/kisl_a_10922720_f0007.gif)
Figure 8.(A) Insulin release into the culture medium under nutrient overload. Islets (35) were cultured in 2 ml cCMRL containing 10 mM glucose or 240 μM FFAs alone or in combination in the absence and presence of 25 nM rapamycin. Cultured medium (50 μl) was removed at the indicated times and the amount of insulin released into the culture medium was determined by radioimmunoassay. Data are the means ± SEM of n = 3 experiments. (B) Insulin content in islets after 4 days treatment under nutrient overload. After treatment, islets were washed and resuspended in 500 μl of 0.1% BSA in PBS, followed by sonication using a microtip probe. Insulin content was determined by radioimmunoassay. Data are the means ± SEM of n = 3 experiments with triplicate samples in each experiment. (C) Pro-insulin levels in islets after 4 days treatment under nutrient overload. After treatment, islets were washed and resuspended in 200 μl of 0.1% BSA in PBS, followed by sonication using a microtip probe. Pro-insulin levels were determined as described in the Methods section. Data are the means ± SEM of n = 3 experiments.
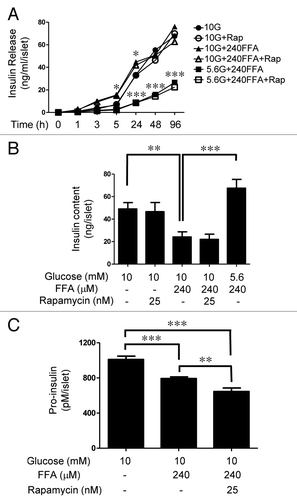
Figure 9.(A) Time course of lipid droplet formation in dispersed human β-cells. Dispersed human islet cells (105) were treated for the indicated time periods with 25 mM glucose and 500 μM FFAs. After treatment, cells were processed for immunostaining. The average Nile red intensity for a total of ~80 clusters of insulin-positive cells per time point from three independent experiments is shown. (B) Nutrient-mediated activation of mTORC1. Human islets (100) were treated for 4 days as indicated. Samples were processed for Western blotting and quantitated by densitometry. Data are the means ± SEM of n = 3 experiments. (C) Human islets (50) were cultured for 4 days in cCMRL containing 5.6 mM glucose, 25 mM glucose + 500 μM FFAs, or 25 mM glucose + 500 μM FFAs + 25 nM rapamycin. Frozen sections (10 μm) of islets were processed for immunostaining [insulin (green), nuclei (blue), and Nile red (red)]. Images were rescaled using Adobe Photoshop software. (D) The bar graph shows the average intensities of Nile red for lipid accumulation and DyLight 647 for insulin, respectively, of 15–20 islet sections per condition from three different experiments is shown.
![Figure 9.(A) Time course of lipid droplet formation in dispersed human β-cells. Dispersed human islet cells (105) were treated for the indicated time periods with 25 mM glucose and 500 μM FFAs. After treatment, cells were processed for immunostaining. The average Nile red intensity for a total of ~80 clusters of insulin-positive cells per time point from three independent experiments is shown. (B) Nutrient-mediated activation of mTORC1. Human islets (100) were treated for 4 days as indicated. Samples were processed for Western blotting and quantitated by densitometry. Data are the means ± SEM of n = 3 experiments. (C) Human islets (50) were cultured for 4 days in cCMRL containing 5.6 mM glucose, 25 mM glucose + 500 μM FFAs, or 25 mM glucose + 500 μM FFAs + 25 nM rapamycin. Frozen sections (10 μm) of islets were processed for immunostaining [insulin (green), nuclei (blue), and Nile red (red)]. Images were rescaled using Adobe Photoshop software. (D) The bar graph shows the average intensities of Nile red for lipid accumulation and DyLight 647 for insulin, respectively, of 15–20 islet sections per condition from three different experiments is shown.](/cms/asset/b074b968-007a-4b20-9fdf-d4a97e2dcb16/kisl_a_10922720_f0009.gif)
Figure 10.(A) Absence of human islet cell growth under nutrient overload. Phase contrast images of human islets (40) before and after the 4-day incubation period under various conditions as indicated were acquired using a 20× objective. The diameter of each islet was computed by converting pixels to μm using the Metamorph image analysis software. The white and the black bars indicate the sizes of the islets before and after the treatment, respectively. Data are the means ± SEM of n = 3 experiments. (B) Insulin release into the culture medium under nutrient overload. Human islets (30) were cultured in 2 ml cCMRL containing 10 mM glucose or 240 μM FFAs alone or in combination of the two nutrients. Cultured medium (50 μl) was removed at the indicated times and the amount of insulin released into the culture medium was determined by radioimmunoassay. Data are the means ± SEM of n = 3 experiments. (C) Insulin content in human islets after 4-day treatment under nutrient overload. After treatment, islets were washed and resuspended in 500 μl of 0.1% BSA in PBS, followed by sonication using a microtip probe. Insulin content was determined by radioimmunoassay. Data are the means ± SEM of n = 3 experiments with triplicate samples in each experiment. (D) Pro-insulin levels in islets after 4-day treatment under nutrient overload. After treatment, islets were washed and resuspended in 200 μl of 0.1% BSA in PBS, followed by sonication using a microtip probe. Pro-insulin levels were determined as described in the Methods section. Data are the means ± SEM of n = 3 experiments.
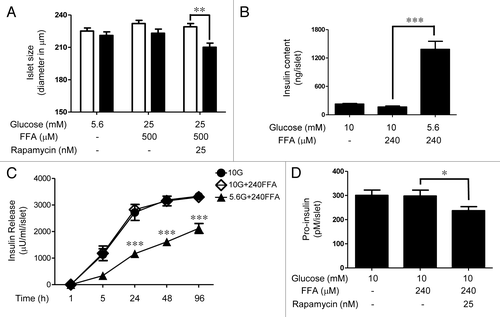