Figures & data
Figure 1. A model of IF evolution from an ancestral lamin progenitor. Intron positions (triangles, numbered with Roman numerals) are shown with respect to protein structure. Large boxes represent coiled-coil segments. The oval represents the lamin Ig-domain. Archetypal intron positions are labeled in red, introns gained in the course of cytoplasmic IF evolution are in blue. Deletion of the region containing the NLS is indicated by gray lines, the resulting new intron is marked by a star. Invertebrate IF proteins lack the last CaaX-encoding exon. Coil 1b in vertebrate IF proteins is shorter by six heptads. The deletion of the six heptads can be explained by generation of a new splice acceptor site of intron I. The heptads, which become deleted, are marked by dashed lines in coil 1b of the invertebrate IF. The tail domains of vertebrate IFs do not show resemblance to their invertebrate progenitors, marked by a hatched line. NLS, nuclear localization signal; CaaX, isoprenylation motif; CDK1, CDK1 phosphorylation site.
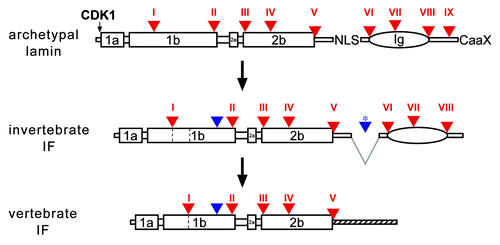
Figure 2. Phylogenetic tree of the metazoan lineage. The tree was modified from reference Citation69. The time scale was taken from molecular clock results of reference Citation70. It is consistent with paleontological findings. Choanoflagellates were taken as the outgroup of metazoans for this phylogenetic tree. The green triangle indicates the acquisition of an intron restricted to the protostome lineage; yellow triangles indicate the acquisition of two introns restricted to nematodes; the blue triangle indicates acquisition of an intron restricted to arthropods; the open triangle represents the acquisition of an intron present in all vertebrate lamin genes.
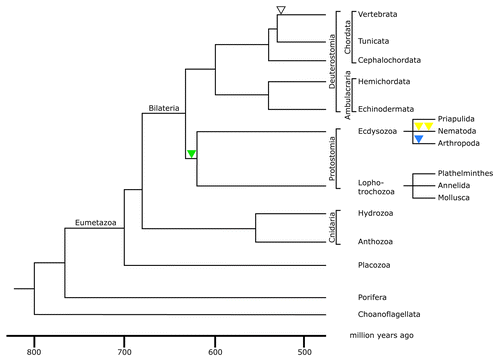
Figure 3. The intron positions in lamin genes are conserved between the most basal metazoans and vertebrates. Alignment of predicted lamin protein sequences of the sponge Amphimedon queenslandica, the placozoan Trichoplax adhaerens, two cnidarians, Hydra magnipapillata, a hydrozoan, and Nematostella vectensis, an anthozoan, and human lamin B1 (Homo B1) was performed with the MultAlin softwareCitation75 using the Blosum62 matrix.Citation76 The sequence for the sponge was manually extracted from the Amphimedon genome project.Citation74 The Trichoplax, Hydra and Nematostella lamin sequences were taken from published data.Citation77 Trichoplax intron positions were re-inspected and a putative CaaX motif-encoding exon was identified and added to the Trichoplax sequence. The gene structure of human lamin B1 was taken from.Citation78 The position of all nine introns present in the lamin genes of the four basal metazoans is conserved in the human lamin. These intron positions are marked by white letters on red background and are numbered with roman numbers I to IX. Introns, which have no match in one of the other four lamin genes, are marked white on black. Where the exon/intron boundary is between two codons, the two adjacent amino acid residues are marked. In the other two cases the exon / intron boundary is between the second and third base of the codon of the marked amino acid residue. The short CaaX motif-encoding exon is heterogeneous in size. Consequently, intron positions of intron IX appear out of register in this alignment.
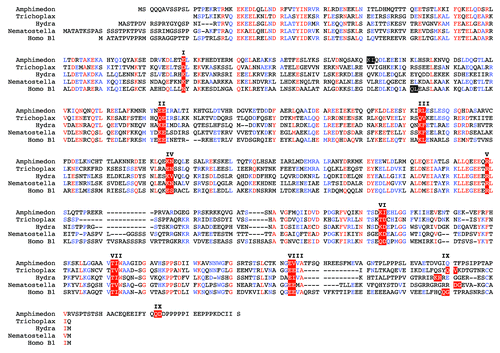
Figure 4. Lamin gene evolution in insects. (A) Phylogenetic tree of insects. The tree and the time scale were taken from reference Citation81. Loss of phylogenetically relevant introns (archetypal in red, arthropod-specific in blue) is indicated below the lines of the tree. For the dipterans only the branches of the Cuculimorpha and the Ephydroidea are shown. (B) Intron patterns of insect lamins. Positions of archetypal introns (red triangles) are shown with respect to lamin protein structure in the top row. Large boxes represent coiled-coil segments. The oval represents the lamin Ig-domain. Head and tail domains are represented by gray bars. Color coding of triangles, green, intron restricted to the protostome lineage; blue, introns restricted to arthropods; gray, introns restricted to hymenopterans (Apis and Nasonia); black, introns unique to individual species. The split blue triangles indicate a deletion of seven heptads in coil 2b of the Anopheles and Aedes lamins.
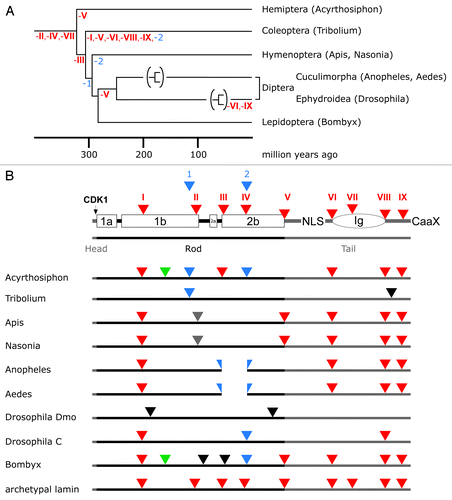
Figure 5. Lamin gene evolution in nematodes. Labeling of lamin structure and intron color code is as in . Yellow triangles: introns restricted to nematodes.
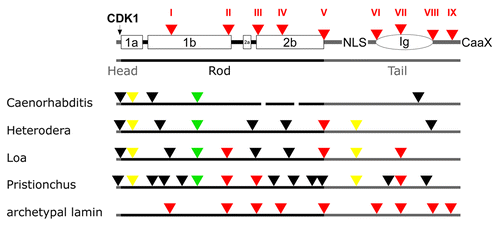
Figure 6. Lamin gene evolution in deuterostomes. Phylogenetic tree (A) and intron patterns (B) of deuterostome lamins. The tree and the time scale were taken from reference Citation69. Labeling in (B) is as described in the legend to . The loss of the Ig domain in the tunicates can be explained by deletion of three exons.
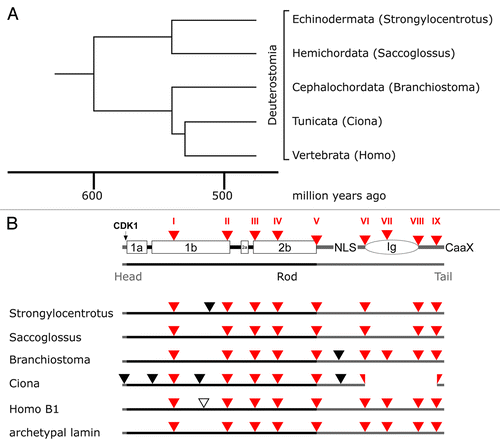
Figure 7. Protein structure of vertebrate lamins. (A) A model for the evolution of lamin A from a B-type progenitor. The lamin A specific domain is shown as a blue bar. The ZMPSTE24 cleavage site is indicated by an upward pointing blue arrow. (B) Lamin C, an alternative splice product of the LMNA gene. Lamin C is identical to lamin A up to residue 566 followed by six unique amino acid residues encoded by the 5′-end of intron IX (marked in black). (C) Protein structure of the two mammalian germline-specific lamins, C2 and B3. In lamin C2 the head, coil 1a, and part of coil 1b of lamin C are replaced by a short stretch of amino acid residues (green bar), which serve as myristoylation site. In lamin B3 the head, coil 1a and 1b of lamin B2 are replaced by a new non-α-helical head. The length of the new head differs between rat, mouse, and human between 50 to 81 residues. Intron positions (triangle, and Roman numerals) are shown with respect to protein structure. Large boxes represent coiled-coil segments. The oval represents the lamin Ig-domain. Archetypal intron positions in red, newly gained intron positions in lamin A in red/blue. The vertebrate-specific intron position is marked by an open triangle. NLS, nuclear localization signal; CaaX, isoprenylation motif; CDK1, CDK1 phosphorylation site.
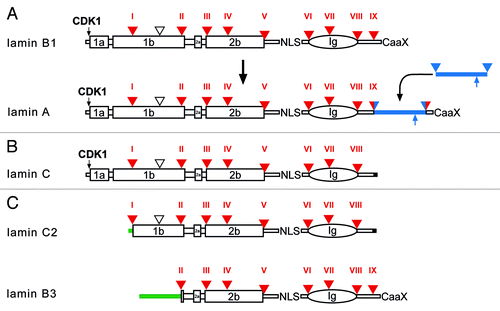