Figures & data
Figure 1. Dynamics of H3.3 and histone modifications during reprogramming in SCNT embryos. (A) and (B) Dynamics of H3K4me3 in the nuclei of SCNT embryos. H3K4me3 levels in the donor nuclei increase from 5 h to 20 h after activation in SCNT embryos (Arrows indicate the transplanted cumulus nuclei). Data are represented as H3K4me3 fluorescence intensities relative to DNA at various time points, with error representing SD (n ≥ 5). (C) and (D) Dynamics of H3K27me3 in the nuclei of H3.3B-HA cumulus nuclear transfer embryos. The H3K27me3 is gradually lost in the donor nuclei of SCNT embryos after activation (Arrows indicate the transplanted cumulus nuclei). Data are represented as H3K27me3 fluorescence intensities relative to DNA at various time points, with error representing SD (n ≥ 5). Bar scale: 20 µm.
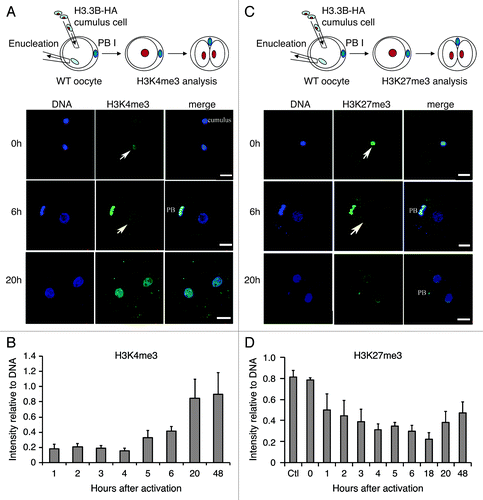
Figure 2. Dynamics of H3.3 and histone modifications during reprogramming in H3.3KD SCNT embryos. (A-C) Knockdown of maternal H3.3 inhibited the removal of donor H3.3B-HA, but did not alter the gain of the H3K4me3 in the donor nuclei of cumulus SCNT embryos. PB, the first polar body from H3.3B-HA wild-type oocytes. Data are represented as H3.3B-HA and H3K4me3 fluorescence intensities relative to DNA at various time points, with error representing SD (n ≥ 5). (D-F) Knockdown of maternal H3.3 in the oocytes impairs the loss of both donor cell-derived H3.3B-HA and H3K27me3 in SCNT embryos. Cumulus, H3.3B-HA wild-type cumulus cells. Data are represented as H3.3B-HA and H3K27me3 fluorescence intensities relative to DNA at various time points, with error representing SD (n ≥ 5). Bar scale: 20 µm.
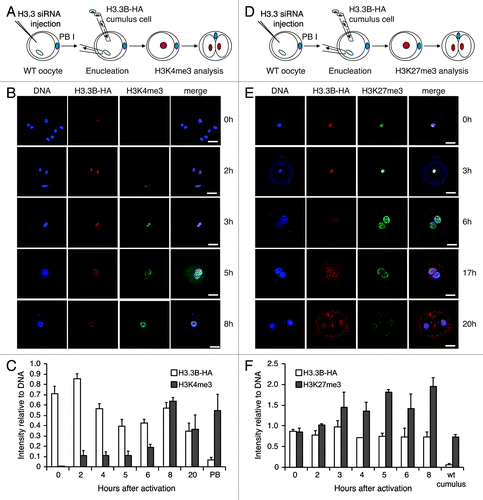
Figure 3. Dynamics of H3.3 and H3K9me3 during reprogramming in H3.3KD SCNT embryos. (A) Dynamics of donor cell-derived H3.3B-HA and H3K9me3 in the nuclei of H3.3B-HA cumulus SCNT embryos. The loss of donor cell-derived H3K9me3 is concurrent with the loss of donor cell-derived H3.3B-HA. (B) Dynamics of the donor cell-derived H3.3B-HA and H3K9me3 in the nuclei of H3.3KD SCNT embryos. The loss of donor cell-derived H3K9me3 is not altered when maternal H3.3 is knocked down. Cumulus: H3.3B-HA wild-type cumulus cells. Data are represented as H3.3B-HA and H3K9me3 fluorescence intensities relative to DNA at various time points, with error representing SD (n ≥ 5).
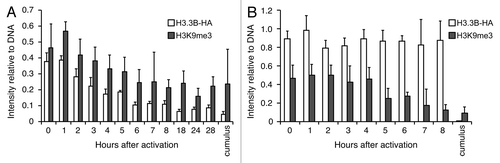
Figure 4. A working model of H3.3 replacement in remodeling the chromatin for gene reactivation. A silenced pluripotency gene is marked with repressive modifications on histone H3 (e.g., H3K27me3, red triangles) on regulatory regions in somatic cells. De novo synthesized H3.3 protein (shown in green and purple) replaces histone H3 carrying modifications associated with repression during the reprogramming process. Consequently, this replacement alters nucleosomal structure and enriches H3.3 in these loci. Through H3.3 replacement, we speculate that the repressive chromatin is remodeled and transformed to a permissive state, which may be accessible to other regulatory factors for gene reactivation to establish a chromatin landscape poised for activation (e.g., H3K4me1, H3K4me3, H3K27ac, green circles) in the pluripotent state.
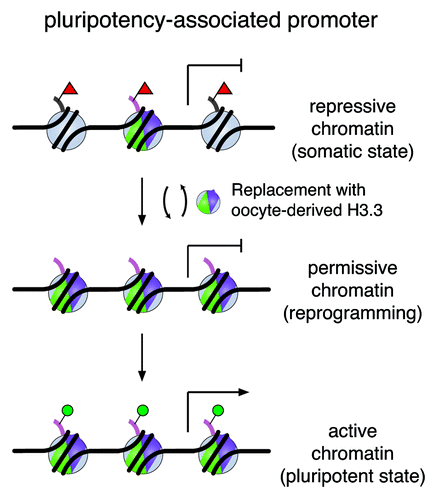