Figures & data
Figure 1. Structural basis of canonical ciliary subcompartments. (A) Left; representation of a canonical C. elegans amphid channel sensory neuronal cilium. Ciliary microtubules (MT) extend from a fully degenerated basal body at the ciliary base, with microtubules flaring proximally into the periciliary membrane compartment (PCMC). The PCMC is a swelling of the distal dendrite tip, bounded at its proximal side by a belt-like adherens junction between the sensory neuron and the enveloping sheath support cell (not shown). An ~0.8 μm long ciliary transition zone emerges from the ciliary base, consisting of nine closely tethered doublet microtubules (via an internal apical ring (AR); see cross section), with each doublet connected to the ciliary membrane via Y-links. Also present are inner singlet microtubules (gray) extending to varying degrees along the axoneme. The TZ is followed by an ~4 μm long middle segment consisting of 9 doublet microtubules, after which the B-tubule of each doublet terminates to establish the nine singlet A-tubule arrangement of the ~3 μm long distal segment. Right; representation of a canonical human primary cilium, showing the basal body (and associated distal (DA) and subdistal (sDA) appendages), transition zone and main axonemal compartments. Example also shows the emergence of the cilium from a ciliary pocket, which is an invagination of the periciliary membrane observed for some ciliary subtypes. (B) Schematic of the amphid sensillum—the largest sensory organ in C. elegans—in the nematode nose region. Ten ciliary axonemes occupy the environmentally exposed channel (only 3 shown for illustration purposes), created by ciliary axonemes punching through the surrounding sheath (glial) cell. Belt-like adherens junctions (AJ) at the base of each periciliary membrane compartment (PCMC) seal the channel.
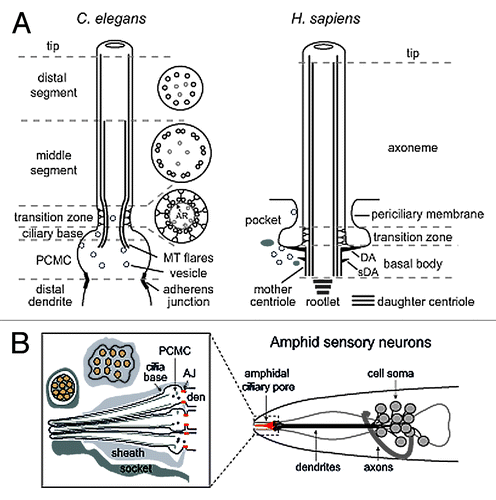
Figure 2.C. elegans proteins with distinct roles in specific subciliary compartments. (A) Proteins known to be enriched at the periciliary membrane compartment (PCMC) and ciliary base region. These include multiple proteins involved in secretion (orange) and endocytosis (yellow), as well as intraflagellar transport (IFT; green). Other enriched proteins are shown in red. The periciliary membrane is thought to be compartmentalised from the ciliary membrane via a lateral diffusion barrier at the transition zone. Although unproven, the belt-like adherens junction may serve to compartmentalise the PCMC from more proximal dendritic membrane. OSTA-1; organic solute transporter anion-1, DYN-1; dynamin, AP2; clathrin adaptor 2 complex, CHC; clathrin heavy chain, STAM-1; endosomal signal transducing adaptor molecule, HGRS-1; endosomal hepatocyte growth factor-regulated tyrosine kinase substrate, BBS; Bardet-Biedl syndrome complex (BBSome), A; IFT subcomplex A, B; IFT subcomplex B, HYLS-1; Hydrolethalus syndrome protein 1, RPI-2; retinitis pigmentosa gene 2, PC1/2; Polycystin 1/2, Tram; translocating chain-associated membrane protein (translocon), GPCRs; G-protein coupled receptors, OA-; organic anion (B) At least 9 proteins linked to ciliopathies are specifically localized in the transition zone (TZ). These are MKS-1/BBS13, MKS-3/TMEM67, MKS-5/RPGRIP1L, MKS-6/Cc2d2a, MKSR-1/B9D1, MKSR-2/B9D2, JBTS-14/TMEM-237, NPHP-1/NPHP1 and NPHP-4/NPHP4. Genetic analyses in C. elegans indicate these proteins form distinct, but functionally associated, modules (‘MKS’ and ‘NPHP’), possibly linked via MKS-5. Together, these modules establish TZ connections with the ciliary membrane by regulating the assembly of Y-links. Also, these modules establish the transition zone as a ciliary gate, regulating lateral diffusion of membrane proteins into and out of the cilium (e.g., as shown for RPI-2). MS; middle segment, PCMC; periciliary membrane compartment. (C) Middle segment (MS) localization of palmitoylated ARL-13/ARL13B, NPHP-2/Inversin and various cyclic gated nucleotide (CNG) channels. Also shown is an anterograde IFT assembly, which in amphid channel ciliary middle segments is powered by the coordinated (and redundant) activities of two kinesin-2 motors, heterotrimeric kinesin-II (kin-II) and homodimeric OSM-3/KIF17. Lateral diffusion barriers flanking the compartment prevent ARL-13 from diffusing out of the compartment into the transition zone (TZ) and distal segment (DS) compartments. ARL-13 can also associate with IFT trains as potential cargo. (D) Assembly of distal segments (DS) via OSM-3 powered anterograde IFT. At tip of middle segment (MS), kinesin-II turns around and is trafficked back to the ciliary base via IFT-dynein (cDHC1b) powered retrograde IFT, leaving OSM-3 to drive anterograde transport of the IFT particle (A and B) and associated cargo to the ciliary tip. At tip, OSM-3 becomes retrograde IFT cargo.
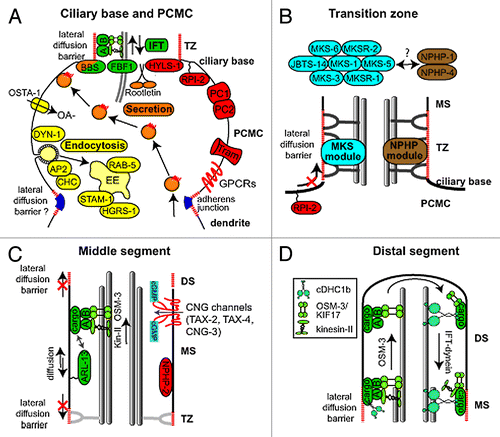