Figures & data
Figure 1. tRF(Gln) expression is decreased in conditions of slowed cell proliferation. (A) HeLa cells were grown in DMEM lacking L-glutamine for the indicated times, and tRF(Gln) levels assayed by northern blot. (B) HeLa cells were grown in DMEM lacking serum for the indicated times, and tRF(Gln) levels assayed by northern blot. Quantifications of tRF(Gln) levels, normalized to the non-coding RNA U6, are shown adjacent to the blots. Error bars show standard deviations, and p values were calculated using Student's t-test (two-tailed).
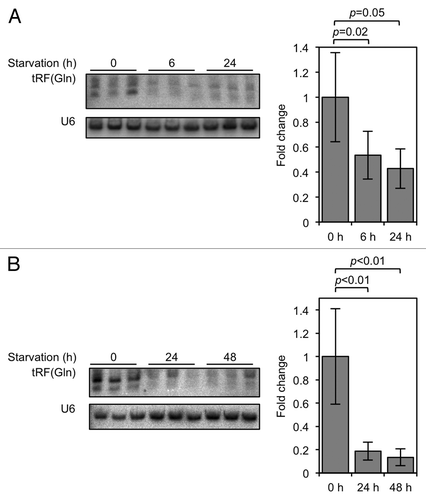
Figure 2. tRF(Gln) does not cause siRNA-like cleavage of a reporter construct. (A) Experimental design. HeLa cells were transfected with a reporter plasmid containing three perfectly complementary target sites to tRF(Gln). When an dsRNA mimic of tRF(Gln) is co-transfected, the reporter is cleaved leading to a reduction in Renilla luciferase expression. This does not happen with a control reporter containing a mismatch in the seed sequence and at the cleavage site (represented as a filled circle). (B) The cleavage reporter construct was co-transfected with either a scrambled dsRNA (Scr), or an dsRNA mimic of tRF(Gln) (Gln). (C) Cleavage reporter constructs containing sites perfectly complementary to either the miRNA let-7 or tRF(Gln) were co-transfected into cells without cotransfection of dsRNA, in order to assay the activity of endogenous molecules. Separately, control reporters for let-7 and tRF(Gln) were transfected; these control reporters contain two mismatches in their respective target sites to render them insensitive to repression (gray bars). Results shown are the mean of three independent experiments, and error bars represent standard deviations.
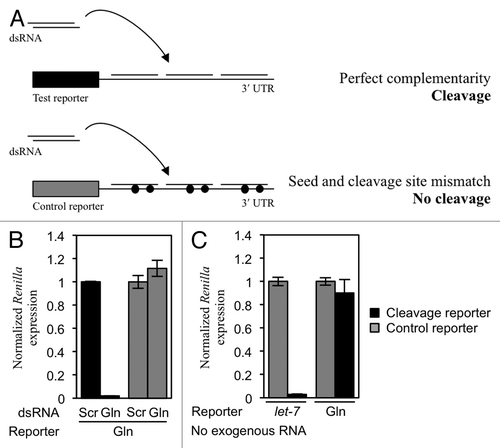
Figure 3. tRF(Gln) does not repress translation of a reporter construct with bulged target sites in the 3′ UTR. (A) Experimental design. HeLa cells were transfected with a reporter plasmid containing six bulged tRF(Gln) target sites, which is predicted to be sensitive to miRNA-like translational repression. In control experiments, a methylated antisense RNA was co-transfected to prevent small RNAs from binding the target sites. The bulge is represented as a filled circle. (B) The translation reporter was co-transfected into HeLa cells with either a scrambled dsRNA (Scr) or a mimic of tRF(Gln) (Gln), and also the indicated antisense RNA. (C) To assay the activity of endogenous molecules, the indicated amounts of a reporter plasmid containing six bulged miRNA-like sites for tRF(Gln) was transfected into HeLa cells (black bars). Renilla expression was assayed separately in the presence of a co-transfected scrambled antisense RNA, and with an antisense RNA containing the reverse complement of tRF(Gln). The relative Renilla expression was calculated as the normalized expression obtained with the scrambled antisense RNA, divided by that obtained with the Gln antisense RNA (see Materials and Methods), such that a value of 1 indicates no tRF(Gln)-dependent repression (dashed line). The same experiment was performed with a control reporter containing a seed mismatch (gray bars). Results shown are the mean of three independent experiments, and error bars represent standard deviations.
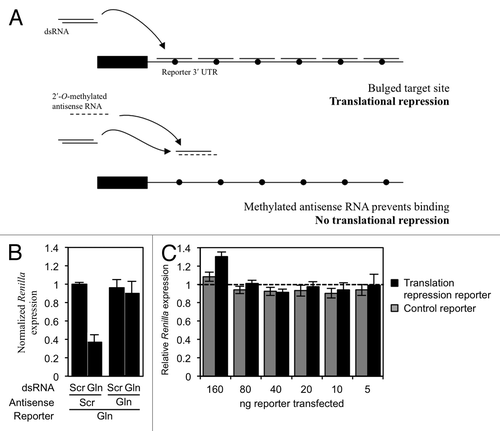
Figure 4. Gln19 can repress translation of Renilla and firefly luciferases that do not contain complementary target sites. (A) HeLa cells were transfected with a reporter plasmid, and 19-base single-stranded RNA molecules containing either the tRF(Gln) sequence or a scrambled sequence. The firefly gene contained no target sites, and the Renilla gene contained either three perfect Gln target sites or mutated control versions of the same, as indicated. The expression of Renilla and firefly luciferases were measured separately. (B) In vitro translation reactions were performed with the indicated concentrations of Gln19 or Gln19(Scr) present in the reaction. The messenger did not contain any Gln19 target sites. Results shown are the mean of three independent experiments, and error bars represent standard deviations.
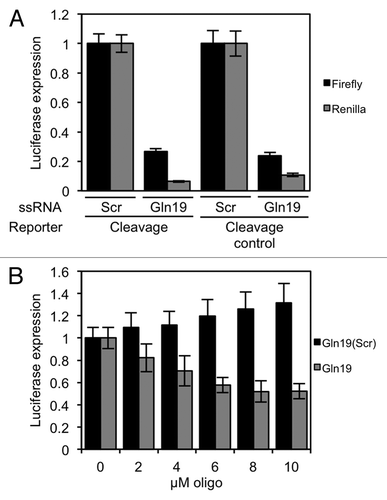
Figure 5. Translation repression requires a 'GG' dinucleotide that is conserved in tRFs. (A) In vitro translation reactions were performed in the presence of the indicated 19-mer oligonucleotides. (B) Diagrams of the Gln19-derived oligonucleotides that were used to find sequence requirements for translation repression. The mutated bases are highlighted. (C) In vitro translation reactions were performed with the Gln19-derived oligonucleotides. Gln19(mGG) showed a severe defect in its ability to repress translation. (D and E) In vitro translation reactions were performed with (D) Lys19 and (E) Val19, and their corresponding mutants. In both cases, translation repression required a 3′ “GG” dinucleotide. In all reactions, the concentrations of oligonucleotide used were 0, 2.5, 5 and 10 µM. Results shown are the mean of three independent experiments, and error bars represent standard deviations.
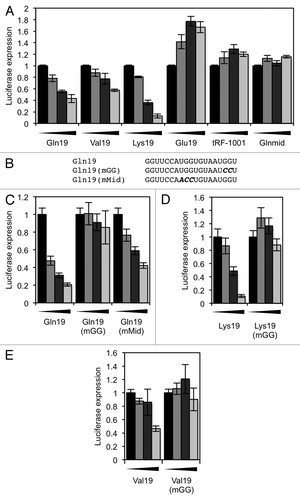
Figure 6. Translation assays using a non-tRNA-derived sequence. (A) A 19-base oligonucleotide (Art19), containing a 3′ “GG,” was designed with only the loop sequence (gray box) derived from tRNA. Related oligos with the 3′ “GG” mutated, and an oligo containing the 3′ “GG” but designed to have less secondary structure, were also designed. (B) The oligonucleotides shown in (A) were used in an in vitro translation reaction. (C) An oligonucleotide was designed that is derived from Art19, but with the loop sequence changed to a non-tRNA-derived sequence. (D) The oligonucleotides shown in (C) were used in an in vitro translation reaction. In all reactions, the concentrations of oligonucleotide used were 0, 2.5, 5 and 10 µM. Results shown are the mean of three independent experiments, and error bars represent standard deviations.
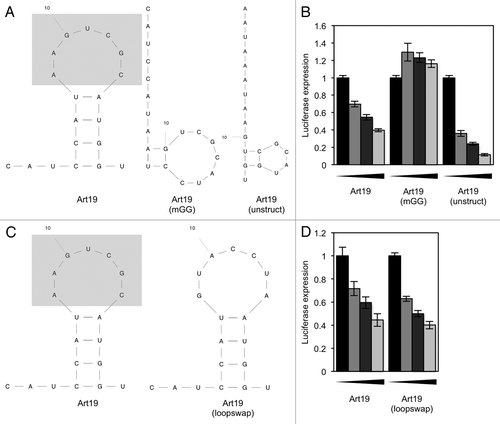
Figure 7. Translation repression by 5′ tRFs does not occur by mRNA or tRNA degradation, and does not involve the mRNA cap structure or poly(A) tail. (A) tRFs do not trigger mRNA deadenylation or degradation. An in vitro translation reaction was performed in the presence of 10 µM Gln19 or Gln19(mGG) and total and polyadenylated Renilla luciferase mRNA quantified by qPCR, using random hexamers or dT20 reverse transcription primers respectively. (B) tRFs do not change tRNA abundance. HeLa cells were transfected with either Gln19 or Gln19(Scr), and after 24 h, tRNA(Gln) quantified using qPCR are shown normalized to 5S abundance. (C) tRFs do not trigger a tRNA charging defect. An in vitro translation reaction was performed in the presence of either buffer, 10 µM Gln19 or 10 µM Gln19(mGG). The amounts of charged tRNA(Gln) and uncharged tRNA(Gln) in each sample were resolved using an acidic gel. (D) mRNAs were synthesized in vitro that contain either a cap analog plus a poly(A) tail, a cap analog and no poly(A) tail, or a non-functional cap analog and no poly(A) tail. These mRNAs were used in an in vitro translation reaction in the presence of Gln19. The concentrations of oligonucleotide used were 0, 2.5, 5 and 10 µM. Results shown are the mean of three independent experiments, and error bars represent standard deviations. Cap: m7G(5′)ppp(5′)G. ACap: A(5′)ppp(5′)G. p(A): poly(A) tail.
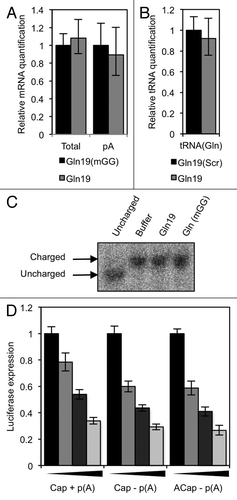
Figure 8. A proportion of cellular tRFs associate with polysomes. Cell lysate from HeLa cells was separated on a 10–50% (w/v) sucrose gradient to resolve polysomes. Cells were either treated with 50 µg/mL cycloheximide (7 min) or the chain terminator puromycin (200 µg/mL, 15 min) before lysis. The absorbance profile at 260 nm is shown. tRF(Gln) was detected using northern blotting, and quantifications of the level in each fraction are shown below the blots.
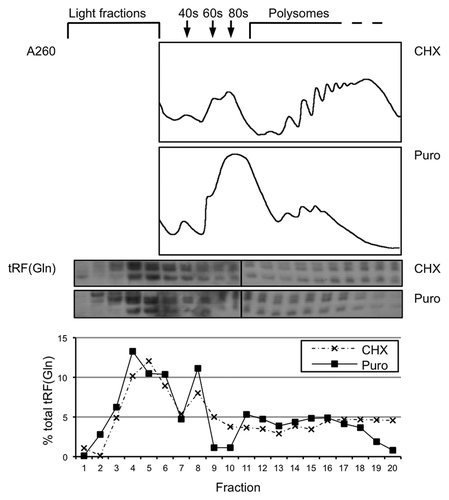