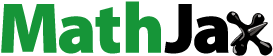
Abstract
The antagonistic effects of kinetin and spermine on stress imposed by seawater on leaf area, pigment, Hill reaction, 14CO2 fixation and chloroplast ultrastructure of wheat flag leaf were investigated. Irrigation of wheat plants by seawater at 25% caused marked decrease in leaf area, pigment content, Hill activity and photosynthetic efficiency of wheat flag leaf at ear emergence. Grain priming with kinetin, spermine or their interaction alleviated the adverse effect of seawater stress by stimulating leaf area expansion, pigment production as well as photosynthetic activity. From transmission electron microscopy micrographs, a continuous “end-to-end” distribution of regular (oval or elliptical) chloroplasts around the cell's periphery was observed in flag leaf mesophyll cells of control wheat plants. Conversely for seawater-stressed plants, the irregular spherical chloroplasts appeared “bulbous” and discrete, the cells also displayed extensive but thin peripheral cytoplasmic regions devoid of chloroplasts. Grain presoaking in 0.1 mM kinetin caused the chloroplast of stressed wheat plants to be more regular, with organized membrane system, large starch grains and projections in the form of tails. Furthermore, ultrastructure analysis cleared that grain priming with spermine, either alone or in combination with kinetin, caused the chloroplast in flag leaf mesophyll cells of stressed wheat plants to be more regular in shape with more starch grains. The changes in pigment content and photosynthetic activity of flag leaf appeared to depend mainly on chloroplast ultrastructure and its numbers, showing a positive correlation between chloroplasts number and pigment content.
1 Introduction
Salt stress is a global problem in crop production, and secondary salinization in soils of protected cultivation has become one of major factors adversely affecting horticultural crops. Salt stress affects every aspect of physiology in crops, as a result virtually reduces their productivity and adversely affects their product quality [Citation1].
Photosynthesis is one of the primary processes most affected by abiotic stresses [Citation2]. Additionally, plant pigments are known to serve a variety of purposes, and are thus critical to the function and health of plants, though the relative concentrations of these pigments can vary significantly depending not only on the species but also on the surrounding environmental factors [Citation3]. It is well documented that salt stress exerts its deleterious effects on plant growth and development through its induction to the reduction in Chl a, Chl b, carotenoids and total pigments [Citation4]. Aldesuquy and Gaber [Citation5] found that seawater at 25% induced massive reduction in soluble, insoluble and total photosynthates as well as ratio of soluble/insoluble photosynthates during growth and development of Vicia faba plants. Furthermore, reduction in the pigment content by various stress conditions was previously recorded [Citation6]. Clear reduction in Chl a, Chl b and carotenoids concentrations was observed in pineapple explants treated with seawater [Citation7]. High salinity induced a decrease in photosynthetic efficiency is often associated with inhibition of photosystem II (PSII) [Citation8,Citation9]. PSII has been considered the main site of salt stress-mediated damage to electron transport processes [Citation10], yet the damage site of PSII seems to vary with the severity and duration of stress [Citation11] and plant species [Citation12].
Electron microscopy showed that in salt-stressed plants, the structure of the chloroplasts becomes disorganized, the number and size of plastoglobuli increased and their starch content decreased [Citation13]. Similarly, under saline conditions, the chloroplasts of Aster tripolium plants were partially horseshoe-shaped. Their thylakoid membranes showed dilations, the spaces between the membranes looked swollen and undilated thylakoid areas were developed. In particular, the number of grana stacks was considerably reduced [Citation14].
Plant growth regulators (PGRs) have been found to play a key role in the integration of the responses expressed by plants under stress conditions [Citation15]. Many reports have indicated that stress tolerance of plants is correlated with their capacity to enhance the synthesis of polyamines upon encountering the stress [Citation16].
Salt stress, especially at the higher NaCl concentration, was found to induce a substantial decrease in leaf area and stomata conductance; chlorophyll content and δ-aminolevulinic acid dehydratase (ALA-D) activity were also affected, resulting in the lower net photosynthetic rate and dry matter production. Meanwhile, kinetin was found to reduce appreciably the adverse effects of salinity, besides favorably modulating antioxidant enzyme activities and alleviating oxidative stress in the test plants, to result in a higher yield as compared to the untreated stressed plants. Overall, the results indicate an optimization of antioxidant defense mechanisms and physiological processes by kinetin and a significant role of exogenous phytohormones in conferring salt tolerance in Nigella sativa L. [Citation17,Citation18]. Shu et al. [Citation1] concluded that Spermidine can alleviate salt-induced damage on cucumber seedlings by regulating the levels of endogenous polyamines, which was associated with an improvement in the photochemical efficiency of PSII of the salt stressed plants.
The objective of this study was to determine the effects of exogenous kinetin, spermine or their interaction on leaf area expansion, pigment content, photosynthetic activity and chloroplast ultrastructure of flag leaf as well as their role in alleviating the damage induced by seawater stress.
2 Materials and methods
2.1 Plant material and growth conditions
For soaking experiment, a homogenous lot of Triticum aestivum L. var. Sakha 93 grains were selected. The grains were surface sterilized by soaking in 0.01 M HgCl2 solution for 3 min, then washed thoroughly with distilled water. The sterilized grains were divided into four sets. Grains of the 1st set were soaked in distilled water to serve as control, while those of the 2nd, 3rd or 4th set were soaked in 0.1 mM kinetin, 0.3 mM spermine or 0.1 mM kinetin + 0.3 mM spermine; respectively, each for about 12 h. After soaking, thoroughly washed grains were drilled in 15th November 2011 in plastic pots (20 cm in diameter) filled with 5.5 kg soil (clay/sand 2/1, v/v), where fifteen grains were sown in each pot. The pots were then kept in a greenhouse at Botany Department, Faculty of Science, Mansoura University, Egypt. The plants were subjected to natural day/night conditions (minimum/maximum air temperature and relative humidity were 15/25 °C and 35/45%; respectively) at mid-day during the experimental period. The plants in all sets were irrigated to field capacity by tap water. After two weeks from sowing, thinning was started so that five uniform seedlings were left in each pot for the subsequent studies. The plants of each set were sub-divided into two groups. The 1st group in each set was still irrigated with normal tap water serving as control, whereas the 2nd one was irrigated with 25% seawater (volume/volume). The resulting eight treatments were marked as following: 1. Control (Cont.), 2. Seawater (SW), 3. Control kinetin (Cont. K), 4. Seawater + kinetin (SW + K), 5. Control spermine (Cont. Spm), 6. Seawater + Spermine (SW + Spm), 7. Control kinetin + spermine (Cont. K + Spm), 8. Seawater + kinetin + spermine (SW + K + Spm). Irrigation with seawater was applied after 30 days from sowing with a periodical soil washing (each two weeks) with tap water. The chemical analyses of the employed seawater, collected from the Mediterranean Sea, revealed that it contains Cl−, 21.6 kg m−3; Na+, 11.1 kg m−3;
, 2.85 kg m−3; K+, 0.49 kg m−3 and P3+ 16.6 μg dm−3. Its salinity was found to be 38.5 g kg−1; pH, 8.1 and electric conductivity (EC), 47 mmhos cm−1 [Citation19]. After thinning and at heading, the plants received 36 kg N ha−1 as urea and 25 kg P ha−1 as super-phosphate. Measurements were carried out at ear emergence (i.e. 65 d from planting). Samples from the flag leaf of the main shoot were taken as follow: ten replicates for measuring leaf area and triplicates for determining pigment, photosynthetic and Hill activities.
2.2 Leaf area
Leaf area was measured according to the equation followed by Quarrie and Jones [Citation20],
2.3 Estimation of photosynthetic pigments
Photosynthetic pigments (chlorophyll a, chlorophyll b and carotenoids) were determined according to the spectrophotometric method recommended by Arnon [Citation21].
2.4 Hill reaction assay
As described by Arnon [Citation21], 4 g of detached leaves were ground three times for 5 s at full speed in a chilled blender in 50 ml of 50 mM N-Tricine (N-Trishydroxyl methylglycine, pH 7.8), 0.3 M sucrose and 2 mM MgCl2. The resulting homogenate was filtered through four layers of nylon mesh. Chloroplast pellets were obtained by centrifugation at 2000 × g for 10 min. The pellets were re-suspended in 30 ml of 0.1 M NaCl and then centrifuged again at 5000 × g for 5 min. The resulting pellets were resuspended in 1 mM N-Tricine (pH 7.8), 10 mM NaCl and 10 mM MgCl2 and then kept at 0–4 °C until used for the analysis. Photosystem II activity, as indicated by the rate of 2, 6 dichlorophenolindophenol (2, 6-DCPIP) photo-reduction [Citation22] was monitored at 600 nm using spectronic 21 D spectrophotometer. The sample cuvette was illuminated by tungsten lamp (6000 lux) using head filter (CuSO4 solution). The photosystem II reaction mixture contained 200 mM Na phosphate (pH 6.7), 2 mM MgCl2 and 0.5 mM DCPIP. The chlorophyll concentration range for this experiment was 10 μg/ml of the reaction mixture (4 ml).
2.5 14CO2 uptake
As described by Aldesuquy and Gaber [Citation5] a definite fresh mass of flag leaf discs was introduced into the fixation apparatus (). An aqueous solution of 14C-sodium carbonate of known activity (3.7 MBQ cm−3) was pipetted into the apparatus followed by 0.2 ml of H2SO4 (10%). The evolved 14CO2 passed through the pores in the upper part of the inner container to the main apparatus where it could be photosynthesized by the green leaf discs. The experiment was carried out in natural sunlight at 10 a.m., when light intensity was about 3500 Lux. At the end of the fixation period (10 min), the leaf discs were quickly transferred to be killed and then extracted in a mortar by grinding. After being cooled, the insoluble fraction was separated from the soluble one by centrifugation at 2000 × g for 20 min. The soluble and insoluble fractions were made up to a known volume (35 ml) and then 2 ml of samples were pipetted into the plastic tubes of scintillator counter. The radioactivity of the green leaf discs was measured using a Packard Scintillation Counter model 526. The count per minute (cpm) obtained was then calculated according to the efficiency of the apparatus used. The radioactivity measured is directly proportional to the amount of CO2 fixed in soluble organic compounds, which was calculated as cpm/mg fresh mass of leaf.
2.6 Electron microscopy
The leaf tissues were processed for transmission electron microscopy (TEM) according to the method of Woods and Gay [Citation23]. The plant tissues were cut into 1 mm2 pieces and fixed in 2.5% glutaraldehyde in 0.1 M sodium cacodylate buffer for 24 h at room temperature, and then post-fixed in 1% osmium tetroxide in the same buffer. Leaf tissues were dehydrated in a graded ethanol series and infiltrated with Araldite. Sections showed silver-gray interference colors cut by Reichert ultramicrotme were collected on copper grids, stained with uranyl acetate and lead citrate [Citation24]. Sections were examined and photographed by using Jeol 1010 TEM.
2.7 Counting of chloroplasts in semi-thin sections
Number of chloroplasts in flag leaf mesophyll tissue of wheat plants was counted for each treatment in 1.0 μm thick sections cut parallel to the epidermis from Araldite-embedded material using ordinary light microscope. A computerized method was followed to count chloroplasts in semi thin sections. The image of 10× magnification power of computerized light microscope (equivalent to 40× using ordinary light microscope) was opened with “PAINT” program. The “Line” tool was then used to make standardization with the aid of the magnification bar already present in the image. This step aims at converting the measurement unit from pixels to microns, where it was found that 40 μm (length of the magnification bar) is equivalent to 57 pixels. Accordingly, the “Rectangle” tool was then used to draw a square of 71 × 71 pixels; since this area would be equivalent to 50 × 50 = 2500 square micron. Such squares were recommended to be drawn in a random fashion on the semi thin sections where the chloroplasts were counted in a replicate of ten square all of the same area. Finally, the mean number of chloroplasts was calculated for each treatment.
2.8 Data analysis
The main effect of factors (seawater stress and both used chemicals), and the interaction (seawater stress × chemicals) were evaluated by general linear model (two ways ANOVA) using SPSS program. A test for significant differences between means at P ≤ 0.05 was performed using least significant difference (LSD) test [Citation25].
3 Results
3.1 Changes in leaf area
As compared with control, 25% seawater caused a significant decrease in the area of flag leaf at ear emergence (). The inhibition of flag leaf area caused by seawater was alleviated when the grains were treated with kinetin, spermine or their interaction (). Kinetin appeared to be the most effective in inducing the maximum leaf area.
Fig. 2 Effect of grain presoaking in kinetin, spermine or their interaction on flag leaf area (cm2) of wheat plants (at heading stage) irrigated with seawater. Vertical bars represent LSD at P≤0.05. Note: cont. = control, SW = seawater, K= kinetin, SW + K = Seawater + kinetin, Spm = Spermine, SW + Spm = Seawater + Spermine, K + Spm = Kinetin + Spermine, SW + K + Spm = Seawater + Kinetin + Spermine.
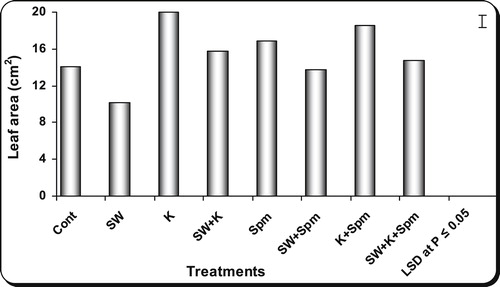
3.2 Changes in pigment content
In relation to the control plants, seawater stress induced drastic reduction (P ≤ 0.05) in pigment content (Chl a, Chl b, Chl (a + b), Chl (a/b) as well as carotenoids and total pigments) of wheat flag leaf at ear emergence ( and ). On the other hand, grain priming with kinetin, spermine or their interaction enhanced pigment production in stressed or unstressed wheat plants. In the majority of cases the application of kinetin + spermine appeared to be the most effective treatment in enhancing the pigment production in flag leaf of stressed and unstressed wheat plants ( and ).
Fig. 3 Effect of grain presoaking in kinetin, spermine or their interaction on Chl a, Chl b and Chl (a + b) contents (mg g−1 d wt) in flag leaf of wheat plants (at heading stage) irrigated with seawater. Vertical bars represent LSD at P ≤ 0.05. Note: cont. = control, SW = seawater, K = kinetin, SW + K = Seawater + kinetin, Spm = Spermine, SW + Spm = Seawater + Spermine, K + Spm = Kinetin + Spermine, SW + K + Spm = Seawater + Kinetin + Spermine.
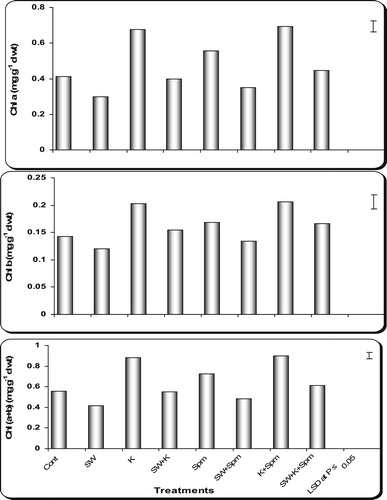
Fig. 4 Effect of grain presoaking in kinetin, spermine or their interaction on and Chl (a/b), carotenoids and total pigments (mg g−1 d wt) contents in flag leaf of wheat plants (at heading stage) irrigated with seawater. Vertical bars represent LSD at P ≤ 0.05. Note: cont. = control, SW = seawater, K = kinetin, SW + K = Seawater + kinetin, Spm = Spermine, SW + Spm = Seawater + Spermine, K + Spm = Kinetin + Spermine, SW + K + Spm = Seawater + Kinetin + Spermine.
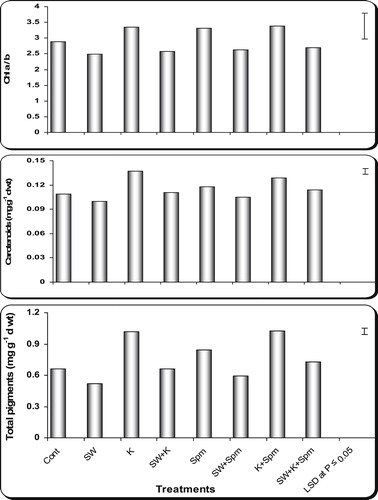
3.3 Changes in Hill reaction
Throughout the ear emergence, there was a significant decrease (P ≤ 0.05) in the Hill reaction rate within the flag leaf under the influence of 25% seawater (). Application of kinetin, spermine or their interaction appeared to mitigate the negative effect of seawater on Hill reaction activity ().
3.4 Changes in photosynthetic 14CO2
Seawater at 25% induced marked reduction (P ≤ 0.05) in soluble, insoluble and total photosynthates in flag leaf of wheat plants during ear emergence (). The magnitude of reduction in soluble, insoluble and total photosynthates was more pronounced. On the other hand, the interaction between seawater and kinetin or spermine induced noticeable increase in soluble, insoluble and total photosynthates. Kinetin was the most effective one in accelerating the accumulation of photosynthates in flag leaf at ear emergence.
3.5 Changes in chloroplast number and frequency in mesophyll cells of wheat flag leaf
Data in showed that irrigation of wheat plants with 25% seawater induced marked reduction (P ≤ 0.05) in chloroplast numbers and frequency in mesophyll cells of wheat flag leaves when compared with those of the control plants. On the other hand, application of kinetin, spermine or their interaction caused additional increase (P ≤ 0.05) in these values. The most pronounced effect was recorded for the interaction of kinetin and spermine treatment.
Fig. 5 Effect of grain presoaking in kinetin, spermine or their interaction on chloroplast number and frequency in mesophyll cells of flag leaf of wheat plants (at heading stage) irrigated with seawater. Vertical bars represent LSD at P ≤ 0.05. Note: cont. = control, SW = seawater, K = kinetin, SW + K = Seawater + kinetin, Spm = Spermine, SW + Spm = Seawater + Spermine, K + Spm = Kinetin + Spermine, SW + K + Spm = Seawater + Kinetin + Spermine.
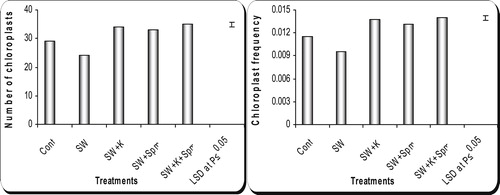
3.6 Changes in ultrastructure of mesophyll cells of wheat flag leaf
Transmission electron microscopic examination of wheat flag leaf revealed that the shape of chloroplast from mesophyll cells of control plants was comparatively regular (tended to be oval or elliptical to somewhat) when compared with that of seawater-stressed plants. Although the membrane system of grana and inter-granal lamellae was not clear enough, the chloroplast contained few starch grains and osmiophilic globules. In addition, big oleosomes were observed (A,B).
Fig. 6 TEM micrograph showing a mesophyll cell from flag leaf of control wheat plant. (A) The cell contains regular ellipsoidal-shaped chloroplasts (C). Note the nucleus (N), oleosomes (O), cell vacuole (V) and cell wall (W). Bar = 3 μm. (B) A magnified part of (A) showing ellipsoidal-shaped chloroplast (C) with an organized chloroplast envelope (e). Note starch grain (S) and osmiophilic globules (os). Bar = 2 μm.
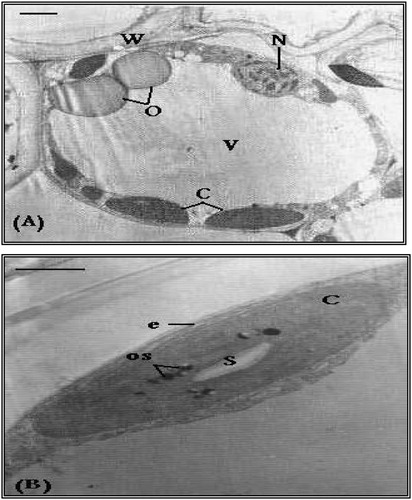
For the flag leaf of wheat plants irrigated with 25% seawater, it was apparent that the chloroplasts began to be less or more spherical with irregular shape. Moreover, disorganized membrane system was identified with swollen thylakoids in these chloroplasts. The starch grains were not only fewer in number, but also smaller in size than those recognized in control plants. More mitochondria and less oleosomes were also identified. Stressed cells also displayed extensive but thin peripheral cytoplasmic regions devoid of chloroplasts. Starch grains had approximately as the same size as those of control plants, but more in number. Projections in the form of tails were also present. The number of osmiophilic globules in chloroplasts was also increased (A).
Fig. 7 A. TEM micrograph of a mesophyll cell from flag leaf of wheat plants irrigated with 25% seawater, showing disorganized membrane system (short arrows) of chloroplasts. Note fragments of chloroplast (long arrows), small starch grain (S), mitochondria (M) and big nucleus (N). Bar = 2 μm. B. TEM micrograph of mesophyll cells from flag leaf of wheat plants treated with kinetin and irrigated with 25% seawater, showing somewhat organized membrane system (arrows) of chloroplast. Note the projection (P) originated from the chloroplast, and starch grains (S). Bar = 0.5 μm.
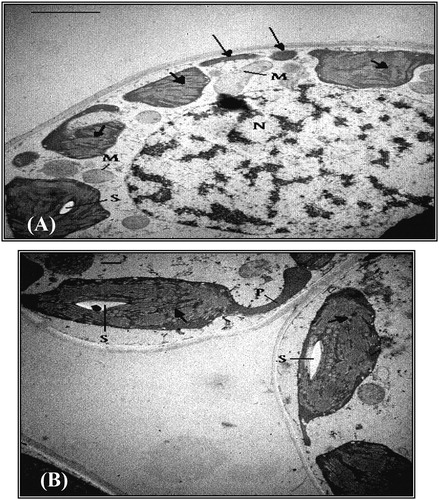
Interaction of kinetin and spermine to salt-stressed plants resulted in regular or oval chloroplasts containing many starch grains and osmiophilic globules. Furthermore, the chloroplast appeared to be divided (B and AB).
Fig. 8 A. TEM micrograph of a mesophyll cell from flag leaf of wheat plants treated with spermine and irrigated with 25% seawater, showing regular-shaped chloroplasts (C) with starch grains (S) and osmiophilic globules (os). Note chloroplast projection (P) and big nucleus (N). Bar = 2 μm. B. TEM micrograph of a mesophyll cell from flag leaf of wheat plants treated with kinetin + spermine and irrigated with 25% seawater, showing regular-shaped chloroplasts (C) packed inside the cell, with an organized membrane system. Note starch grains (S) and osmiophilic globules (os). Bar = 2 μm.
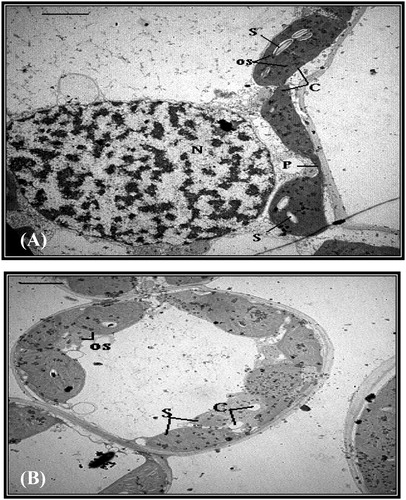
4 Discussion
In this contribution, the effects of exogenous kinetin, spermine or their interaction on leaf area, pigment content, photosynthetic activity and chloroplast ultrastructure of flag leaf in alleviating the damage induced by seawater stress were manifested. The general pattern of plant response to salt stress is a reduction in the rate of leaf surface expansion, followed by a cessation of expansion as the stress intensifies [Citation26]. The reduction of cell expansion due to salt stress may result from the decrease in cell wall extensibility [Citation27]. For that, it is assumed that salt stress could reduce cell extension by lowering the apoplastic acidification through its osmotic stress [Citation28]. Furthermore, it is demonstrated that both cell number and cell area are significantly reduced below a threshold of soil water content [Citation29].
Under saline conditions, plant growth is usually reduced by reducing the rate of leaf elongation, enlargement and the division of the cells in these leaves [Citation30]. On the other hand, it is proposed that due to abiotic stress originating from salinity, the plant tries to cope with the situation by decreasing its leaf area and hence allows the conservation of energy [Citation31]. The inhibition of leaf area in response to seawater irrigation was alleviated when the grains were soaked in kinetin. This recovery may result from the role of kinetin in stimulating the rate of movement of nutrients and hormones from the plant root toward the developing leaves, and thus accelerating the rate of leaf expansion [Citation5,Citation32]. The potentiality of kinetin, as a priming agent, to mitigate the adverse effects of seawater irrigation on morphological features of wheat plants may be also due to the ability of kinetin to enhance the accumulation and overproduction of polyamines (PAs). Matching this speculation, Iqbal and Ashraf [Citation33] assumed that presoaking of wheat grains in kinetin under salt stress could reverse growth inhibition mainly by increasing PAs titers (especially Spm) in leaves of the stressed plants.
Table 1 Effect of grain presoaking in kinetin, spermine or their interaction on the Hill reaction by chloroplasts (H2O → DCPIP) [mmol (DCPIP) kg−1 (Chl) s−1 and photosynthetic activity [s−1 kg−1 (f.m.) of wheat plants irrigated by seawater.
Reductions in pigment content under seawater stress were in accord with those observed in many plant species [Citation34]. The adverse effect of seawater irrigation on pigment content could be also ascribed to the reduced activity of 5-aminolevulinic acid dehydratase (ALA-D) which catalyzes the formation of the porphyrin ring utilized in the building of chlorophyll. Thus, the conditions that suppress ALA-D synthesis will subsequently interfere with chlorophyll synthesis. In accordance with this assumption, It was reported that water stress reduced the leaf content of Chl a and Chl b, with a concomitant inhibition in ALA-D activity in maize plants [Citation35].
Cytokinins are known to increase accumulation of chlorophylls and carotenoids distribution of chloroplasts around the cell's periphery was observed in control plants, the chloroplasts of seawater-treated cells appeared “bulbous” and discrete. Grain presoaking in kinetin caused the chloroplast of stressed plants to be more regular. Starch grains were larger in size and the membrane system of grana and intergranal lamellae was somewhat organized. More specifically, some projections in the form of tails were recorded.
Grain presoaking in kinetin enhanced the production of different pigment in flag leaves of stressed and unstressed wheat plants. In agreement with these results, Demetriou et al. [Citation36] found that when kinetin was applied to Raphanus sativus plants, it led to substantial production of pigment. The stimulating effect of kinetin on pigment production may be attributed to the ability of this hormone to regulate the biogenesis of chloroplast which was previously manifested as one of the typical biological activities of cytokinins. This phytohormone is known to control the development of chloroplasts, biogenesis of photosynthetic membranes and synthesis of chlorophyll as well as senescence resistance. In addition, the dramatic effect exerted by kinetin may be due to the fact that kinetin could increase the rate of transpiration Aldesuquy et al. [Citation37] and this could result in increased rate of translocation of minerals and cytokinins from root to the developing shoot. In addition, kinetin was presumed to increase the activity and concentration of some protochlorophyllide-synthesizing enzymes [Citation38]. Supplementation of cytokinin could eventually increase the number of chloroplasts in the leaf of Vigna sinensis and Zea mays plants by increasing both intensity of cell growth phytohormones and the activity of cytoplasm ribosomes and consequently stimulate chlorophyll synthesis [Citation39].
The rate of transformation of soluble photosynthates to insoluble ones and the Hill reaction were drastically reduced in seawater stressed wheat plants. This inhibition of photosynthesis may be due the reduction of chloroplast numbers as well as the decline in chlorophyll content in consequence to reduction in leaf area. Salt stress which resulted in the suppression of photosynthetic capacity might be ascribed to stomatal closure [Citation40], chlorophyll content loss, inhibition of Ribulose 1,5 bisphosphate carboxylase oxygenase (Rubisco) activity [Citation41], and degradation of membrane proteins in photosynthetic apparatus [Citation42]. High salinity induced a decrease in photosynthetic efficiency is often associated with inhibition of photosystem II (PSII) [Citation8,Citation9]. PSII has been considered the main site of salt stress-mediated damage to electron transport processes [Citation10], yet the damage site of PSII seems to vary with the severity and duration of stress [Citation11] and plant species [Citation12].
The positive of grain pretreatment with kinetin, spermine (Spm) or their interaction on seawater stressed wheat plants was more pronounced with kinetin than the other ones. In this connection, there are many reports on plant hormones stimulation on chloroplast development as well as the whole photosynthetic machinery [Citation5,Citation43]. Generally, the inhibition of photosynthesis by seawater was alleviated and even promoted over the control value by all pretreatments especially kinetin. However, the leaf area was inhibited by seawater and might undergo changes in chemical composition that would affect the photosynthesis. A very important point was that the plants at salinity might respond as plant under water stress, and thus the reduction in photosynthesis could well be the result of more closed stomata, which may wider opened by kinetin, spermine and their interaction.
In the present investigation, grain priming with Spm, whether alone or in combination with kinetin, seemed to enhance the accumulation of different pigment fractions in seawater-stressed and unstressed wheat plants. Similar promoting effects of polyamines (PAs) on photosynthetic pigments had been observed by El-Bassiouny et al. [Citation44] on mulberry, bean and wheat plants; respectively.
A possible explanation for the promoting effect of exogenously-applied spermine on photosynthetic pigment of wheat plants is that PAs might retard chlorophyll destruction and/or increase its biosynthesis. The role of PAs in chlorophyll synthesis is supported by Askar and Treptow [Citation45] who suggested that the enzyme diamine aminotransferase transfers the amino group from PAs to oxoglutaric acid which is the precursor of chlorophyll. Furthermore, retention of photosynthetic pigments by PAs via the interaction with thylakoid membrane was also stated by Gonzalez-Aguilar et al. [Citation46] who demonstrated that PAs may retard senescence by altering the stability and permeability of such membranes, in addition to protecting membranes and preventing chloroplast from senescing and therefore retarding chlorophyll loss.
It was observed that certain changes in PAs were correlated with changes in the structure and function of the photosynthetic apparatus, such as the increase in the functional size of the antenna as light-harvesting centers [Citation47]. Thus, the enhanced effect of Spm on ultrastructural features of chloroplast may be another reason for pigment retention in seawater-stressed wheat plants. In accord to these results Shu et al. [Citation1] found that spermidine (Spd) is involved in the protection of photosynthetic pigments content under salt stress. Spd induced an increase in the amount of both total Chl and Chl a/b ratios as compared with non-Spm treated salt-stressed plants. Furthermore, Chattopadhayay et al. [Citation47] demonstrated that at physiological concentrations, Spd and Spm significantly prevented chlorophyll loss and down-regulated chloroplast-encoded genes, such as psbA, psbB, psbE and rbcL. Similarly, it was reported that Spd mitigated the degradation of chlorophyll in salt-stressed rice [Citation48]. Therefore, this result indicated that Spm could protect the integrity of PSII from salinity-induced damage.
The study of Chattopadhayay et al. [Citation47] using putrescine, Spd and Spm on rice plants demonstrated the retardation of leaf senescence via the retardation of chlorophyll loss. Furthermore, there is good evidence for hormonal regulation of ion uptake by plant cells. In the present study, the increases in chlorophyll contents as a result of kinetin and/or Spm treatments concomitantly with increasing Mg levels [Citation37] in differently treated wheat plants could be attributed to the role of Mg ion as a structural component of chlorophyll and reinforced the role of kinetin or Spm in chlorophyll biosynthesis. Similar results were obtained by El-Bassiouny et al. [Citation44] by applying Put to wheat plants.
The results of the present work indicated a substantial increase of carotenoid levels in Spm-pretreated wheat plants. Carotenoids may act as photoreceptor pigment [Citation36]. The accumulation of carotenoids is of great significance to the photosynthetic plant cells, where there are at least five roles of carotenoids to play in photosynthesis. These are; (i) light harvesting via singlet state energy transfer, (ii) photoprotection via the quenching of chlorophyll triplet state, (iii) singlet oxygen scavenging, (iv) excess energy dissipation and (v) plastid structural stabilizing [Citation49].
Salinity-induced ultrastructural alterations in flag leaf mesophyll cells of seawater-irrigated wheat plants were highlighted in the present investigation using transmission electron microscopic examination. Chloroplasts seemed to be the organelles more sensitive to salt stress. From TEM micrographs, a continuous “end-to-end” distribution of regular (oval or elliptical) chloroplasts around the cell's periphery was observed in flag leaf mesophyll cells of control wheat plants. Conversely and for seawater-stressed plants, the irregular spherical chloroplasts appeared “bulbous” and discrete, and the cells also displayed extensive but thin peripheral cytoplasmic regions devoid of chloroplasts. In this connection, it was reported that the rounding of chloroplasts may be due to the swelling of thylakoids, and that this causes the stroma of chloroplasts to be less dense with disorganized membrane system [Citation16]. This study revealed that seawater salinity induced disruption of membrane system of chloroplast with swelling thylakoids. These results fit well the observations of Aldesuquy and Baka [Citation50] who recorded similar ultrastructural changes in seawater-stressed wheat plants.
Swelling of thylakoids and disorganized membrane system of chloroplasts in response to salt stress was also reported for different plant species. Swollen thylakoids, disintegration of chloroplast membranes and the round shape of chloroplasts were the ultrastructural changes induced by salinity in Phragmites communis plants [Citation51].
It is common that grana, composed of layered thylakoids, appear to be much more regular and densely stacked, and the intergranal thylakoids are distinct and well-developed in chloroplasts of control tissues. Meanwhile, in salt-stressed cells, grana are disrupted and the thylakoids within them are loosely and unevenly stacked [Citation52]. The structural change and swelling of thylakoid caused by seawater stress in the present study are probably due to a change in the ionic composition of the stroma liquid [Citation52]. Furthermore, with the observed alteration in the typical structure of chloroplasts in response to seawater irrigation in the present investigation, the ability of these organelles to synthesize the pigments in an amount and rate allowing the photosynthetic machinery to work efficiently should be reduced. This was reflected in the marked suppression in the content of different pigment fractions. As a consequence, the amount of sugars manufactured during photosynthesis is lowered resulting in the appearance of starch grains not only smaller in size but also fewer in number for seawater-stressed wheat plants.
It is clear from the results of the present study that the application of exogenous kinetin could counteract the injurious effects of seawater irrigation on ultrastructural features of mesophyll cells of wheat flag leaves. Grain presoaking in 0.1 mM kinetin caused the chloroplast of stressed wheat plants to be more regular, with organized membrane system, large starch grains and projections in the form of tails. Cytokinins are known to stimulate chloroplast maturation and grana development as well as amyloplast formation [Citation53]. It was demonstrated that the internal structure of chloroplasts was maintained in cytokinin-treated wheat leaves during senescence, with well-organized grana thylakoids and small plastoglobuli [Citation54]. In contrast, chloroplasts of control leaves deteriorated rapidly with disorganized internal membranes, and more and larger plastoglobuli (osmiophilic globules).
Transmission electron micrograph cleared that grain presoaking in kinetin caused the chloroplast of stressed wheat flag leaves to appear as dividing. As a similar effect, Aldesuquy and Baka [Citation50] reported that application of GA3 to salt-stressed wheat plants appreciably enhanced chloroplast division. For that, cytokinins are considered to be crucial endogenous regulators of chloroplast biogenesis. They activate the synthesis of chloroplast proteins and photosynthetic pigments, enhance expression of genes encoding enzymes of chlorophyll biosynthesis and accelerate differentiation of etioplasts and chloroplasts. The effects of cytokinins on chloroplasts are mostly related to the involvement of phytohormones in the control of expression of nuclear and chloroplast genes encoding plastid proteins [Citation55].
Polyamines are known to be involved in the delay or prevention of plant senescence [Citation56]. Ultrastructural analysis cleared that grain priming with Spm, either alone or in combination with kinetin, caused the chloroplast in flag leaf mesophyll cells of stressed wheat plants to be more regular in shape with more starch grains. Spermine-promoting efficacy to intensify starch grains in chloroplast of stressed wheat plants may be related to the enhanced accumulation of photosynthetic pigments in flag leaves of the plants treated with Spm. Higher pigment content resulted in faster photosynthesis with the production of more starch.
The alleviation of adverse effects of seawater by kinetin and/or Spm application may be due to the increase in grana number which consequently increased thylakoids. In this regard, it is well known that the increase in thylakoids resulted in an increase in chlorophyll content. In addition, greater stacking of grana may confer an advantage by providing a suitable environment for energy transfer (PSI and PSII) and improving the control of light harvesting and photochemical operations [Citation50].
The greater stacking of grana induced by kinetin, Spm or their interaction may explain the accumulation of carotenoids in flag leaf of wheat plants. These results are in agreement with those obtained by Weier and Benson [Citation57] who suggested that the accumulation of carotenoids pigment as a result of stacking may protect chlorophyll pigment from bleaching by light at high intensities.
5 Conclusions
In conclusion, the changes in pigment content and photosynthetic activity of flag leaf appeared to depend mainly on chloroplast ultrastructure and its numbers, showing a positive correlation between chloroplasts number and pigment content. Application of exogenous kinetin, Spermine or their interaction increased leaf expansion and pigment content as well as improved the photosynthetic capacity of the seawater stressed wheat plants. Furthermore, the positive effect of Spm on photosynthesis might be involved in exogenous Spm regulating endogenous polyamines as well as the physiological effects of exogenous Spm on the structure and function of chloroplast. The applied kinetin or spermine and their interaction on ultrastructural characteristics of wheat flag leaves are reflected on enhancing overall growth and productivity of wheat plants as indicated by noticeable accumulation of photosynthetic pigments as well as photosynthetic activity of flag leaf at ear emergence.
Notes
Peer review under responsibility of Mansoura University
References
- S.ShuY.L.YuanS.R.GuoL.SunC.LiuEffects of exogenous spermidine on photosynthesis, xanthophyll cycle and endogenous polyamines in cucumber seedlings exposed to salinityAfr J Biotechnol1201260646074
- X.LiuB.HuangPhotosynthetic acclimation to high temperatures associated with heat tolerance in creeping bentgrassJ Plant Physiol165200819471953
- A.H.El-NahryA.Y.HammadAssessment of salinity effects and vegetation stress, West of Suez Canal, Egypt using remote sensing techniquesJ Appl Sci Res52009316322
- M.M.AzoozA.M.HassaneinF.A.FaheedRiboflavin (vitamin B) treatments counteract the adverse effects of salinity on growth and some relevant physiological responses of Hibiscus sabdariffa L. seedlingsBull Fac Sci Assiut Univ312002295303
- H.S.AldesuquyA.M.GaberEffect of growth regulators on Vicia faba plants irrigated by seawater: leaf area, pigment content and photosynthetic activityBiol Plant351993519527
- A.Kishore KumarC.Abdul JaleelP.ManivannanB.SankarR.SridharanR.SomasundarametalChanges in biochemical constituents under salinity in Solenostemon rotundifolius (Poir.) MortonIndian J Appl Pure Biol222007223225
- A.M.HamedE.A.M.AliEffect of different seawater concentrations on growth parameters of pineapple (Ananas comosus) in vitro and in vivoJ Appl Sci Res32007713722
- H.M.KalajiG.K.BosaJ.KościelniakK.Żuk-GołaszewskaEffects of salt stress on photosystem II efficiency and CO2 assimilation of two Syrian barley landracesEnviron Exp Bot1020101016
- J.R.XiaY.J.LiD.H.ZouEffects of salinity stress on PSII in Ulva lactuca as probed by chlorophyll fluorescence measurementsAquat Bot802004129137
- P.MehtaA.JajooS.MathurS.BhartiChlorophyll a fluorescence study revealing effects of high salt stress on photosystem II in wheat leavesPlant Physiol Biochem4820101620
- A.J.MisraS.M.SahuM.MisraP.SinghI.MeeraN.DasetalSodium chloride induced changes in leaf growth, and pigment and protein contents in two rice cultivarsBiol Plant391997257262
- M.MegdicheK.HessiniF.GharbiC.A.JaleelR.KsouriC.AbdellyPhotosynthesis and photosystem 2 efficiency of two salt adapted halophytic seashore Cakile maritima ecotypesPhotosynthetica462008410419
- J.A.HernándezE.OlmosF.J.CorpasF.SevillaL.A.DelrioSalt-induced oxidative stress in chloroplasts of pea plantsPlant Sci1052006151167
- N.GeisslerS.HussinH.W.KoyroInteractive effects of NaCl salinity and elevated atmospheric CO2 concentration on growth, photosynthesis, water relations and chemical composition of the potential cash crop halophyte Aster tripolium LEnviron Exp Bot652009220231
- G.N.AmzallagH.R.LenerA.Polijakoff-MayberExogenous ABA as a modulator of the response of Sorghum to high salinityJ Exp Bot541199015291534
- V.KasinathanA.WinglerEffect of reduced arginine decarboxylase activity on salt tolerance and on polyamine formation during salt stress in Arabidopsis thalianaPhysiol Plant1212004101107
- S.H.ShahKinetin improves photosynthetic and antioxidant responses of Nigella sativa to counteract salt stressRuss J Plant Physiol582011454459
- S.H.ShahGrowth and photosynthetic characteristics of Nigella sativa L. as affected by presowing seed treatment with kinetinPhotosynthetica492011154160
- H.S.AldesuquyZ.A.M.BakaB.M.MickkyDoes exogenous application of kinetin and spermine mitigate the effect of seawater on yield attributes and biochemical aspects of grains?J Stress Physiol Biochem920132134
- S.A.QuarrieH.G.JonesGenotype variation in leaf water potential, stomatal conductance and abscisic acid concentration in spring wheat subjected to artificial drought stressAnn Bot441979323332
- D.I.ArnonCopper enzymes in isolated chloroplasts: polyphenoloxidase in Beta vulgarisPlant Physiol241949115
- A.TrebstMeasurement of Hill reaction and photoreductionS.A.PietroMethods in enzymology1972Academic PressNew York146165
- A.M.WoodsJ.L.GayEvidence for a neckband delimiting structural and physiological regions of the host plasma membrane associated with haustoria of Albugo candidaPhysiol Mol Plant Pathol2319837388
- B.E.JuniperG.C.CoxA.J.GilchristP.K.WilliamsTechniques for plant electron microscopy1970Blackwell Scientific PublicationsOxford
- G.W.SnedecorW.G.CochranStatistical methods6th ed.1970Oxford IBH Publishing CoNew Delhi
- A.K.ParidaA.B.DasSalt tolerance and salinity effects on plants: a reviewEcotoxicol Environ Saf602005324349
- R.MunnsJ.M.GuoJ.B.PassiouraG.R.CramerLeaf water status controls day-time but not daily rates of leaf expansion in salt-treated barleyAust J Plant Physiol272000949957
- B.PitannS.SchubertK.H.MühlingThe role of plasma membrane H+-ATPase and apoplastic pH in adaptation of maize (Zea mays) to salt stressJahrestag Deutschen Gesellschaft für Pflanzenernährung, Osnabrückvol. 620091112
- L.AguirrezabalC.S.BouchieA.RadziejwoskiM.DauzatS.J.CooksonC.GranierPlasticity to soil water deficit in Arabidopsis thaliana: dissection of leaf development into underlying growth dynamic and cellular variables reveals invisible phenotypesPlant Cell Environ29200622162227
- H.A.S.OudaPredicting the effect of water and salinity stresses on wheat yield and water needsJ Appl Sci Res22006746750
- A.C.JaleelB.SankarR.SridharanR.PanneerselvamSoil salinity alters growth, chlorophyll content, and secondary metabolite accumulation in Catharanthus roseusTurk J Biol3220087983
- A.E.RichmondA.LangEffect of kinetin on protein content and survival of detached Xanthium leavesScience1251975650651
- M.IqbalM.AshrafWheat seed priming in relation to salt tolerance: growth, yield and levels of free salicylic acid and polyaminesAnn Bot Fenn432006250259
- F.KhosravinejadR.HeydariT.FarboodniaEffects of salinity on photosynthetic pigments, respiration, and water content in two barley varietiesPak J Biol Sci11200824382442
- R.JameiR.HeidariJ.KharaS.ZareThe interaction effects of flooding and kinetin on growth criteria, chlorophyll content and 5-aminolevulinic acid dehydratase activity in corn seedlingsTurk J Biol322008253257
- G.DemetriouC.NeonakiE.NavakoudisK.KotzabasisSalt stress impact on the molecular structure and function of the photosynthetic apparatus: the protective role of polyaminesBiochim Biophys Acta (BBA) – Bioenerg17672007272280
- H.S.AldesuquyZ.A.M.BakaB.M.MickkyRole of kinetin and spermine in the reversal of seawater stress-induced alteration in growth vigor, water relations and nucleic acids of wheat plantsPhyton5412013 [in press]
- L.F.DemetriouEffect of kinetin on the state of the protochlorophyll pigment in the inner membrane system of etioplastsBiochem (Moscow) Suppl Ser A, Membr Cell Biol22008237242
- O.A.EL-ShahabyM.M.NematallaM.E.YounisZ.M.ElbastawisyEffect of kinetin on photosynthetic activity and carbohydrate content in waterlogged or seawater-treated Vigna sinensis and Zea mays plantsPlant Biosyst1362003277288
- D.A.MeloniM.A.OlivaC.A.MartinezJ.CambraiaPhotosynthesis and activity of superoxide dismutase, peroxidase and glutathione reductase in cotton under salt stressEnviron Exp Bot4920036976
- E.BrugnoliO.BjörkmanGrowth of cotton under continuous salinity stress: influence on allocation pattern, stomatal and nonstomatal components of photosynthesis and dissipation of excess light energyPlanta1871992335347
- M.A.KhanI.A.UngarEffects of thermoperiod on recovery of seed germination of halophytes from saline conditionsAm J Bot841997279283
- A.WalidR.ZerbeS.KindThe influence of IAA on the photosynthetic apparatus of Sinapis alba plants grown under high light conditionG.AkoyonoglouPhotosynthesisvol. 61981Balaban Int. Sci. Serv.Philadelphia339348
- H.M.El-BassiounyH.A.MostafaS.A.El-KhawasR.A.HassaneinS.I.KhalilA.A.Abd El-MonemPhysiological responses of wheat plant to foliar treatments with arginine or putrescineAust J Basic Appl Sci2200813901403
- A.AskarH.TreptowBiogene amine in lebensmittlen a Veleg Eugen ulmer, Stuttgart. grown in the dark and at different light quanta fluence ratesPhotochem Photobiol352006217221
- G.A.Gonzalez-AguilarL.ZacariasM.MulasM.T.LafuenteTemperature and duration of water dips influence chilling injury, decay and polyamine content in Fortune MandarinsPostharvest Biol Technol1219976169
- M.K.ChattopadhayayB.S.TiwariG.ChattopadhyayA.BoseD.N.SenguptaB.GhoshProtective role of exogenous polyamines on salinity-stressed rice (Oryza sativa) plantsPhysiol Plant1162002192199
- A.RoychoudhuryS.BasuD.N.SenguptaAmelioration of salinity stress by exogenously applied spermidine or spermine in three varieties of indica rice differing in their level of salt toleranceJ Plant Physiol1682011317328
- D.W.LawlorLimitations to photosynthesis in water-stressed leaves: stomatal vs. metabolism and the role of ATPAnn Bot892002871885
- Aldesuquy HS, Baka ZAM. Interactive effects of seawater and plant hormones on the pigments content and chloroplast ultrastructure of wheat flag leaf. Proceedings, sixth Egyptian botanical conference, Cairo University, Giza, November 24–26, 1998, vol. 1. p. 51–64.
- J.H.LiuH.KitashibaJ.WangY.BanT.MoriguchiPolyamines and their ability to provide environmental stress tolerance to plantsPlant Biotechnol242007117126
- C.F.SmethurstW.M.GillS.ShabalaUsing excised leaves to screen lucerne for salt tolerance: physiological and cytological evidencePlant Signal Behav420093941
- H.A.Zavaleta-ManceraH.López-DelgadoH.Loza-TaveraM.Mora-HerreraC.Trevilla-GarcíaM.Vargas-SuárezetalCytokinin promotes catalase and ascorbate peroxidase activities and preserves the chloroplast integrity during dark senescencePlant Physiol164200715721582
- Y.O.ZuboM.V.YamburenkoS.Y.SelivankinaF.M.ShakirovaA.M.AvalbaevN.V.KudryakovaetalCytokinin stimulates chloroplast transcription in detached barley leavesPlant Physiol148200810821093
- H.Y.NakataniJ.BarberJ.A.ForresterSurface charges on chloroplast membranes as studied by particle electrophoresisBiochim Biophys Acta (BBA) – Bioenerg5041987215225
- A.S.CohenS.ZalikMagnesium replacement by polyamines in higher plant cell-free polyphenylalanine synthesisPhytochemistry171987113118
- T.E.WeierA.A.BensonThe molecular organization of chloroplast membranesAm J Bot541967389402