Abstract
Mass drug administration against malaria and parasitic worm co-infections is capable of increasing health risk. This study investigated the hepatotoxicity, genotoxicity and oxidative stress induced by combinations of Arthemether-Lumefantrine (A-L) with Albendazole (ABZ) and Ivermectin (IVR) treatments in rats. 65 rats equally distributed into 13 groups were orally gavaged human therapeutic doses (×1.0), half of the doses (×0.5) and twice the doses (×2.0) of these drugs per body weights. Blood, liver and bone marrow cells were analyzed for serum biochemistry, histopathology and micronucleated polychromatic erythrocytes (MNPCE) respectively. Treated rats showed clinical signs of toxicity. Aspartate aminotransferase (AST), alanine aminotransferase (ALT), total bilirubin and malondialdehyde (MDA) significantly increase with concomitant decrease in superoxide dismutase (SOD) and catalase (CAT) in the serum. Liver histology revealed single cell hepatocellular necrosis and kupffer cell hyperplasia, multiple foci vacuolar changes in the hepatocytes, thinning of hepatic cord and congestion of the sinusoids by inflammatory cells. Also, frequency of MNPCE significantly increased in the treated rats. The findings revealed that combine treatment of A-L with ABZ and IVR mostly at ×2.0 and ×1.0 induced liver dysfunctions and somatic mutations through oxidative stress in rats. These suggest health risk in wildlife and human populations during treatments with these drug combinations.
1 Introduction
Parasites infect more than 200 million people worldwide. Chronic infestations may elicit inflammation, lead to cancer formation and deaths [Citation1]. Infections from parasitic worms and protozoa are the major cause of human and wildlife morbidity and mortality. In tropical and sub-tropical regions, Plasmodium species, the parasitic protozoan responsible for human and animal malaria, is the leading cause of morbidity and mortality with children highly vulnerable, accounting for about 1–2 million deaths annually [Citation2]. Similarly, schistosomiasis, lymphatic filariasis, ascariasis, enterobiasis and onchocerciasis caused by helminthes and nematodes, are common health issues in most tropical and sub-tropical countries [Citation3]. In endemic situations, these protozoan and worms coexist in human and animals to cause severe infestation and death [Citation4]. In this situation, mass drug administration is usually the recommended treatment strategy for effective parasite clearance [Citation5].
Coartem®, an artemisinin combination therapy containing Artemether-Lumefantrine (A-L), is the leading and first line drug recommended for the treatment of acute and complicated malarial fever in patients with minimum 5 kg body weight [Citation6–Citation[7]Citation8]. Clinical trials showed that Coartem® is effective, safe and has good tolerability against multi-drug resistant Plasmodium falciparum [Citation9,Citation10]. It produces about 10,000 folds decrease in parasite biomass per asexual cycle [Citation11]. Albendazole (ABZ) and Ivermectin (IVR) are effective anti-parasitic agents usually recommended for the treatment of human and veterinary parasitic worm infections [Citation12–Citation[13]Citation14]. Co-administration of these drugs with A-L during helminthic, nematode and malarial co-infection or resistance has shown to be effective. Horton et al. [Citation15] reported that single dose of IVR and ABZ combined therapy against lymphatic filariasis produced better treatment efficacy than the individual drug effects. Mohammed et al. [Citation16] also reported that co-administration of praziquantel and ABZ was effective against schistosomiasis and soil-transmitted helminthiasis morbidity. A-L a fixed-dose combination tablet of 20 mg artemether and 120 mg lumefantrine in the ratio 1:6 is effective against complicated P. falciparum malaria [Citation8,Citation17]. These reports are in support of the efficacy of mass drug administration against co-infections caused by parasitic protozoa and worms.
Considering that drugs are synthetic chemicals, they are capable of producing both beneficial and harmful effects. It is important to evaluate the possible deleterious effects of administering mass drugs during protozoa and worm co-infections to avert the probable health effects. This is necessary considering that the harmful drug effects have been linked to liver damage, bone marrow toxicity, carcinogenesis and fetal developmental anomalies [Citation18,Citation19]. It is plausible that combine drug administration against malaria and parasitic worm co-infection may elicit toxic effects in the host due to drug–drug interactions [Citation20]. In vivo and in vitro studies of individual drugs; A-L, ABZ and IVR induced various toxicities in biological systems. Artemether treatment induced necrosis in gastric cancer cell line (PG100), and necrosis and apoptosis in human lymphocytes [Citation24]. ABZ induced cytotoxicity in liver cells of treated rats via elevation of liver function enzymes and alterations in oxidative stress enzymes during sub-chronic exposure [Citation25]. IVR similarly induce cytotoxicity and genotoxicity in treated mice and Chinese hamster ovary cells [Citation20,Citation26]. These reports showed that A-L, ABZ and IVR are potentially harmful to biological systems. They may be considered effective and safe at therapeutic doses, but an overdose may result into severe tissue injury, organ failure and death [Citation27].
Liver, the most sensitive predictor of chemical induced toxicity due to its involvement in metabolism, detoxification and storage of drugs and their metabolites, is an important target organ for drug induced injury in mammals [Citation25,Citation28,Citation29]. Also, bone marrow the site of blood cell proliferation may be subject to drug induced toxicity during acute and chronic exposure [Citation25,Citation26]. Considering that Artemether and Lumefantrine (A-L), Ivermectin (IVR) and Albendazole (ABZ) may be ignorantly abused following the mass drug administration strategy in the control of malaria and parasitic worm co-infection. It is important to understand the possible mechanism of liver dysfunctions and bone marrow cell toxicity (genotoxicity) of combine treatments of A-L, IVR and ABZ in mammalian systems is linked to reactive oxygen species formation.
This study investigated the hepatotoxicity, genotoxicity (using micronucleus assay) and alterations in serum antioxidant enzymes (catalase and superoxide dismutase) and lipid peroxidation as possible mechanisms of co-administrations of A-L, ABZ and IVR induced toxicity in Wistar rats.
2 Materials and methods
2.1 Chemicals
Artemisinin-based combination therapy containing Artemether and Lumefantrine (A-L); Coartem® (Norvatis Pharmaceuticals Corporation Suffern, New York, USA), Ivermectin (IVR); Mectizan® (Merck & Co., Inc., Whitehouse station, New Jersey, USA) and Albendazole (ALB); Expezol® (Swiss Pharma Nigeria Limited, Lagos), Cyclophosphamide monohydrate (Endoxan™ Mfg Lic. No. 186. Frankfurt am Main, Germany), positive control, Fetal calf serum (Sigma St Louis, MO, USA), Giemsa and May-Grünwald stains (Merck, Germany) used for this study were of analytical grades.
2.2 Animals
Sixty five (65) male Wistar rats (between 7 and 8 weeks old) obtained from the animal unit, College of Medicine, University of Ibadan, Nigeria were used for the study. They were acclimatized for 14 days until they were 134.0 ± 2.0 g (mean ± SD) body weight. They were maintained in laboratory conditions of 12 h dark and light cycle, temperature of 27 ± 8 °C, relative humidity of 69 ± 15% and had access to clean drinking water and standard rodent chow (Ladokun feed Nigeria®) ad libitum. Guide for care and use of Laboratory Animals published by US National Institutes of Health (NIH Publication No. 85–23, revised in 1996), and approved by the ethical committee, University of Lagos for the use of animals in experimental studies was carefully adhered.
2.3 Experimental design and drug administration
5 rats per group were randomly distributed into 13 experimental groups with cyclophosphamide (CYP, 20 mg/kg/bwt) and distilled water (vehicular solvent) used as positive and negative controls respectively. Human therapeutic doses for the three drugs (×1.0), sub-curative (half of the dose; ×0.5) and twice the doses (×2.0) were administered to the rats via oral gavaging according to their body weights (mg/kg). The experimental groups are A (negative control), B [human therapeutic dose for Artemether-Lumefantrine, 20/120 mg/kg bwt (×1.0 AL)] [Citation7,Citation10], C [sub-curative human therapeutic dose for Artemether-Lumefantrine, 10/60 mg/kg bwt (×0.5 AL)], D [twice the human therapeutic dose for Artemether-Lumefantrine, 40/240 mg/kg bwt (×2.0 AL)], E [human therapeutic doses for Artemether-Lumefantrine, 20/120 mg/bwt and Albendazole, 400 mg/kg bwt (×1.0 AL + ABZ)] [Citation39], F [sub-curative human therapeutic doses for Artemether-Lumefantrine, 10/60 mg/bwt and Albendazole, 200 mg/kg; (×0.5 AL + ABZ)], G [(twice of the therapeutic doses Artemether-Lumefantrine, 40/240 mg/bwt and Albendazole, 800 mg/kg (×2.0 AL + ABZ)], H [human therapeutic doses for Artemether-Lumefantrine, 20/120 mg/bwt and Ivermectin, 3 mg/kg (×1.0 AL + AVR)] [Citation13,Citation41], I [sub-curative therapeutic doses for Artemether-Lumefantrine, 10/60 mg/bwt and Ivermectin, 1.5 mg/kg (×0.5 AL + IVR)], J [twice of therapeutic doses for Artemether-Lumefantrine, 40/240 mg/bwt and Ivermectin, 6 mg/kg (×2.0 AL + IVR)], K [therapeutic doses for Artemether-Lumefantrine, 20/120 mg/bwt and single administration of Albendazole, 400 mg/kg], L [therapeutic dose for Artemether-Lumefantrine, 20/120 mg/bwt and single administration of Ivermectin, 3 mg/kg] and M [Cyclophosphamide, (CYP) 20 mg/kg]. The drugs were administered to the various groups for three consecutive days. Similar treatment was concurrently given to the negative control group, A (distilled water; vehicular solvent). presents the summary of the experimental design for drug administration to the various groups.
Table 1 Experimental animal grouping and drug dosage and administration.
2.4 Clinical signs of toxicity and mortality
Rats in each treatment group were observed twice daily (before and after exposure) for signs of clinical toxicity in the appearances of their skin and fur, eyes and mucous membrane, behavioral pattern, morbidity and mortality.
2.5 Serum biochemical analysis
At the end of exposure periods, rats were fasted overnight and blood collected from the orbital plexus using heparinized 70 ml micro-hematocrit capillary tubes into lithium coated serum separator tubes. The clotted blood was centrifuged at 3000 g for 10 min to separate the serum (supernatant) and stored at −70 °C prior to biochemical analysis. Serum biochemical markers of oxidative stress were measured according to standard protocols: Catalase (CAT; EC 1.11.1.6) activity was measured according to the method of Aebi [Citation30], superoxide dismutase (SOD; EC 1.15.1.1) activity was measured in accordance with Magwere et al. [Citation31] method, while lipid peroxidation was measured as malondialdehyde (MDA) concentrations in accordance with Nichaus and Samuelson [Citation32] method. Protein concentration was measured according to the methods of Lowry et al. [Citation33]. Serum liver function test markers; transaminases were measured according to Reitman and Frankel [Citation34] and total bilirubin according to Treitz [Citation35] using Randox Laboratory (UK) diagnostic kits. The absorbances for all the reactions were measured spectrophotometrically using HAICE®, DR 3000 (Germany).
2.6 Mammalian bone marrow micronucleus assay
Bone marrow micronucleus test was conducted according to Schmid [Citation36]. Femoral bones from treated and control rats were surgically removed and the bone marrow flushed into eppendof tubes using 0.5 ml of Fetal Bovine Serum (FBS). The cells were centrifuged at 2000 rpm for 5 min and smear made on pre-cleaned grease free slides. Prepared slides were air dried and stained with May-Grunwald and Giemsa stains. They were coded and examined under an Olympus light microscope at 1000× magnification. 2000 cells per rat were scored for micronucleated polychromatic erythrocytes (MNPCE).
2.7 Histopathological analysis
Slices of the right lobe of the liver tissue from drug treated and control rats were fixed in 10% neutral buffered formalin. The tissues were dehydrated in ascending order of ethyl alcohol-water concentrations, cleared in xylene and sequentially embedded in paraffin wax blocks using rotary microtome. Tissue sections of 3–5 μm thick were cut and prepared on clean slides for Hematoxylin-Eosin (H-E) staining before mounting in neutral DPX medium. Prepared slides were examined at 400× magnification by trained pathologist.
2.8 Statistical analysis
All statistical analyses were conducted with Graphpad prism 5.0® computer programs. One-way ANOVA was used to determine the differences (p < 0.05) among the various treated groups and control. Difference between each treatment group and the negative control was determined using comparison procedure of the Dunnett multiple post-hoc test (DMPT). Significance was considered at p < 0.05; p < 0.01 and p < 0.001.
3 Results
3.1 Clinical signs of toxicity and mortality
During the exposure periods, two animals died, one each in groups E and G. The survivors in groups D, E, G, H, J, K and L exhibited sluggishness and weak movement (signs of reduced activities), diarrheae, ungroomed fur and labored breathing movement. They also exhibited reduction in their feeding habit. A rat in the H group developed abscess in the thigh region of the right leg (Fig. 1). These signs of toxicity were rarely observed in rats from groups B, C, F and I and none was observed in the negative control group. There was no significant change in the body weight gain and liver weight gain between treatment groups and the negative control (data not shown).
3.2 Biochemical indicators of hepatic function, lipid peroxidation and oxidative stress
Fig. 2(a–c) presents the results of the serum hepatic function tests. The activities of ALT, AST and total bilirubin concentration significantly (p < 0.001) increased in rats exposed to the drug combinations. Drug exposure to rats in groups D, E, G, H, J, K and L resulted in significant increase in ALT activity by 37.69, 38.52, 78.13, 58.72, 94.65, 50.66 and 71.94% folds respectively as well as AST activity by 26.05, 34.74, 98.51, 67.29, 127.77, 66.42 and 81.41% folds respectively compared to the negative control. shows the results of the antioxidant enzyme activities and lipid peroxidation. SOD and CAT activities significantly (p < 0.05) decreased in the drug administered groups compared to the negative control, with the reduction dependent on drug treatment groups. The percentage decrease for SOD activities in rats from E, G, H, L, K, and J groups were significantly different from the negative control by 33.33, 40.33, 21.28, 18.41, 15.08 and 29.11% folds respectively. While the percentage decrease for CAT activity in rats from E, G, H, L, K and M groups are 31.82, 47.98, 22.22, 7.07, 17.68 and 31.31% folds respectively. Reduction in the activities of these enzymes is accompanied with concomitant significant (p < 0.05) increase in MDA concentration with 34.78, 73.46, 2.13, 11.0, 14.11 and 48.36% folds increase for rats in the treatment groups: E, G, H, L, K and J respectively compared to the negative control. The results of the biochemical alterations showed positive correlations according to the drug administration (Fig. 2a–c and ).
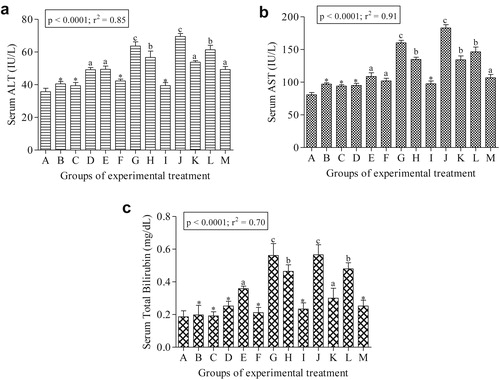
Table 2 Effects of drug combinations on serum CAT, SOD and MDA in treated and control rats.
3.3 Micronucleus analysis
Fig. 3 shows the genotoxicity data. There is significant increase (p < 0.05) in the frequencies of micronucleated polychromatic erythrocytes. The treatment groups: E, G, H, J, K and L were significantly higher than the negative control by 6.78, 8.12, 1.81, 3.11, 5.49 and 2.97 folds respectively. Fig. 4 shows representative polychromatic erythrocyte (PCE) and micronucleated PCE scored for the genotoxicity assessment of the drugs.
3.4 Histopathological assessment of the liver
Fig. 4a–Fig. 4Fig. 4Fig. 4Fig. 4Fig. 4f presents the histological sections of the liver from the treated rats and the negative control. Sections of the liver from the negative control rats showed apparently normal hexagonal or pentagonal lobule of the hepatocytes. These cells are regular and contain a large spheroidal nucleus (Fig. 4a). Histology of the liver tissues from combined drug treated rats showed some distorted architectural structures from the negative control. These alterations are single cell hepatocellular necrosis and kupffer cell hyperplasia, which were common among most treated rats in the following groups; D, E, G, H, J, K, L and the positive control. Also multiple foci vacuolar changes in the hepatocytes, thinning of hepatic cord and congestion of the sinusoids by inflammatory cells were observed in these treated groups (Fig. 5b–f).
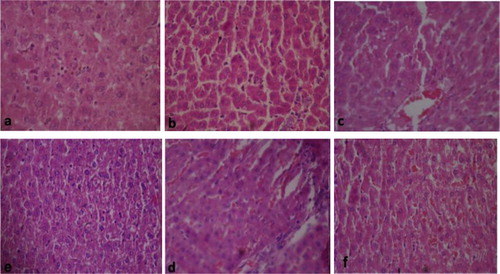
4 Discussion
Mass drug administration strategy against protozoa and worm co-infections [Citation8] is capable of increasing human exposure to multiple drug combinations. Mostly in developing countries where drugs are readily purchased from unregistered patent medicinal stores and hawkers without physician prescriptions. Free access to drugs, coupled with wrong application due to ignorance of drug toxicity may endanger human and animal health. Ivermectin, Albendazole and Artemether-Lumefantrine used in this study were selected based on their overwhelmingly and extensively use as broad-spectrum anti-nematode, anti-helminthic and antimalarial drugs respectively [Citation5,Citation7,Citation13,Citation14,Citation37]. Also individual toxic effects of these drugs have been observed in mammalian systems [Citation21–Citation[22]Citation23,Citation25,Citation27,Citation38,Citation39], but there is dearth of information on the toxic effects of their binary combinations. This study corroborated oxidative stress induced by binary combinations of AL, AL + ABZ and AL + IVR with structural and biochemical alterations in the liver, and micronuclei polychromatic erythrocyte formation in the bone marrow cells of rats.
Clinical signs of toxicity observed in the treated rats in groups D, E, G, H, J, K and L suggest systemic toxicity of the drug combinations in the treated rats. Anorexia (loss of appetite) is a common symptom of liver injury attributable to drug exposure and is consistent with reduced activities; sluggishness and weakness [Citation40]. Labored breathing, diarrheae, ungroomed hair and hair loss observed in the treated rats are common symptoms of hepatotoxicity and immune systems dysfunction due to toxicosis from overdose of the administered drugs and their pharmacokinetics [Citation41,Citation42]. Mortality recorded at the ×1.0 AL + ABZ (group E) and ×2.0 AL + ABZ (group G) treatment may be associated with overdose which caused acute toxicity. Abscess observed in a rat from group H (×1.0 AL + IVR) may be associated with cellular inflammatory actions induced by the drug combination. This assertion lends credence to the observed necrotic cells in the liver of the ×1.0 AL + IVR treated rats. Moreover, high dose administration of Ivermectin induced neutrophilic activation and eosinophilic bursting of the human peripheral blood [Citation43,Citation44] further supported the cellular inflammatory actions of the drugs. Generally inflammatory cells; macrophages, neutrophils and lymphocytes function by destroying invading microorganisms, removal of necrotic cells (accidental cell death) and cellular debris caused by drugs and other xenobiotics during phagocytosis [Citation45,Citation46]. This process is usually associated with intracellular ROS formation via autoxidation [Citation45], which is in support of Hyslop et al. [Citation47] that abscess is associated with inflammatory processes capable of producing ∼100 μM of H2O2 (ROS). Alterations in the anti-oxidant enzymes and lipid peroxidation status of the treated rats compared to the negative control further supported the cellular inflammatory actions.
The liver in mammalian systems is prone to drug-induced injury due to its central role in drug metabolism, detoxification, storage, and portal location within the circulation [Citation48]. It is the most sensitive predictor of chemical toxicity that correlates well with histopathology and serum biochemistry with little inter-animal variations [Citation19]. As the major site of AL, ABZ and IVR metabolism [Citation25,Citation49,Citation50], the liver may be prone to the toxic effects of these drugs and or their metabolites. Cell membrane damage in the liver is usually associated with the release of a number of cytoplasmic enzymes into the circulatory system; this provides the basis for clinical diagnosis. Serum AST and ALT are the most used biochemical markers of hepatocellular necrosis and are considered sensitive indicators of hepatic drug induced injuries [Citation51,Citation52]. Significant increase in the activities of serum ALT, AST and total bilirubin observed in the treated rats mostly from groups D, E, G, H, J, K and L suggests acute hepatocellular injury due to drug induced necrosis. It is suggested that the administered drugs and or their metabolites induced lipid peroxidative damage to the hepatocytes (accidental cell death; necrosis) which increased cell membrane permeability to the cellular enzymes. This is supported by the observation of numerous hepatic necroses in the liver cells and significant increase in serum lipid peroxidation in the treated rats. Moreover the reports that Ivermectin [Citation29], Abendanzole [Citation25], Artemether-Lumefantrine [Citation49] and co-administration of albendazole and ivermectin [Citation50] treated rats at different concentrations significantly increased transaminase concentrations is in concert with the observations herein. Also, increase in serum total bilirubin concentration with concomitant elevation of transaminases in AL treated rats have been associated with hepatocellular injury [Citation53,Citation54]. Histological study yields the most reliable information on the type of lesions induced by drugs and their metabolites in tissues. It is also useful in providing information about acute and chronic effects of toxic substances that may not be detected by biochemical analysis [Citation55,Citation56]. Acute exposure of the drug combinations induced hepatic necrosis, kupffer cell hyperplasia and congestions of the hepatocytes by inflammatory cells observed in the liver cells of the treated rats correlated with the clinical signs and biochemical findings. These lesions were similarly reported in rats dosed with combination of Artemether-Lumefantrine and were attributed to drug induced liver injury [Citation57].
Drug combinations can act in synergy, potentiation and or antagonism to induce alterations in enzyme biochemistry, biological molecules (ROS) and cell membrane [Citation20,Citation58,Citation59]. These alterations may include increase in cellular formation of oxidative stress via creating imbalance between ROS and the antioxidant systems [Citation48]. SOD activity is important in preventing oxidative damage by scavenging and converting superoxide anions to hydrogen peroxides, while catalase causes the decomposition of the hydrogen peroxide to protect tissues from the actions of the highly reactive hydroxyl radicals [Citation52,Citation60]. During these processes the activities of these enzymes may be altered leading to ROS induced pathological disorders and DNA damage [Citation46]. The observed significant decrease in the activities of SOD and CAT in the treated rats suggests harmful effects of the drug combinations (groups D, E, G, H, J, K and L) via excess undetoxified free radical formation. These unscavenged free radicals probably induced lipoperoxide formation which caused lipid peroxidation in the cell membrane (increase MDA concentration) of the treated rats. Studies using rats exposed to AL [Citation49,Citation53] and ABZ [Citation61], and rabbits treated with IVR [Citation62,Citation63] showed reduced SOD and CAT activities either in the serum or liver with concomitant increase in MDA concentrations, are in concert with the findings herein.
Micronucleus (MN) test is the most widely utilized and recommended test for the genotoxic and mutagenic evaluation of chemicals [Citation64,Citation65]. This is due to its technical simplicity and ability to detect both clastogens and aneugens [Citation64]. The significant increase in the MNPCE observed in bone marrow of rats treated with ×2.0 AL, AL + ABZ, ×2.0 AL + ABZ, AL + IVR, ×2.0 AL + IVR in groups D, E, G, H and J respectively suggests DNA damage induced by the tested drug combinations. It is plausible that the drug combinations induced MNPCE was via ROS formation. Since DNA damage may be associated with accidental cell death (necrosis) or apoptosis induced by reactive oxygen species [Citation66]. The combination of these drugs possibly affected the actively proliferative bone marrow cells during the M-phase of the cell cycle to induce oxidative DNA damage in the treated rats. Similar data obtained from the cyclophosphamide treated rats (positive control; group M) is in support of this assertion. Cyclophosphamide, an alkylating agent widely used in cancer chemotherapy [Citation67], is known to alkylate DNA and protein via oxidative stress induction [Citation68,Citation69], leading to cytotoxicity and genotoxicity [Citation68]. Alterations in the serum oxidative stress parameters and increase MNPCE formation in the bone marrow of cyclophosphamide treated rats (positive control; group M), validated the findings herein. Studies similarly reported that mice treated with abendazole harbored significant increase MNPCE in the bone marrow [Citation70]. Pediatric patients with hepatic hydatid disease on abendazole treatment presented significant increase in sister chromatid exchange (SCE) and micronucleus frequencies in peripheral lymphocytes [Citation39]. Also, mice exposed to ivermectin expressed higher chromosome aberration, MNPCE formation and DNA fragmentation compared to the negative control [Citation20]. The combinations of the tested drugs generated reactive oxygen species (ROS) which played important role in MNPCE formation in the treated rats. Somatic DNA damage due to drug toxicity predisposes cells to chromosome related disorders, aging and carcinogenicity [Citation29,Citation39,Citation59,Citation71,Citation72].
The role of oxidative stress in the induction of abnormal cellular functions and pathological disorders are the current research focus for many studies [Citation73–Citation[74]Citation75]. Oxidative stress induced cell damage may occur via a number of mechanisms [Citation74] and the damaged cells may be eliminated either by programmed cell death (apoptosis) or accidental cell death (necrosis). Necrosis, the most common histological lesions observed in the liver of the treated rats, is associated with the destruction of certain signal pathways in the cells and the disruption of mitochondrial functions via inflammatory process [Citation76]. Cellular necrosis and inflammatory cells have been associated with liver damage in Artemether-Lumefantrine treated rats [Citation57]. This study showed that the combination of the tested drugs, mostly at higher doses elicited ROS formation in rats. Oxidative stress induction correlated with cellular necrosis, inflammatory cell production and other pathological lesions observed in the hepatocytes. Also MNPCE formation in bone marrow cells of rats. In conclusion, A + L, AL + ABZ, and AL + IVR drug combinations induced hepatotoxicity and somatic mutation in rats may be associated with oxidative stress induction.
Acknowledgment
The authors appreciate the contributions of Mr. Samuel Akindele, Department of Biochemistry, Nigerian Institute of Medical Research (NIMR), Yaba, Lagos and Dr John, Department of Veterinary Pathology, University of Ibadan, in the biochemical and histological analysis respectively.
Notes
Peer review under responsibility of Mansoura University.
References
- International Agency for Research on Cancer (IARC)IARC monograph on the evaluation of carcinogenic risks to humansSchistosomes, liver flukes and Helicobacter pylorivol. 611994 Lyon
- B.GreenwoodT.MutabingwaMalaria in 2002Nature4152002670672
- World Health Organization (WHO)Prevention and control of schistosomiasis and soil transmitted Helminthiasis Fourth Report of the expert committee. Technical report series No7122002WHO Geneva
- G.RasoA.LuginbuhlC.A.AdjouaN.T.Tian-BiK.D.SilueMultiple parasite infections and their relationship to self-reported morbidity in a community of rural Coˆte d'IvoireInt J Epidemiol33200410921102
- World Health Organization (WHO)Towards accelerated reduction of neglected tropical diseases2012World Health OrganizationGeneva, Switzerland
- A.A.AminG.O.KokwaroAntimalarial drug quality in AfricaJ Clin Pharmacol Ther322007429440
- Z.G.PremjiCoartem®: the journey to the clinicMalar J8200916
- World Health Organization (WHO)Guidelines for the treatment of malaria2nd ed2010World Health OrganizationGeneva, Switzerland
- F.KurthS.BelardA.A.AdegnikaO.GayeP.G.KremsnerM.RamharterDo paediatric drug formulations of artemisinin combination therapies improve the treatment of children with malaria? A systematic review and meta-analysisLancet Infect Dis102010125132
- B.E.NgasalaM.MalmbergA.M.CarlssonP.E.FerreiraM.G.PetzoldD.Blessbornet alEfficacy and effectiveness of artemether-lumefantrine after initial and repeated treatment in children <5 years of age with acute uncomplicated Plasmodium falciparum malaria in rural Tanzania: a randomized trialClin Infect Dis522011873882
- F.NostenN.J.WhiteArtemisinin-Based combination treatment of falciparum malariaAm J Trop Med Hyg772007181192
- W.C.CampbellBenzimidazoles: veterinary usesParasitol Today61990130133
- P.H.GannF.A.NevaA.A.GamA randomized trial of single- and two-dose ivermectin versus thiabendazole for the treatment of strongyloidiasisJ Infect Dis169199410761079
- T.G.GearyIvermectin 20 years on: maturation of a wonder drugTrends Parasitol212005530532
- J.HortonC.WittE.A.OttesenJ.K.LazdinsD.G.AddissAn analysis of the safety of single dose, two drug regimens used in programmes to eliminate lymphatic filariasisParasitology1212000S147S160
- K.A.MohammedH.J.HajiA.GabrielliL.MubilaL.ChitsuloM.H.Bradleyet alTriple co-administration of ivermectin, albendazole and praziquantel in Zanzibar: a safety studyPLoS Neglect Trop Dis2200817
- G.O.AdjeiB.Q.GokaF.BinkaJ.A.KurtzhalsArtemether-lumefantrine: an oral antimalarial for uncomplicated malaria in childrenExptal Rev Anti Infect Ther72009669681
- H.P.RangM.M.DalePharmacology2nd ed1991Longman Goup, UK Limited
- M.G.SturgillG.H.LambertXenobiotic-induced hepatotoxicity: mechanisms of liver injury and methods of monitoring hepatic functionClin Chem43199715121526
- B.H.EssaA.F.El-NahasU.E.MahrousA.S.El-TahawyGenotoxicity of Ivermectin (P-gp inhibitor) as a model of drug-drug interactionAlex J Vet Sci3820122332
- T.G.BrewerJ.O.PegginsS.J.GrateJ.M.PetrasB.S.LevineP.J.Weinaet alNeurotoxicity in animals due to arteether and artemetherTrans R Soc Trop Med Hyg881994S33S36
- A.NontprasertS.PukrittayakameeA.M.DondorpR.ClemensS.LooareesuwanN.J.WhiteNeuropathologic toxicity of artemisinin derivatives in a mouse modelAm J Trop Med Hyg672002423429
- J.M.PetrasD.E.KyleM.GettayacaminG.D.YoungR.A.BaumanH.K.Websteret alArteether: risks of two week administration in Macaca mulattaAm J Trop Med Hyg561997390396
- D.D.AlcântaraH.F.RibeiroP.C.CardosoT.M.AraújoR.R.BurbanoA.C.Guimarãeset alIn vitro evaluation of the cytotoxic and genotoxiceffects of artemether, an antimalarial drug, in a gastric cancer cell line (PG100)J Appl Toxicol332013151156
- M.A.Abd El-RahmanI.M.Abdel-NabiM.A.OmranM.F.MohamedCytotoxic effects of Albendazole, antiparasitic drug, on the liver of the rat: sub-chronic studyEgypt J Biol119991629
- G.MolinariS.SloneskiM.A.ReigosaM.L.LarramendyIn vitro genotoxic and cytotoxic effects of ivermectin and its formulation ivomec on Chinese hamster ovary (CHOK1) cellsJ Hazard Mater15200910741082
- A.O.AbolajiM.U.EtengO.OmonuaY.AdenreleInfluence of co-administration of artemether and lumefantrine on selected plasma biochemical and erythrocyte oxidative stress indices in female Wistar ratsHum Exp Toxicol322013206215
- Y.LinH.ChernM.ChuHepatotoxicity in the review of clinical safety dataDrug Info J372003155158
- S.QureshiBiochemical toxicity of Ivermectin in Wistar albino ratsAme Eura J Toxicol Sci520131519
- H.AebiCatalase estimationH.V.BergmeyerMethods of enzymatic analysis1974Verlag ChemicNew York
- T.MagwereY.S.NaikJ.A.HaslerEffects of chloroquine treatment on antioxidant enzymes in rat liver and kidneyFree Radic Biol Med221997321327
- W.G.NichausB.SamuelsonFormation of malondialdehyde from phospholipid arachidonate during microsomal lipid peroxidationEur J Biochem61968126130
- O.H.LowryN.J.RosebroughtA.L.FarrR.J.RandallProtein measurement with the folin phenol reagentJ Biol Chem1931951265275
- S.ReitmanS.FrankelA colorimetric method for determination of serum glucose oxaloacetate and glutamic pyruvate transaminasesAm J Clin Pathol2819575356
- W.TreitzFundamentals of clinical chemistry with clinical correlation1970W.B. Saunders Philadelphia vary B. detaeselier
- W.SchmidThe micronucleus testMutat Res311975915
- H.R.AdamsChemotherapy of parasitic diseasesVeterinary pharmacology and therapeutics7th eds.1995Iowa state University PressAmes, Iowa 50014898
- S.S.DadarkarM.D.DeoreM.M.GatneComparative evaluation of acute toxicity of ivermectin by two methods after single subcutaneous administration in ratsRegul Toxicol Pharmacol472007257260
- S.OztasA.B.SalmanA.TatarM.YigiterH.YazgiM.Erteket alGenotoxic effect of albendazole in pediatric patients with hepatic hydatid diseaseInt J Infect Dis112007446449
- J.M.CullenMechanistic classification of liver injuryToxicol Pathol33200568
- J.KamgnoJ.GardonN.Gardon-WendelD.NgangueB.O.L.DukeM.BoussinesqAdverse systemic reactions to treatment of onchocerciasis with ivermectin at normal and high doses given annually or three monthlyTrans R Soc Trop Med Hyg982004496504
- A.SaprsaJ.M.BonnetblanceI.PeyrotV.Loustaud-RattiE.VidalC.BedaneSystemic adverse reactions with Ivermectin treatment of scabiesAnn Dermatol Venereol1332006784787
- F.L.NjooC.E.HackJ.OostingJ.S.StilmaA.KijlstraNeutrophils activation in Ivermectin-treated onchocerciasis patientsClin Exp Immunol941993330333
- F.W.TischendorfN.W.BrattigA.HoyerG.C.E.Medina-De LaF.GeisingerModulatory effects of antifilial drugs ivermectin, CGP 6140 and CGP 20376 on the oxidative burst of eosinophilic granulocytesActa Trop5319932737
- H.JaeschkeReactive oxygen species and mechanisms of inflammatory liver injuryJ Gastroenterol Hepatol152000718724
- S.RamaiahH.JaeschkeRole of neutrophils in the pathogenesis of acute inflammatory liver injuryToxicol Pathol352007757766
- P.A.HyslopD.B.HinshawI.U.ScraufstatterC.G.CochraneS.KunzK.VosbeckHydrogen peroxide as a potent bacteriostatic antibiotic: implications for host defenseFree Radic Biol Med1919953137
- A.L.JonesAnatomy of the normal liverD.ZakinT.D.BoyerHepatology: a textbook of liver disease3rd ed1996WB SaundersPhiladelphia
- O.A.AdaramoyeD.O.OsaimojeA.M.AkinsanyaC.M.NnejiM.A.FafunsoO.G.AdemowoChanges in antioxidant status and biochemical indices after acute administration of Artemether, Artemether-Lumefantrine and Halofantrine in ratsBasic Clin Pharmacol Toxicol1022008412418
- R.O.AriseS.O.MalomoEffects of ivermectin and albendazole on some liver and kidney function indices in ratsAfri J Biochem Res32009190197
- G.Y.ChoiH.W.YangS.H.ChoD.W.KangH.GoW.C.Leeet alAcute drug-induced hepatitis caused by albendazoleJ Korean Med Sci232008903905
- J.A.TimbrelPrinciples of biochemical toxicology4th ed2009Informa HealthcareNew York, USA
- G.N.AnyasorO.T.OdunsanyaCoartemether in dietary oil induces oxidative stress and hepatotoxicity in albino ratResearcher320113541
- E.GianniniR.TestaV.SavarinoLiver enzyme alteration: a guide for cliniciansCan Med Assoc J1722005367379
- D.E.AmacherS.J.SchomakerS.E.BoldtM.MirskyThe relationship among microsomal enzyme induction, liver weight and histological change in Cynomolagus monkey toxicology studiesFood Chem Toxicol442006528537
- L.L.LanningD.M.CreasyR.E.ChapinP.C.MannN.J.BarlowK.S.Reganet alRecommended approaches for the evaluation of testicular and epididymal toxicityToxicol Pathol302002507520
- I.F.UkekweP.A.AkaliA.C.EzikeC.O.OkoliAssessment of the sub-acute and delayed toxicity of Artemether-Lumefantrine combination in ratsInt J Res Ayurveda Pharm42013168176
- D.T.DaviesEnzymology in preclinical safety evaluationToxicol Pathol201992501505
- D.Escobar-GarciaR.Camacho-CarranzaI.PerezV.DoradoM.Arriaga-AlbaJ.J.Espinosa-AguirreS9 indution by the combined treatment with cyclohexanol and albendazoleMutagenesis162001523528
- C.MichielsM.RaesO.ToussaintJ.RemacleImportance of Se-glutathione peroxidase, catalase, and Cu/Zn-SOD for cell survival against oxidative stressFree Radic Biol Med171994235248
- E.YarsanS.CeikG.EraslanH.AycicekEffects of albendazole treatment on lipid peroxidation of healthy and Toxocaris canis infected miceIsr Vet Med Assoc J572002111
- E.AtakisiO.AtakisiB.TopcuM.UzunEffect of therapeutic dose of ivermectin on plasma nitric oxide and total antioxidant capacity in rabbitsEuro Rev Med Pharmacol Sci132009425429
- S.K.BeheraU.DimriS.K.SinghR.K.MohantaThe curative and antioxidative efficiency of ivermectin and ivermectin plus vitamin E and selenium treatment on canine Sarcoptes scabiei infestationVet Res Commun352011237244
- G.KrishnaM.HayashiIn vivo rodent micronucleus assay: protocol, conduct and data interpretationMutat Res4552000155166
- T.MoritaN.AsanoT.AwogiY.F.SasakiS.SatoH.Shimadaet alEvaluation of the rodent micronucleus assay in the screening of IARC carcinogens (Group 1. 2A and 2B). The summary report of the 6th collaborative study by CSGMT/JEMS MMSMutat Res38919973122
- Z.OuanesS.AbidI.AyedR.AnaneT.MobioE.E.Creppyet alInduction of micronuclei by Zearalenone in Vero monkey kidney cells and in bone marrow cells of mice: protective effect of vitamin EMutat Res5381–220036370
- R.E.FlemingAn overview of cyclophosphamide and ifosfamide pharmacologyPharmacotherapy17199714651545
- P.ChakrabortyU.H.SkN.MurmuJ.K.DasS.PalS.BhattacharyaModulation of cyclophosphamide-induced cellular toxicity by diphenylmethyl selenocyanate in vivo, an enzymatic studyJ Cancer Mol42009183189
- D.GhoshU.B.DasS.GhoshM.MallickJ.DebnathTesticular gametogenic and steroidogenic activities in cyclophosphamide treated rat: a correlative study with testicular oxidative stressDrug Chem Toxicol252002281292
- F.U.AlkanS.SenerLack of the antimutagenic effect of ascorbic acid on the genotoxicity of albendazole in mouse bone marrow cellsBull Vet Inst Pulawy532009493497
- G.BrozovicN.OrsolicR.RozgajV.KasubaF.KnezevicA.H.Knezevicet alDNA damage and repair after exposure to sevoflurane in vivo, evaluated in Swiss albino mice by the alkaline comet assay and micronucleus testJ Appl Genet5120107986
- F.J.Van SchootenA.Besarati NiaS.De FloraF.D'AgostiniA.IzzottiA.Camoiranoet alEffects of oral administration of N-acetyl-L-cysteine: a multi-biomarker study in smokersCancer Epidemiol Biomarkers Prev112002167175
- B.HalliwellRole of free radicals in the neurodegenerative diseases: therapeutic implications for antioxidant treatmentDrugs Aging182001685716
- K.JomovaD.VondrakovaM.LawsonM.ValkoMetals, oxidative stress and neurodegenerative disordersMol Cell Biochem345201091104
- M.ValkoM.IzakovicM.MazurC.J.RhodesJ.TelserRole of oxygen radicals in DNA damage and cancer incidenceMol Cell Biochem26620043756
- M.D.PulidoA.R.ParrishMetal-induced apoptosis: mechanismsMutat Res5332003227241