Abstract
The role of green tea polyphenon-60 (PP-60) in decreasing metabolic risk factors, oxidative stress, and inflammation and in the amelioration of cardiac apoptosis in experimentally induced diabetes was investigated. After the induction of diabetes in male rats by streptozotocin (STZ; 50 mg/kg, i.p.), PP-60 (100 mg/kg) was orally administered for two weeks. The treatment of the diabetic rats with PP-60 ameliorated hyperglycemia and HbA1c, increased insulin secretion, improved the HOMA-IR level and lipid profile and increased the levels of IL-1b, IL-6, and TNF-α. PP-60 also blunted the increase in the level of MDA and H2O2 and the decreases in the levels of GSH, SOD, and CAT in the heart of STZ-treated rats and improved heart function. PP-60 decreased the apoptosis of cardiomyocytes; enhanced Bcl-2 expression; blocked the increases in p53, Bax, caspases 9, 8 and 3; and ameliorated DNA damage in the heart. Our data suggests that PP-60 decreased apoptosis by blocking DNA damage via the action of caspases and the suppression of pro-inflammatory cytokines. The pleiotropic effects of PP-60, including its anti-hyperglycemic effect, hypolipidemic influence, attenuation of oxidative stress reduction of circulating pro-inflammation cytokines and anti-apoptotic action through modulation of its related regulated proteins, contribute to and accompany the beneficial effects of treatment with PP-60 on diabetic heart dysfunction.
1 Introduction
Diabetes mellitus is one of the most common endocrine metabolic disorders and is associated with marked morbidity and mortality due to cardiac complications [Citation1,Citation2]. The pathophysiology underlying diabetes-induced cardiac damage is complex and is induced by several factors, including elevated oxidative stress, high proinflammatory cytokines and dyslipidemia as the main risk factors [Citation3]. It was recently reported that short-term diabetes induces cardiac antioxidant depletion, increases the oxidative stress levels, and results in cardiac apoptosis as early as three weeks after diabetic induction in rats [Citation4]. A similar finding was reported after the administration of streptozotocin (STZ) for four days [Citation5].
In diabetes, the dysregulation of programmed cell death is a significant factor of cardiomyopathy. In human and animal models of diabetes, cardiac pathology is associated with excessive apoptosis [Citation5,Citation6] that progressively leads to histopathological features and eventually to heart disease [Citation7,Citation8]. Overwhelming evidence indicates that cardiomyocyte apoptosis in diabetes is associated with cytopathological features [Citation9,Citation10], which is likely a direct result of hyperglycemia-triggered caspase 3 activation [Citation3,Citation11]. There are two pathways known to produce cardiac apoptosis, namely the mitochondrial and the death receptor-mediated pathways, and both are influenced by cellular antioxidants [Citation5]. Caspases 8 and 9 are initiator caspases for the extrinsic and intrinsic pathways, respectively, and caspase 3 is activated by both pathways and initiates apoptosis. The early event of myocyte apoptosis is characterized by nuclear damage, which is often undetectable morphologically, but DNA damage can be identified by several techniques, including the comet assay. The initial phase of the apoptotic process is followed by nuclear fragmentation and later by cytoplasmic alterations [Citation12,Citation13]. In diabetes, oxidative stress is the key element in all of mechanisms underlying cardiomyopathy and apoptosis, both of which are associated with hyperglycemia and hyperlipidemia [Citation3].
Beyond the beverage use of tea, naturally derived catechins have been recognized to have numerous health benefits. However, the molecular mechanisms through which these molecules mediate their effects remain largely unknown [Citation14,Citation15]. Green tea is obtained from the fresh leaves of the Camellia sinensis plant and is processed to prevent oxidation of the polyphenolic constituents [Citation16]. Experimental and clinical studies have confirmed the efficacy of phytochemicals in the modulation of oxidative stress-induced cardiovascular diseases associated with diabetes [Citation17,Citation18]. Catechins are flavanols that constitute the majority of soluble solids of green tea. EGCG [(−)-epigallocatechin-3-gallate] is the main catechin [Citation19] and ECG [(−)-epicatechin-3-gallate] is the second most concentrated catechin component of green tea. Other major catechins found in green tea include (−)-epicatechin (EC) and (−)-epigallocatechin (EGC) [Citation20]. Catechins afford an antioxidant effect by inducing antioxidant enzymes, inhibiting pro-oxidant enzymes and scavenging free radicals [Citation16]. The potential mechanisms underlying the antioxidant effects of tea as effective scavengers of physiologically relevant reactive oxygen and nitrogen species were reviewed previously. The aromatic phenol rings present in these molecules cause them to behave as antioxidants by reducing free radicals and chelating metals in the body [Citation21].
Catechins of green tea, particularly EGCG, have antioxidant activity [Citation22], antidiabetic activity and antiobesity [Citation23] as well as anti-inflammatory activities [Citation24,Citation25], and can modulate apoptotic processes in the liver [Citation26]. EGCG binds to low-density lipoproteins (LDL) and protects them from glycation and oxidation under high-glucose medium, which mimics diabetes. Oxidized LDL and soluble vascular cell adhesion molecule-1 are markedly decreased after 30 days of green tea ingestion [Citation27]. Oral treatment with green tea polyphenol offers cardioprotection against ischemia-reperfusion injury in an isolated rat heart model [Citation28]. Plyphenol-60 (PP-60) has a therapeutic effect on acne by suppressing inflammation, specifically by inhibiting IL-8 secretion [Citation29].
The beneficial effects of green tea are related to catechins, particularly EGCG [Citation30]. However, no previous study has investigated the efficacy of polyphenon-60, which contains pure catechins, mainly EGCG, in diabetes-induced cardiomyopathy in rats. In addition, the mechanisms involved in its beneficial effects are not fully understood. Therefore, the present study was designed to evaluate the cardio-ameliorative effects of polyphenon-60 in STZ-induced early cardiac injury in rats and attempts to understand the mechanism underlying the therapeutic effects of this compound with reference to biochemical and molecular markers.
2 Materials and methods
2.1 Chemicals
Green tea polyphenol (polyphenon-60) and streptozotocin (STZ) were purchased from Sigma (Sigma, St. Louis, MO, USA).
2.2 Experimental design and animal groups
Male Wistar rats weighing approximately 250–300 g were obtained from the Laboratory Animal Maintenance Unit, Faculty of Science, Mansoura University and acclimatized to the laboratory conditions for two weeks. They were maintained at 25 ± 2 °C and a 12-h light/12-h dark cycle. The rats were given standard commercial rat chow and water ad libitum. All of the experiments were conducted in accordance with protocols approved by the local ethical committee for in vivo animal experiments. The animals were divided into four groups of eight rats each. The first group served as a control. The second group received an oral dosage of polyphenon-60 (100 mg/kg body wt) every other day for a period of two weeks. The third group received a single i.p. injection of STZ (40 mg/kg body wt). The diabetic state of the rats was confirmed by determination of the blood glucose level after two days of STZ injection. The fourth group received STZ followed by green tea polyphenon-60 at the same doses as the second and third groups.
After two weeks of treatments, overnight fasted rats were anaesthetized with sodium pentobarbital (50 mg/kg i.p.), and their blood was collected by cardiac puncture. The sera were separated by centrifugation (500× g) for 5 min for biochemical determinations. The heart samples were homogenized in 10 volumes of 50 mM sodium phosphate buffer (pH 7.4) at 4 °C for 30 s. The homogenate was centrifuged (1000× g) for 5 min in a refrigerated centrifuge. The resulting supernatant was used for the biochemical determinations.
2.3 Biochemical analysis
The blood glucose, insulin and HbA1c levels were estimated using kits supplied by Spinreact (St. Esteve d'en Bas Girona, Spain), Abcam (Cambridge, MA, USA) and BioSystems (Barcelona, Spain), respectively. The profile of the serum lipids, including total lipids, triglyceride, total cholesterol, high-density lipoprotein (HDL), low-density lipoprotein (LDL), very low-density lipoproteins (VLDL), was assayed following the kit's instructions (Spinreact, St. Esteve d'en Bas Girona, Spain). The heart function was estimated by determining the creatine kinase (CK-MB) and lactic dehydrogenase (LDH) activities using kits purchased from Elitech (Puteaux, France). The lipid peroxidation was estimated by measuring the amount of malondialdehyde (MDA). The hydrogen peroxide (H2O2) concentrations were determined following the kit's instructions (Dokki-Giza, Egypt). The antioxidant levels were estimated by determining superoxide dismutase (SOD) activity, catalase (CAT) activity, and glutathione (GSH) content using kits supplied by Biodiagnostic (Dokki-Giza, Egypt). The pro-inflammatory markers interleukin 6 (IL-6), interleukin 1 beta (IL-1b) and tumor necrosis factor alpha (TNF-α) were estimated according to kits purchased from R&D Systems (NE Minneapolis, MN, USA) and EBioscience (San Diego, CA, USA). The protein concentrations were determined as previously described [Citation31].
2.4 Molecular analysis
Heart samples were prepared for flow cytometry as previously described [Citation32]. The cells obtained were suspended in PBS with BSA, divided into aliquots collected in round-bottom tubes (Becton Dickinson) and stored at 4 °C for flow cytometric analyses. The flow cytometric analyses were performed on a FACSCalibur™ cytometer (BD Biosciences, San Jose, CA, USA) using the CellQuest Pro software (Becton Dickinson) for data acquisition and analysis [Citation33].
2.5 Flow cytometric analysis of apoptosis with Annexin V–FITC/PI staining
Apoptosis in the heart samples was probed using fluorescein isothiocyanate-conjugated annexin V/PI with the ApoAlert kit from Clontech (Palo Alto, CA, USA) according to the manufacturer's instructions. The flow cytometric analyses were performed on a FACSCalibur™ cytometer (BD Biosciences, San Jose, CA, USA) using the CellQuest Pro software (Becton Dickinson) for data acquisition and analysis [Citation33].
2.6 Flow cytometric analysis of Bcl-2 and Bax
Heart samples were prepared with PBS/BSA buffer and then incubated with anti-Bcl-2 [100/D5] antibody (ab692) or anti-Bax [6A7] antibody (ab5714) for 15 min at room temperature. The cells were then resuspended in 0.5% paraformaldehyde in PBS/BSA and analyzed by flow cytometry.
2.7 Flow cytometric analysis of P53
The cell suspensions were prepared with a PBS/BSA buffer, incubated with antibody (mouse anti-P53 “aa20-25” FITC, Clone: DO-1), mixed well and incubated for 30 min at room temperature. The cells were washed with PBA/BSA and centrifuged at 400× g for 5 min, and the supernatant was discarded. The cells were then resuspended in 0.5% paraformaldehyde in PBS/BSA and analyzed using a flow cytometer [Citation34].
2.8 Flow cytometric analysis of CD95
The cell suspensions were prepared with a PBS/BSA buffer, incubated with antibody (monoclonal anti-Fas (CD95/Apo-1) antibody), mixed well and incubated for 30 min at room temperature. The cells were washed with BD Perm/Wash (BD Bioscience) and centrifuged at 400× g for 5 min, and the supernatant was discarded. The cells were then resuspended in BD Perm/Wash and analyzed by flow cytometry.
2.9 Flow cytometric analysis of caspases 3, 8 and 9
The cell suspensions were prepared with a PBS/BSA buffer, incubated with antibody FITC rabbit anti-active caspase-3 antibody (CPP32; Yama; Apopain, BD Bioscience); anti-caspase-8 (E6) antibody (Abcam); or rabbit monoclonal anti-caspase 9 (E23) antibody (ab32539), mixed well and incubated for 30 min at room temperature. The cells were washed with BD Perm/Wash (BD Bioscience) and centrifuged at 400× g for 5 min, and the supernatant was discarded. The cells were then resuspended in BD Perm/Wash and analyzed by flow cytometry.
2.10 Single-cell gel electrophoresis (comet assay)
The DNA damage in the heart samples was assessed using the single-cell gel electrophoresis (comet assay) method, a well-validated technique developed for measuring DNA strand breaks in individual cells, as described previously [Citation35,Citation36]. The quantification of the DNA from the obtained images was performed with the CASP software, which allows the direct determination of the percentage of DNA in the tail.
2.11 Statistical analysis
The data are presented as the means ± S.D. The statistical analyses were performed by one-way ANOVA followed by Students Newman–Keuls post-hoc test. Statistical significance was considered as P < 0.05.
3 Results
The effect of polyphenon-60 on blood glucose and body weight in the different animal groups was studied during the experimental period. The body weight of the diabetic rats was gradually decreased during the experimental period compared with that of the control group. The treatment of the diabetic rats with PP-60 (100 mg/kg) resulted in a marked amelioration along the experimental period compared with the diabetic group (Fig. 1a). The blood glucose of the diabetic rats was significantly increased during the experimental period compared with that of the control group. The treatment of the diabetic rats with PP-60 resulted in a marked amelioration along the experimental period compared with the diabetic group (Fig. 1b), but the level was significantly lower than that of the control group.
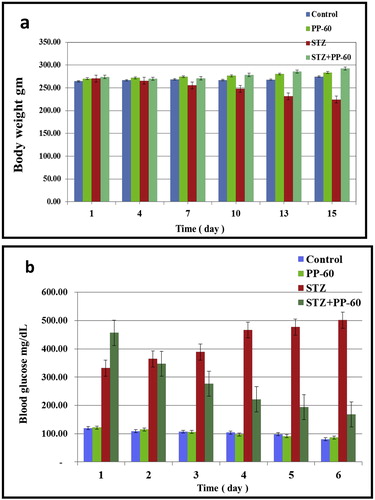
STZ treatment produced a significant elevation in the serum glucose and HbA1c levels two weeks after injection (Fig. 2a and b). These diabetic rats had significantly higher HOMA-IR levels and secreted significantly less insulin than the control group (Fig. 2c and d). The oral treatment of the diabetic rats with PP-60 showed a significant effect on the serum glucose and HbA1c levels and presented significantly higher insulin and HOMA-IR values compared with that of the diabetic rats.
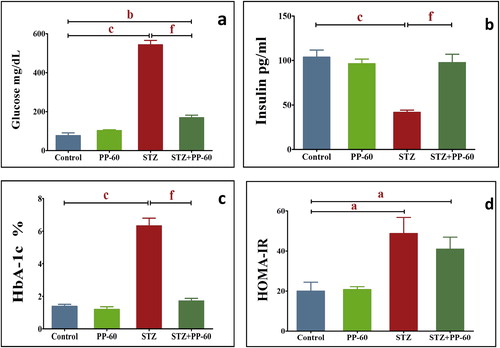
Compared with the control group, the lipid profile, including the levels of total lipids, triglycerides, total cholesterol, low-density lipoprotein and very-low lipoprotein, was significantly higher, whereas the level of high-density lipoprotein was significantly lower in the diabetic rats (). The treatment of the diabetic rats with PP-60 caused marked amelioration of the lipid fractions toward the control levels compared with the diabetic group ().
Table 1 Effect of polyphenon-60 on the serum lipid profile (total lipids (TL), cholesterol (Ch), triglycerides (TG), high-density lipoprotein (HDL), low-density lipoprotein (LDL), and very-low-density lipoprotein (VLDL)) expressed as mg/dl in the different animal groups.
The diabetic rats showed significantly higher activities of CK-MB and LDH in the serum compared with the control values. The oral treatment with PP-60 after diabetic induction normalized the increase in the activities of these enzymes and demonstrated insignificant changes compared with those of the control group (Fig. 3).
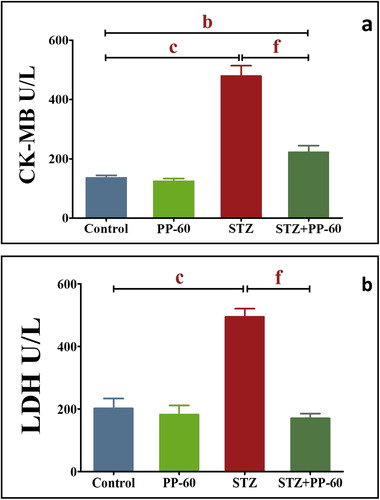
A significant increase in lipid peroxidation was demonstrated in the heart of the diabetic rats, as indicated by the increase in the MDA and H2O2 concentrations after STZ treatment (). A marked control of lipid peroxidation and generation of H2O2 in the heart against the diabetic-induced increase in the MDA level and generation of H2O2 by the oral administration of PP-60 after STZ treatment was demonstrated. The cardiac content of GSH and activities of SOD and CAT significantly decreased after diabetic induction by STZ. This effect was significantly prevented in the heart by the oral administration of PP-60 into the diabetic rats.
Table 2 Effect of polyphenon-60 on the levels of the lipid peroxidation product MDA (nmol/mg protein), hydrogen peroxide (H2O2), glutathione (GSH; mg/g protein) superoxide dismutase (SOD; U/mg protein) and catalase (CAT; U/mg protein) in the heart in the different animal groups.
The degree of apoptosis in the heart was assessed through flow cytometric analysis with Annexin V-FITC staining (Fig. 4). The data demonstrate that the percentage of apoptotic cells was significantly higher in the heart of the diabetic rats compared with the controls (Fig. 4a). Treatment with PP-60 significantly minimized the number of apoptotic cells compared with the diabetic rats. In addition, DC95 was significantly increased in the heart of diabetic rats compared with the controls (Fig. 4b). The effects of PP-60 and diabetes on specific apoptotic proteins were assessed. The flow cytometric analysis demonstrated that the anti-apoptotic protein Bcl-2 was significantly decreased and that the apoptotic protein Bax was increased in the heart of diabetic rats and that treatment with PP-60 markedly normalized the changes in the expression of these proteins (Fig. 4c and d). The expression of caspases 9, 8 and 3 was strongly detected in the heart of the diabetic rats, and the treatment with PP-60 significantly inhibited the diabetes-induced activation of these apoptotic proteins (Fig. 5a–c).
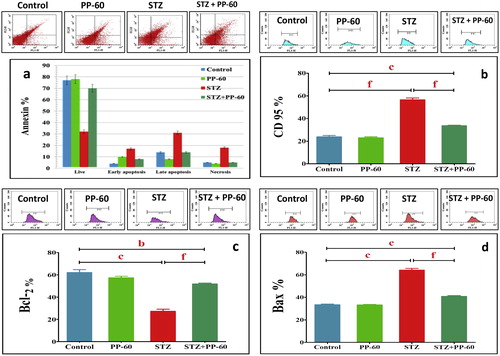
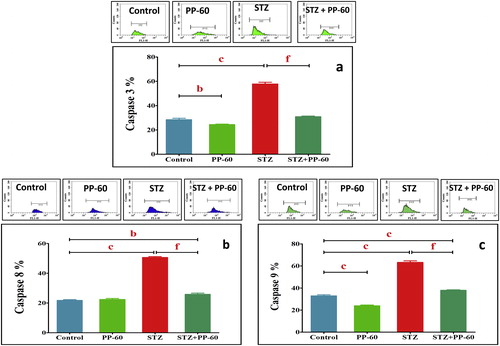
The diabetic rats had significantly higher levels of IL-1b, IL-6, and TNF-α compared with the control values. The oral treatment with PP-60 after diabetic induction normalized the increase in the levels of these cytokines in the serum and displayed insignificant changes compared with those of the control (Fig. 6a–Fig. 6c).
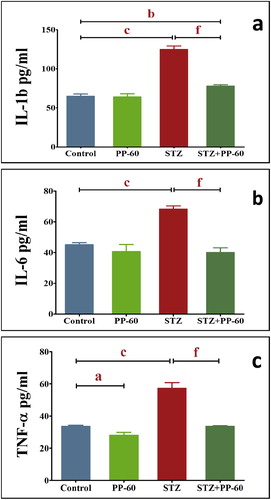
The rats in both the control and PP-60-treated groups had lower levels of both the percentage of p53 and tail DNA in the hearts compared with the diabetic rats, with the latter appearing to be more severe than the former (Fig. 7a), suggesting oxidative DNA damage in the diabetic rats. The treatment of diabetic rats with PP-60 significantly ameliorated both the percentage of tail DNA and p53 in the heart compared with the diabetic rats (Fig. 7b).
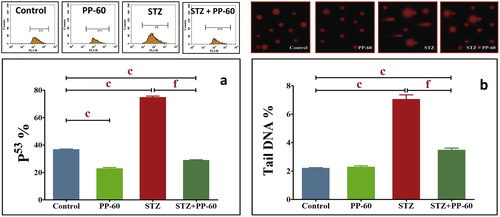
4 Discussion
There is increasing evidence of a protective effect of green tea catechins against cardiac disease. The present results demonstrate the mechanism underlying the antiapoptotic action of green tea PP-60 synchronized with amelioration of metabolic risk factors and oxidative stress as well as the modulation of proinflammatory cytokines in STZ-diabetic rats.
The results demonstrated a significant improvement in the levels of serum glucose and HbA1c accompanied with a marked amelioration of insulin release and HOMA-IR in the diabetic rats treated with PP-60. Previous studies have shown that green tea catechins inhibit intestinal glucose uptake by the sodium-dependent glucose transporter SGLT1 [Citation37], activates glucose uptake, and mimics insulin in that it decreases the expression of genes that control gluconeogenesis [Citation38]. Moreover, EGCG increases the tyrosine phosphorylation of the insulin receptor and insulin receptor substrate [Citation39]. Furthermore, the administration of 100 mg/kg PP-60 markedly improved the body weight loss in the diabetic rats in comparison with the diabetic group. This is likely due to the protective effect of green tea catechins in decreasing gluconeogenesis and regulating muscle loss [Citation40].
The present study showed that green tea PP-60 may increase the heart antiglycating activity produced by diabetes, as previously observed in aortic tissue [Citation41], and this finding was supported by a decrease in HB-A1c in the PP-60-treated diabetic rats (Fig. 2). This finding is supported by the present findings that PP-60 ameliorates the elevation of IL-1b, IL-6, and TNF-α in the sera of diabetic rates treated with PP-60. It has been suggested that the presence of the gallate group at position 3 plays an essential role in the protection against protein oxidation and glycation [Citation42].
In line with these findings, PP-60 significantly reduced HOMA-IR in STZ-diabetic rats (Fig. 2), supporting the finding that green tea catechin improves the insulin resistance of diabetic rats. Similarly, the HOMA-IR index has been found to increase when dogs become obese and decreases after green tea treatment [Citation43]. It is possible that green tea increases GLUT4 translocation in muscle cells [Citation43]. EGCG also inhibits dexamethasone-induced insulin resistance through the AMPK and PI3K/Akt pathways [Citation44]. This finding implies that PP-60 may protect against insulin sensitivity and improves glucose homeostasis. Previous studies have also shown that EGCG may increase insulin activity [Citation45,Citation46]. Thus, supplementation with PP-60 ameliorates risk factors, improves glucose homeostasis and may be beneficial to diabetes for preventing possible early cardiac events.
Hyperglycemia is not the only major factor affecting cardiac complications. Dyslipidemia has been reported to be particularly important for the development of cardiomyopathy [Citation6,Citation47]. Therefore, improvement of the lipid profile in serum is a feasible health strategy that considers the therapeutic potential of EGCG on cardiovascular disease. Both experimental and clinical studies have suggested that EGCG may have such an effect [Citation46,Citation48]. The administration of PP-60 to the diabetic rats significantly lowered the serum levels of total cholesterol, triglycerides, and low-density and very-low-density lipoproteins and resulted in a significantly higher serum level of high-density lipoprotein compared with the diabetic rats. Previous studies have shown that catechin extracted from green tea improves the serum lipid profile in rats fed an atherogenic diet [Citation49] and injected with STZ [Citation50] and in obese patients with metabolic syndrome [Citation51]. A recent study showed that the tea polyphenol EGCG actually binds to lipoprotein particles and facilitates the LDL antioxidant and anti-glycation activities in a high glucose environment. Lowering the levels of serum lipid risk factors through dietary polyphenol treatment appears to be the main scheme for decreasing the threat of cardiac disease and related complications [Citation52,Citation53]. These results indicate that EGCG is the major component of polyphrnol-60 that has a beneficial effect for protecting against risk of cardiac disease by improving the lipid levels. Thus, EGCG is a primary agent in LDL-lowering therapy for the reduction of cardiac risk.
Concomitant with the disturbed metabolic risk factors, increased events of oxidative stress were found in the heart of the diabetic rats. Treatment with PP-60 evidently prevented the diabetes-induced decrease in SOD and CAT activities and MDA formation, which culminated into a marked amelioration of oxidative stress in the heart tissue. Oxidative stress leads to the accumulation of the lipid peroxidation product MDA in the heart and causes impaired cell function. Mounting evidence strongly indicates the potency of the free-radical-scavenging abilities of catechins due to the presence of the C-ring gallate group. Galloylated catechins, including PP-60, are strong antioxidants due to their higher phospholipid/water partition coefficients and hence have the protective properties of the membrane phospholipids [Citation54]. Green tea extract can decrease lipid peroxidation markers, including lipid hydroperoxides, 4-hydroxynonenal and malondialdehyde, in the liver, serum and brain [Citation55]. These results confirm the ability of PP-60 to attenuate oxidative stress in the heart.
Marked increased in the serum CK-MB and LDH activities were demonstrated in the diabetic group, suggesting the incidence of significant injury in the membrane of cardiomyocytes, likely due to lipid peroxidation, and the leaking of the functional marker enzymes into the blood. After PP-60 treatment, the diabetic rats presented significant reductions in the elevated activities of CK-MB and LDH, demonstrating the efficiency of green tea catechin for the protection of cardiomyocyte integrity. This finding agrees with the ability of EGCG to significantly decrease reactive oxygen species (ROS) and cytosolic Ca2+ and to prevent alterations in the protein expression of the adherens molecules β-catenin and N-cadherin and the gap junction protein connexin 43 in cardiac cells [Citation56]. Green tea significantly reduces the serum biomarker levels, increases the antioxidants levels in cardiac tissue and restores electrocardiographic changes compared with the isoproterenol-only-treated group [Citation57] as well as reduces cyclophosphamide-induced myocardial toxicity [Citation58]. These data clearly show that PP-60 is effective against cardiac stress.
The upregulation of the pro-inflammatory cytokine TNF-α, which increases after increases in ROS and oxidative stress, has emerged as a significant contributor to myocardial heart failure [Citation59,Citation60]. The effect of hyperglycemia-induced oxidative stress on inflammation has been documented by numerous studies [Citation61,Citation62]. A previous study found that the levels of IL-6, TNF-α and IL-18 were increased significantly in patients with impaired glucose tolerance [Citation63,Citation64]. Therefore, it has been hypothesized that polyphenols can modulate cellular signaling processes during inflammation due to their antioxidant properties. It has been reported that green tea extract reduces the serum levels of TNF-alpha, insulin, and free fatty acid and decreases the mRNA expression level of TNF-alpha, interleukin (IL)-6, IL-1β, and IL-18 in the liver of obese mice [Citation65] and prevents cardiac fibrosis by attenuating TNF-α [Citation66]. TNF-α is critical for the induction of the inflammatory response. Experimentally induced diabetes mellitus is characterized by intramyocardial inflammation, including increased TNF-α expression and myocardial fibrosis [Citation67]. Hyperglycemia can produce ROS, which in turn triggers TNF-a gene expression, and treatment with the ROS scavenger N-acetylcysteine significantly reduces the hyperglycemia-induced TNF-α [Citation68]. Our experimental results are consistent with those of previous studies, which have revealed that the circulating IL-1b, IL-6, and TNF-α levels are elevated in STZ-induced diabetic rats [Citation67], and agree with the results of a study that showed that EGCG reduces the expression of IL-6 and TNFα in the heat of an I/R injury model by decreasing NFκB [Citation69]. We further observed that PP-60 suppresses the elevated level of these cytokines. In conjunction with the hypoglycemic and antioxidant activities of tea catechins, the results imply that PP-60 may reduce pro-inflammatory cytokines by modulating the glucose levels and oxidative stress in the heart of diabetic rats.
To investigate the mechanism underlying the action of PP-60 on cardiac apoptosis in diabetes, we determined the levels of apoptosis and related regulatory proteins in the heart of STZ-induced diabetic rats before and after PP-60 treatment. In the diabetic group, the numbers of both apoptotic and necrotic cells were increased significantly compared with the control group, whereas PP-60 markedly reduced the numbers of these cells. In addition, PP-60 normalized the levels of CD95, caspases 8, 9, and 3 and Bax in the same group. These results indicate a marked modulation of both extrinsic and intrinsic apoptotic pathways in the heart of PP-60-treated diabetic rats and provide evidence that EGCG has the ability to modulate apoptotic pathways in diabetes. EGCG protects against subsequent apoptosis following I/R injury in cardiac surgery by regulating the Bcl-2/Bax ratio and blocking the cleavage of caspase 3 [Citation28]. Similarly, EGCG increases the expression levels of Bcl-2, Mn-superoxide dismutase, and catalase, decreases the levels of Bax and increases the ratio of Bcl-2/Bax in isolated rat hearts [Citation70]. In addition, EGCG modulates apoptosis by preventing the expression of the proapoptotic genes Bax, Bad and Mdm2 while inducing the anti-apoptotic genes Bcl-2, Bcl-w and Bcl-xL in SH-SY5Y cells [Citation71]. These results show that EGCG, which is the most abundant antioxidant catechin in PP-60, exerts a protective effect on cardiac injury by acting as an antioxidant and anti-apoptotic agent.
The present results further show that the mechanism may be related to the inhibitory effects of PP-60 on p53 induction and DNA damage. A previous study demonstrated that p53 plays an important role in cardiomyocyte apoptosis in cardiac hypertrophy because the upregulation of the p53 and its dependent genes is important in the modulation of myocyte apoptosis in heart failure [Citation71]. The present results demonstrated obvious DNA damage with a markedly high fraction of tail DNA and typical comet-like nuclei, which are indicative of increased DNA damage in the hearts of diabetic rats. Thus, apoptosis results in nuclear fragmentation via the action of caspases as well as pro-inflammatory cytokine release.
The oral treatment of diabetic rats with PP-60 inhibits the increase in the comet parameter compared with diabetic rats. The mechanism of protection may be attributed to the effective antioxidant and scavenging free radical potential of catechin. These data confirm recent reports that EGCG markedly protects against DNA damage and oxidative stress in different cells and tissues, including human leucocytes [Citation72], human mucosa cell cultures [Citation73] and liver cells [Citation74]. PP-60 treatment effectively upregulates Bcl-2, downregulates p53 and protects against DNA damage. Hyperglycemia activates p53 and p53-regulated genes leading to myocyte cell death [Citation13]. It has been reported that p53 can act to regulate the intracellular redox state and induces apoptosis by a pathway dependent on ROS production [Citation71,Citation75]. In contrast, Bcl-2 exerts anti-apoptotic and anti-necrotic effects through its antioxidant influence on intracellular ROS [Citation6,Citation76]. Bcl-2 may decrease lipid peroxidation by enhancing cell resistance to ROS and preventing ROS production [Citation71]. Therefore, we propose that the anti-apoptotic effect of PP-60 by decreasing p53 induction and the decrease in Bcl-2 may be related, at least in part, to its antioxidant impact. It appears that PP-60 decreases apoptosis by blocking DNA damage via the action of caspases and pro-inflammatory cytokine suppression.
5 Conclusions
The modulation of pro-inflammatory and pro-apoptotic pathways by green tea catechins in the diabetic heart may be an additional pathophysiological mechanism that prevents further consequences of the diabetic heart and beneficially prevents progression of the complication. It appears that the pleiotropic effects of polyphenon-60, including its anti-hyperglycemic effect, its influence of hypolipidemia, its attenuation of the oxidative stress reduction induced by circulating pro-inflammation cytokines and its anti-apoptotic action through the modulation of its related regulated proteins, contribute to and accompany the beneficial effects of treatment with PP-60 on diabetic heart dysfunction.
Acknowledgments
The facilities provided by Faculty of Science, Mansoura University, Egypt, are greatly acknowledged and appreciated.
Notes
Peer review under responsibility of Mansoura University.
References
- J.M.ForbesM.E.CooperMechanisms of diabetic complicationsPhysiol Rev9312013137188
- M.BosC.AgyemangPrevalence and complications of diabetes mellitus in Northern Africa, a systematic reviewBMC Public Health132013387
- K.HuynhB.C.BernardoJ.R.McMullenR.H.RitchieDiabetic cardiomyopathy: mechanisms and new treatment strategies targeting antioxidant signaling pathwaysPharmacol Ther14232014375415
- M.A.El-MissiryA.I.OthmanM.A.Al-AbdanA.A.El-SayedMelatonin ameliorates oxidative stress, modulates death receptor pathway proteins, and protects the rat cerebrum against bisphenol-A-induced apoptosisJ Neurol Sci3471–22014251256
- S.GhoshD.QiD.AnT.PulinilkunnilA.AbrahanK.H.Kuoet alCardiomyocyte apoptosis induced by short-term diabetes requires mitochondrial GSH depletionAm J Physiol Heart Circ Physiol28922005H768H776
- A.H.AminM.A.El-MissiryA.I.OthmanMelatonin ameliorates metabolic risk factors, modulates apoptotic proteins, and protects the rat heart against diabetes-induced apoptosisEur J Pharmacol747C2014166173
- S.GhoshS.TingH.LauT.PulinilkunnilD.AnD.Qiet alIncreased efflux of glutathione conjugate in acutely diabetic cardiomyocytesCan J Physiol Pharmacol82102004879887
- S.GhoshT.PulinilkunnilG.YuenG.KewalramaniD.AnD.Qiet alBrief episode of STZ-induced hyperglycemia produces cardiac abnormalities in rats fed a diet rich in n-6 PUFAAm J Physiol Heart Circ Physiol28762004H2518H2527
- K.HuynhH.KiriazisX.J.DuJ.E.LoveS.P.GrayK.A.Jandeleit-Dahmet alTargeting the upregulation of reactive oxygen species subsequent to hyperglycemia prevents type 1 diabetic cardiomyopathy in miceFree Radic Biol Med602013307317
- Y.S.YoonS.UchidaO.MasuoM.CejnaJ.S.ParkH.C.Gwonet alProgressive attenuation of myocardial vascular endothelial growth factor expression is a seminal event in diabetic cardiomyopathy: restoration of microvascular homeostasis and recovery of cardiac function in diabetic cardiomyopathy after replenishment of local vascular endothelial growth factorCirculation11116200520732085
- L.CaiW.LiG.WangL.GuoY.JiangY.J.KangHyperglycemia-induced apoptosis in mouse myocardium: mitochondrial cytochrome C-mediated caspase-3 activation pathwayDiabetes51200219381948
- Z.DarzynkiewiczG.JuanX.LiW.GorczycaT.MurakamiF.TraganosCytometry in cell necrobiology: analysis of apoptosis and accidental cell death (necrosis)Cytometry2711997120
- F.FiordalisoA.LeriD.CesselliF.LimanaB.SafaiB.Nadal-Ginardet alHyperglycemia activates p53 and p53-regulated genes leading to myocyte cell deathDiabetes5010200123632375
- A.KatzM.EfrosJ.KaminetskyK.HerrlingerD.ChirouzesM.CeddiaA green and black tea extract benefits urological health in men with lower urinary tract symptomsTher Adv Urol6320148996
- S.GaurR.AgnihotriGreen tea: a novel functional food for the oral health of older adultsGeriatr Gerontol Int1422014238250
- P.BhardwajD.KhannaGreen tea catechins: defensive role in cardiovascular disordersChin J Nat Med112013345353
- A.SingalS.KaurN.TirkeyK.ChopraGreen tea extract and catechin ameliorate chronic fatigue-induced oxidative stress in miceJ Med Food8120054752
- A.M.El-MowafyM.M.Al-GayyarH.A.SalemM.E.El-MeseryM.M.DarweishNovel chemotherapeutic and renal protective effects for the green tea (EGCG): role of oxidative stress and inflammatory-cytokine signalingPhytomedicine17201010671075
- N.KhanF.AfaqM.SaleemN.AhmadH.MukhtarTargeting multiple signaling pathways by green tea polyphenol (-)-epigallocatechin-3-gallateCancer Res66200625002505
- S.ValcicB.N.TimmermannD.S.AlbertsG.A.WachterM.KrutzschJ.Wymeret alInhibitory effect of six green tea catechins and caffeine on the growth of four selected human tumor cell linesAnticancer Drugs741996461468
- B.FreiJ.V.HigdonAntioxidant activity of tea polyphenols in vivo: evidence from animal studiesJ Nutr1331020033275S3284S
- B.N.SinghS.ShankarR.K.SrivastavaGreen tea catechin, epigallocatechin-3-gallate (EGCG): mechanisms, perspectives and clinical applicationsBiochem Pharmacol8212201118071821
- T.M.RainsS.AgarwalK.C.MakiAntiobesity effects of green tea catechins: a mechanistic reviewJ Nutr Biochem221201117
- R.SinghN.AkhtarT.M.HaqqiGreen tea polyphenol epigallocatechin-3-gallate: inflammation and arthritisLife Sci8625–262010907918
- S.RiegseckerD.WiczynskiM.J.KaplanS.AhmedPotential benefits of green tea polyphenol EGCG in the prevention and treatment of vascular inflammation in rheumatoid arthritisLife Sci9382013307312
- H.A.El-BeshbishyO.M.TorkM.F.El-BabM.A.AutifiAntioxidant and antiapoptotic effects of green tea polyphenols against azathioprine-induced liver injury in ratsPathophysiology1822011125135
- H.SungW.K.MinW.LeeS.ChunH.ParkY.W.Leeet alThe effects of green tea ingestion over four weeks on atherosclerotic markersAnn Clin Biochem4242005292297
- S.YanagiK.MatsumuraA.MaruiM.MorishimaS.H.HyonT.Ikedaet alOral pretreatment with a green tea polyphenol for cardioprotection against ischemia-reperfusion injury in an isolated rat heart modelJ Thorac Cardiovasc Surg14122011511517
- M.K.JungS.HaJ.A.SonJ.H.SongY.HouhE.Choet alPolyphenon-60 displays a therapeutic effect on acne by suppression of TLR2 and IL-8 expression via down-regulating the ERK1/2 pathwayArch Dermatol Res30482012655663
- J.D.LambertR.J.EliasThe antioxidant and pro-oxidant activities of green tea polyphenols: a role in cancer preventionArch Biochem Biophys501120106572
- O.H.LowryN.J.RosebroughA.L.FarrR.J.RandallProtein measurement with the Folin phenol reagentJ Biol Chem19311951265275
- J.GongL.QianX.KongR.YangL.ZhouY.Shenget alCardiomyocyte apoptosis in the right auricle of patients with ostium secundum atrial septal defect diseasesLife Sci8012200711431151
- W.S.JuanH.W.LinY.H.ChenH.Y.ChenY.C.HungS.H.Taiet alOptimal Percoll concentration facilitates flow cytometric analysis for annexin V/propidium iodine-stained ischemic brain tissuesCytom Part A812012400408
- B.A.BonsingW.E.CorverM.C.GorsiraM.van VlietP.S.OudC.J.Cornelisseet alSpecificity of seven monoclonal antibodies against p53 evaluated with Western blotting, immunohistochemistry, confocal laser scanning microscopy, and flow cytometryCytometry2819971124
- N.P.SinghM.T.McCoyR.R.TiceE.L.SchneiderA simple technique for quantitation of low levels of DNA damage in individual cellsExp Cell Res17511988184191
- R.R.TiceP.W.AndrewsN.P.SinghThe single cell gel assay: a sensitive technique for evaluating intercellular differences in DNA damage and repairBasic Life Sci531990291301
- Y.KobayashiM.SuzukiH.SatsuS.AraiY.HaraK.Suzukiet alGreen tea polyphenols inhibit the sodium-dependent glucose transporter of intestinal epithelial cells by a competitive mechanismJ Agric Food Chem4811200056185623
- M.E.Waltner-LawX.L.WangB.K.LawR.K.HallM.NawanoD.K.GrannerEpigallocatechin gallate, a constituent of green tea, represses hepatic glucose productionJ Biol Chem2773820023493334940
- H.AshidaT.FuruyashikiH.NagayasuH.BesshoH.SakakibaraT.Hashimotoet alAnti-obesity actions of green tea: possible involvements in modulation of the glucose uptake system and suppression of the adipogenesis-related transcription factorsBiofactors222004135140
- A.ShirwaikarK.RajendranC.Dinesh KumarR.BodlaAntidiabetic activity of aqueous leaf extract of Annona squamosa in streptozotocin-nicotinamide type 2 diabetic ratsJ Ethnopharmacol9112004171175
- P.V.BabuK.E.SabithaC.S.ShyamaladeviTherapeutic effect of green tea extract on advanced glycation and cross-linking of collagen in the aorta of streptozotocin diabetic ratsClin Exp Pharmacol Physiol332006351357
- T.NakagawaT.YokozawaK.TerasawaS.ShuL.R.JunejaProtective activity of green tea against free radical- and glucose-mediated protein damageJ Agric Food Chem508200224182422
- S.SerisierV.LerayW.PoudrouxT.MagotK.OuguerramP.NguyenEffects of green tea on insulin sensitivity, lipid profile and expression of PPARalpha and PPARgamma and their target genes in obese dogsBr J Nutr996200812081216
- Z.F.ZhangQ.LiJ.LiangX.Q.DaiY.DingJ.B.Wanget alEpigallocatechin-3-O-gallate (EGCG) protects the insulin sensitivity in rat L6 muscle cells exposed to dexamethasone conditionPhytomedicine17120101418
- H.S.MoonH.G.LeeY.J.ChoiT.G.KimC.S.ChoProposed mechanisms of (-)-epigallocatechin-3-gallate for anti-obesityChem Biol Interact167220078598
- L.Y.WuC.C.JuanL.T.HoY.P.HsuL.S.HwangEffect of green tea supplementation on insulin sensitivity in Sprague-Dawley ratsJ Agric Food Chem5232004643648
- J.PossF.CustodisC.WernerO.WeingartnerM.BohmU.LaufsCardiovascular disease and dyslipidemia: beyond LDLCurr Pharm Des1792011861870
- P.V.BabuK.E.SabithaC.S.ShyamaladeviGreen tea impedes dyslipidemia, lipid peroxidation, protein glycation and ameliorates Ca2+ -ATPase and Na+/K+ -ATPase activity in the heart of streptozotocin-diabetic ratsChem Biol Interact1622006157164
- E.RameshR.ElanchezhianM.SakthivelT.JayakumarR.S.Senthil KumarP.Geraldineet alEpigallocatechin gallate improves serum lipid profile and erythrocyte and cardiac tissue antioxidant parameters in Wistar rats fed an atherogenic dietFundam Clin Pharmacol2232008275284
- M.RoghaniT.BaluchnejadmojaradHypoglycemic and hypolipidemic effect and antioxidant activity of chronic epigallocatechin-gallate in streptozotocin-diabetic ratsPathophysiology17120105559
- A.BasuK.SanchezM.J.LeyvaM.WuN.M.BettsC.E.Astonet alGreen tea supplementation affects body weight, lipids, and lipid peroxidation in obese subjects with metabolic syndromeJ Am Coll Nutr2920103140
- M.A.El-MissiryA.M.El GindyAmelioration of alloxan induced diabetes mellitus and oxidative stress in rats by oil of Eruca sativa seedsAnn Nutr Metab443200097100
- M.A.El-MissiryA.I.OthmanM.A.AmerL-Arginine ameliorates oxidative stress in alloxan-induced experimental diabetes mellitusJ Appl Toxicol24220049397
- N.CaturlaE.Vera-SamperJ.VillalainC.R.MateoV.MicolThe relationship between the antioxidant and the antibacterial properties of galloylated catechins and the structure of phospholipid model membranesFree Radic Biol Med342003648662
- B.A.SutherlandR.M.RahmanI.AppletonMechanisms of action of green tea catechins, with a focus on ischemia-induced neurodegenerationJ Nutr Biochem1752006291306
- S.R.HsiehC.S.HsuC.H.LuW.C.ChenC.H.ChiuY.M.LiouEpigallocatechin-3-gallate-mediated cardioprotection by Akt/GSK-3beta/caveolin signalling in H9c2 rat cardiomyoblastsJ Biomed Sci20201386
- M.ChakrabortyJ.V.KamathPharmacodynamic interaction of green tea extract with hydrochlorothiazide against ischemia-reperfusion injury-induced myocardial infarctionJ Adv Pharm Technol Res532014134139
- M.ChakrabortyJ.V.KamathA.BhattacharjeePharmacodynamic interaction of green tea extract with hydrochlorothiazide against cyclophosphamide-induced myocardial damageToxicol Int2122014196202
- I.RahmanS.K.BiswasP.A.KirkhamRegulation of inflammation and redox signaling by dietary polyphenolsBiochem Pharmacol7211200614391452
- I.RahmanI.M.AdcockOxidative stress and redox regulation of lung inflammation in COPDEur Respir J2812006219242
- A.CerielloR.TestaAntioxidant anti-inflammatory treatment in type 2 diabetesDiabetes Care32Suppl. 22009S232S236
- F.PiarulliG.SartoreA.CerielloE.RagazziR.ReitanoL.Nollinoet alRelationship between glyco-oxidation, antioxidant status and microalbuminuria in type 2 diabetic patientsDiabetologia527200914191425
- S.KhuranaK.VenkataramanA.HollingsworthM.PicheT.C.TaiPolyphenols: benefits to the cardiovascular system in health and in agingNutrients510201337793827
- S.KhuranaM.PicheA.HollingsworthK.VenkataramanT.C.TaiOxidative stress and cardiovascular health: therapeutic potential of polyphenolsCan J Physiol Pharmacol9132013198212
- M.ShimizuH.SakaiY.ShirakamiY.YasudaM.KubotaD.Terakuraet alPreventive effects of (-)-epigallocatechin gallate on diethylnitrosamine-induced liver tumorigenesis in obese and diabetic C57BL/KsJ-db/db MiceCancer Prev Res432011396403
- S.H.CherngC.Y.HuangW.W.KuoS.E.LaiC.Y.TsengY.M.Linet alGABA tea prevents cardiac fibrosis by attenuating TNF-alpha and Fas/FasL-mediated apoptosis in streptozotocin-induced diabetic ratsFood Chem Toxicol6520149096
- D.WestermannS.Van LinthoutS.DhayatN.DhayatA.SchmidtM.Noutsiaset alTumor necrosis factor-alpha antagonism protects from myocardial inflammation and fibrosis in experimental diabetic cardiomyopathyBasic Res Cardiol10262007500507
- Y.QuanC.T.JiangB.XueS.G.ZhuX.WangHigh glucose stimulates TNFalpha and MCP-1 expression in rat microglia via ROS and NF-kappaB pathwaysActa Pharmacol Sin3222011188193
- R.AnejaP.W.HakeT.J.BurroughsA.G.DenenbergH.R.WongB.ZingarelliEpigallocatechin, a green tea polyphenol, attenuates myocardial ischemia reperfusion injury in ratsMol Med1020045562
- C.S.PiaoD.S.KimK.C.HaH.R.KimH.J.ChaeS.W.ChaeThe protective effect of epigallocatechin-3 gallate on ischemia/reperfusion injury in isolated rat hearts: an ex vivo approachKorean J Physiol Pharmacol1552011259266
- R.ShengZ.L.GuM.L.XieW.X.ZhouC.Y.GuoEGCG inhibits cardiomyocyte apoptosis in pressure overload-induced cardiac hypertrophy and protects cardiomyocytes from oxidative stress in ratsActa Pharmacol Sin2822007191201
- D.PandirProtective effect of (-)-epigallocatechin-3-gallate on capsaicin-induced DNA damage and oxidative stress in human erythrocyes and leucocytes in vitroCytotechnology6722014367377
- P.BaumeisterM.ReiterS.ZiegerC.MatthiasU.HarreusDNA-protective potential of polyphenols in human mucosa cell culturesHNO562008795798
- G.C.YenJ.W.JuC.H.WuModulation of tea and tea polyphenols on benzo(a)pyrene-induced DNA damage in Chang liver cellsFree Radic Res3822004193200
- D.CesselliI.JakoniukL.BarlucchiA.P.BeltramiT.H.HintzeB.Nadal-Ginardet alOxidative stress-mediated cardiac cell death is a major determinant of ventricular dysfunction and failure in dog dilated cardiomyopathyCirc Res8932001279286
- A.J.KowaltowskiG.FiskumRedox mechanisms of cytoprotection by Bcl-2Antioxid Redox Signal73–42005508514