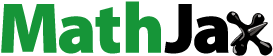
Abstract
Rapidly synthesized phyto-mediated silver nanoparticles (Ag NPs) using Artemisia nilagirica aqueous leaf filtrate has been confirmed through UV–visible spectrophotometer. The synthesized Ag NPs were further characterized using Fourier transform infra-red (FTIR), X-ray diffraction analysis (XRD) to determine the present of functional groups and average particle size (6.723 nm with cubic nature), respectively. Spherical shape (≤30 nm) of Ag NPs was confirmed by scanning electron microscopy (SEM). Bio-efficacy of these nanoparticles showed larvicidal and pupicidal properties than the aqueous leaf extract treatment alone against developmental stages (I–IV instars and pupa) of malaria vector Anopheles stephensi and dengue vector Aedes aegypti at 0.25% concentration level. The LC50 (LCL:UCL at 95% confidence limit) values of I–IV instar and pupa of An. stephensi were recorded at 0.343 (0.261:0.405), 0.169 (0.025:0.263), 0.198 (0.105:0.265), 0.141 (0.045:0.205) and 0.050 (0.606:0.224) % respectively and for Ae. aegypti (I–IV instar and pupa) 0.460 (0.364:0.537), 0.352 (0.239:0.432), 0.331 (0.833:0.549), 0.217 (0.228:0.378) and 0.161 (0.630:0.356) % were observed, after 24 h exposure. The first report of present investigation revealed that the rapid biological synthesis of silver nanoparticles using A. nilagirica leaf filtrate would be an effective potential alternative green larvicide for the control of mosquitoes at the developmental stages with eco-friendly approach.
1 Introduction
At present, mosquitoes are well known for their public health importance as vector for causing dreadful diseases such as malaria, chikungunya, dengue, Zika fever, yellow fever, lymphatic filariasis and Japanese encephalitis by transmitting host specific parasite Plasmodium falciparum and Wuchereria bancrofti by Anopheles stephensi and Culex quinquefasciatus respectively, and pathogens alphavirus and flavivirus by Aedes aegypti. Anopheles stephensi and Aedes aegypti are the primary vectors of malaria and dengue fever in tropical and subtropical regions, respectively. World Health Organization received summarized information from 106 malaria-endemic countries that about 3.3 billion people are at risk of malaria cases and 655,000 malaria deaths were reported [Citation1]. Recently, WHO estimated nearly 50–100 million dengue infections with 20,000 deaths occurring annually worldwide, 75% of which occurs in Asia Pacific Region [Citation2].
The spreading of these diseases by mosquito is mainly due to the ever increasing urbanization and associated anthropogenic activities. No effective drug or vaccine is available so far, but control of mosquito population in their breeding sites may directly contribute as an alternative source to prevent these mosquito-borne diseases [Citation3]. In this respect, different control strategies using synthetic insecticide Lufenuron (Match®) [Citation4], plant extract of Knema attenuata [Citation5], Acalypha alnifolia and microbial insecticide Metarhizium anisopliae [Citation6], essential oils [Citation7], and nereistoxin from marine annelid Lumbriconereis heteropoda [Citation8] have been reported against adult and developmental stages of Aedes, Anopheles and Culex. But these treatments exhibits its default like lower efficacy, development of resistance, chronic effects in non-target organisms and its long-term residual accumulation in the environment, thereby ecological imbalance through food chain and harm cattle and human beings. Hence, there is a constant need for developing biologically active larvicides from natural products which are biodegradable.
In this respect, the complex nature of environmentally hazardous synthetic chemicals, on reduction into micro or nano sized particles using bio-precursors possessed wide applications and has attracted considerable attention of many researchers to synthesis nanoparticles using plants like Cissus quadrangularis [Citation9], leaves of Cassia auriculata [Citation10], Euphorbia hirta and Nerium indicum [Citation11], Ipomoea aquatica, Enhydra fluctuans, Ludwigia adscendens [Citation12] and fruits of Carica papaya [Citation13], fungi Chrysosporium tropicum [Citation14], Cochliobolus lunatus [Citation15] and Puccinia graminis [Citation16], bacteria Ureibacillus thermosphaericus [Citation17] and Rhodococcus sp. [Citation18] to subsidise the use of physical and chemical methods, which require high cost, pressure, energy, temperature and chemical precursors that leads to toxic accumulation in environment.
Due to lack of data on the effect of synthesis of Ag NPs from weeds, the current study first preferred a weed plant with medicinal value, Artemisia nilagirica (C.B. Clarke) Pamp. () belongs to family Asteraceae, is an aromatic shrub, ovate leaves and commonly found in mountainous regions of India, was selected. It has been characterized for its antimicrobial [Citation19,Citation20] , anthelmintic, antiseptic, and larvicidal [Citation21] properties. Interestingly, this plant aqueous leaf filtrate mediated synthesis mechanism of Ag NPS were predicted and shown in .
The synthesis of bio-metal nanoparticles is a vital source of active area of academic and application research in many fields like industrial, pharmaceutical and medical, especially in diagnostics and therapeutics. Their diverse in vitro properties like, antioxidant [Citation22], antibacterial [Citation23], cytotoxic and antibiofilm efficacy [Citation24], inflammatory response and coagulation [Citation25], target drug delivery [Citation26], antiplasmodial activity [Citation27] and very recently mosquito larvicidal activity [Citation28] towards medically challenged pathogens and dreadful vectors like mosquito have been reported. Based on these analyses, the present report primarily focused on the synthesis, characterization and bio-efficacy of the Ag NPs synthesized from A. nilagirica aqueous leaf filtrate against developmental stages of An. stephensi and Ae. aegypti for the prevention of malaria and dengue.
2 Materials and methods
2.1 Vectors (An. stephensi and Ae. aegypti) collection and maintenance
Vectors used in this study were reared according to Sundaravadivelan et al. [Citation3]. Briefly, they were collected from National Centre for Disease Control (NCDC), Mettupalayam, Coimbatore, and maintained in enamel trays containing distilled water in the laboratory at room temperature.
2.2 Preparation of A. nilagirica aqueous leaf extract
Fresh and healthy leaves of A. nilagirica were collected nearby UPASI Tea Research Institute, Valparai, Coimbatore and identified by Prof. R. Gopalan, Department of Botany, Karpagam University, Coimbatore. The collected leaves were washed with running tap water to remove dust materials and rinsed in large volumes of deionized water and allowed to shade dry at room temperature (RT: 27 ± 2 °C). These dried leaves were powdered mechanically using electrical mixer and stored in airtight glass bottle for further use. One gram of leaves powder was dissolved in 100 mL of double distilled water and left for 5 h without disturbance, then filtered through Whatman filter paper (No. 1). The filtrate (=aqueous leaf extract) was considered as 1% stock solution. From this stock solution, concentrations were prepared ranging from 0.2, 0.4, 0.6, 0.8, and 1.0% for an effective bioassay.
2.3 Synthesis of silver nanoparticles (Ag NPs)
For the synthesis of Ag NPs, initially A. nilagirica aqueous leaf filtrate was prepared by using 10 g of fresh clean leaves; cut into very fine pieces and suspended in flask containing 100 mL of distilled water which was held in water bath at 60 °C for 60 min. The boiled extract was filtered through Whatman (No. 1) filter paper and this aqueous filtrate (A) was used for synthesis of Ag NPs. Freshly prepared 10 mL aqueous leaf filtrate was mixed with 90 mL of silver nitrate (AgNO3; HiMedia) solution (final concentration = 1 mM). This reaction mixture was held in dark at RT.
2.4 Characterization of Ag NPs
The synthesized Ag NPs were confirmed by visual observation due to color change in the reaction mixture. UV–vis spectrum (UV-2450 Shimadzu, Japan) was performed at different time intervals (30 min, 1, 2, 3 and 4 h) and the surface plasmon vibration was analyzed at a wavelength of 300–800 nm at a resolution of 1 nm. For further conformational characterization, the synthesized Ag NPs were pelleted by centrifugation (20,000×g, 30 min, 4 °C), and the obtained pellet was washed thrice using sterile deionized water. Finally, the pellet was dried and mixed with KBr for FTIR spectra cm−1 (IRAffinity-1, Shimadzu). Further, for X-ray diffraction analysis (XRD X’Pert PRO, PANalytical) the powder was coated an XRD grid and the spectra were recorded from 10° to 90° at 2θ angles. The obtained pattern was calculated using Scherrer’s equation to determine the size of Ag NPs. Morphology and shape of the particles were confirmed by SEM (FEI Quanta 200, Icon Analytical).
2.5 Effect of A. nilagirica aqueous leaf extract and Ag NPs on vectors
Larvicidal and pupicidal activity of A. nilagirica aqueous leaf extract and synthesized Ag NPs were performed against larvae (I–IV instar) and pupa of An. stephensi and Ae. aegypti, in accordance with World Health Organization standard protocols [Citation29] with slight modifications. For bioassay, different test concentrations of aqueous leaf extract (0.2, 0.4, 0.6, 0.8 and 1.0%) and synthesized Ag NPs (0.05, 0.10, 0.15, 0.20 and 0.25%) in 200 mL deionized water were prepared in autoclaved glass bottles. Twenty larvae of each instar and pupa were exposed to each concentration of aqueous leaf extract and Ag NPs separately. Five replicates were performed for each test concentration and each vector with controls run concurrently by substitution of distilled water against test solutions. No food was provided during the experiment. The number of dead larvae (I–IV instars) and pupa were counted after 24 h exposure. The percent mortality rates of different instars and pupa were determined using the formula given below:
2.6 Data analysis
The average crystallite domain size of synthesized nanoparticles was calculated using Scherrer’s formula (D = n λ/β Cos θ) where, D = average crystallite domain size, n = shape factor (0.94), λ = X-ray wavelength, β = Full width at half maximum (FWHM) and θ = diffraction angle. The average larvae and pupa mortality data were subjected to profit analysis for calculating LC50 and 95% confidence limit (upper and lower) and compared with Duncan’s multiple range tests to determine the differences between test substances and chi-square values using statistical analysis tool SPSS 9.0 version [Citation30].
3 Results
3.1 Visual analysis and microscan of Ag NPs
Biogenic Ag NPs were synthesized using 10% aqueous leaf filtrate of A. nilagirica within 60 min, bringing in change of color from pale yellow to brown in the reaction mixture (B). The absorption spectra were recorded as early as 30 min and thereafter at every 1 h interval up to 4 h (). A peak value at 463.50 nm with intensity of 1.839 was observed corresponding to Ag NPs.
3.2 FTIR analysis
Infra-red (IR) spectra of Ag NPs synthesized using A. nilagirica aqueous leaf filtrate after bioreduction of silver obtained by FTIR were recorded between 500 and 2000 cm−1 (). Troughs were observed at 1635.69 cm−1, 1381.08 cm−1, 1280.78 cm−1, 1118.75 cm−1, 1064.74 cm−1, 709.83 cm−1 and 609.53 cm−1, wherein, the signals at 1635.69 cm−1 and 1381.08 cm−1 are associated with the stretching vibrations at C=C and C–H in-plane bend (alkenes) respectively. The band at 1280.78 cm−1 is due to C–O stretch mode of carboxylic acids, 1118.75 cm−1, 1064.74 cm−1, 709.83 cm−1 and 609.53 cm−1 specifically associated with the stretching vibrations for C–C, C–O, CH2 and C–H bend responsible to functional groups like ketones, alcohols, alkenes and alkynes. The energy of these vibrations is sensitive to the secondary and tertiary structure of protein.
3.3 XRD and SEM analysis
On the basis of face-centered cubic structure, Bragg’s reflection is indexed by XRD of Ag NPs. The XRD pattern showed four intense peaks at 28.00°, 32.50°, 38.50° and 46.75° in the whole spectrum of 2θ values ranging from 10 to 90°. The diffraction features were in accord with the crystal data of silver and corresponded to (9 8), (1 0 1), (1 1 1) and (2 0 0) as per lattice plane index (). The mean size of Ag NPs was calculated using the Scherrer’s formula (D = n λ/β Cos θ), which was about 6.723 nm and monocrystalline in nature. Furthermore, SEM images showed the high-density of Ag NPs synthesized without aggregation by using A. nilagirica aqueous leaf filtrate () and their size was measured about ≤30 nm.
3.4 Larvicidal and pupicidal effect of Ag NPs
Larvicidal and pupicidal effect of synthesized Ag NPs were recorded against I–IV instar larvae and pupa of malaria vector An. stephensi and dengue vector Ae. aegypti. A cent percent mortality of third, fourth instar and pupa was observed against malaria vector An. stephensi treated with Ag NPs at a concentration of 0.25% and given in . On the other hand, only 92% and 96% mortality was observed with first and second instar at the same concentration, their LC50 values were found to determine the dose to cause 50% mortality in the treated larvae and pupa, where LC50 (LCL:UCL at 95% confidence limit) was noted in first instar 0.343 (0.261:0.405), second instar 0.169 (0.025:0.263), third instar 0.198 (0.105:0.265), fourth instar 0.141 (0.045:0.205) and pupa 0.050 (0.606:0.224). Mortality rates (%) and LC50 (LCL:UCL at 95% confidence limit) values of first, second, third, fourth instars and pupa were observed about 64%, 0.722 (0.653:0.806), 68%, 0.599 (0.485:0.714), 74%, 0.381 (0.222:0.484), 82%, 0.224 (0.003:0.348) and 88%, 0.066 (0.514:0.136) respectively while exposed to 1% aqueous leaf extract alone, which is the highest concentration in the treatment.
Table 1 Larvicidal and pupicidal activity of phyto-synthesized Ag NPs from Artemisia nilagirica aqueous leaf filtrate against Anopheles stephensi.
Similarly, Ag NPs treatment on larvae and pupa of Ae. aegypti resulted in 100% mortality of third, fourth instar and pupa followed by 80% in first and 88% in second instar were recorded (). The LC50 (LCL:UCL at 95% confidence limit) values of I–IV instar and pupa were recorded 0.460 (0.364:0.537), 0.352 (0.239:0.432), 0.331 (0.833:0.549), 0.217 (0.228:0.378) and 0.160 (0.630:0.356) % respectively, at 0.25% concentration which was the initial concentration of aqueous leaf extract treatment alone, where only 52% (LC50 = 0.167%) pupa mortality was observed after 24 h exposure. On the other hand, no mortality was observed in control. The chi-square value was significant at p < 0.05.
Table 2 Larvicidal and pupicidal activity of phyto-synthesized Ag NPs from Artemisia Nilagirica aqueous leaf filtrate against Aedes aegypti.
4 Discussion
Control of human vector populations have been studied by several researchers using botanicals which comprises of leaf extracts [Citation31], essential oils [Citation7], isolated compounds [Citation32] and recently, synthesized silver nanoparticles [Citation33]. In the present study the Ag NPs synthesized using aqueous leaf filtrate of A. nilagirica as a bioprecursor was investigated in two cases: characterization of the synthesized Ag NPs, and its bioactivity towards lethal effect against larvae and pupae of two vector species, An. stephensi and Ae. aegypti. The rapid color change from pale yellow to brown within 60 min in the reaction mixture strongly indicates the formation of biosilver due to effective reduction with active compounds and the color intensity for silver biometal recorded is due to excitation of surface Plasmon resonance [Citation34]. The different peaks of absorption spectra of phyto-reduced bio-silver (Ag+ → Ag0) recorded at different time intervals (30 min, 1, 2, 3 and 4 h), indicated the continuous involvement of phyto-constituents towards stable the NPs deliverable. Interestingly, the obtained broadened peak value during absorption exhibits the size, shape and its distribution of the Ag NPs in the reaction aqueous suspensions and observed Ag NPs could be stable with very little aggregation. The steadily increased surface Plasmon peak of Ag NPs with reaction time became saturated at 480 nm indicating complete reduction of the silver nitrate was observed with myco-synthesized Ag NPs [Citation14].
The FTIR spectra showed the formation of phyto-synthesized Ag NPs from proteins and water soluble active compounds of A. nilagirica aqueous leaf filtrates. The signals at 1635.69 cm−1, 1381.08 cm−1, 1280.78 cm−1, 1118.75 cm−1, 1064.74 cm−1, 709.83 cm−1 and 609.53 cm−1 showed evidence of bio-precursor being responsible for functional groups like alkenes, carboxylic acids, ketones, alcohols and alkynes in the reduction mixture, which has the strongest ability to bind with reduced non-ionic Ag metal for avoiding the agglomeration and holding stabilization in the medium. Udayasoorian et al. [Citation10] have also observed the possible functional groups like primary and secondary proteins, alcohols, ethers, carboxylic acids, esters and anhydrides existing responsible peaks at the region of 3400–1000 cm−1 using Cassia auriculata leaf extract. The polyols responsible peak at 1650 cm−1 for reduction of silver ions due to oxidation process by black tea leaf extracts was observed and it was predicted that polyphenols or flavonoids were the main metabolites/biomolecules for synthesis of silver and gold nanoparticles [Citation35]. It is also observed that presence of nitrate reductase in the reaction mixture enabled stable production of Ag NPs using P. tithymaloides stem extract [Citation36].
X-ray diffraction analysis performed to determine the nature and structure of synthesized Ag NPs by aqueous leaf filtrate showed four intense peaks in the spectrum of 2θ values between 25° and 50° corresponding to full width half maximum of Bragg’s reflection based on the face-centered-cubic (fcc) structure of Ag NPs. The average size of particles calculated (D = 6.723 nm) using Scherrer’s formula, clearly indicated the phyto-synthesized Ag NPs were crystalline in nature and could determine the monocrystallinity of particles formed in the reaction mixture [Citation37]. Moreover, few insignificant peaks were also recorded that ranged between 50° and 80°, which can be attributed to other organic molecules of culture supernatant.
With reference to Sintubin et al. [Citation38], the synthesized Ag NPs from plants, algae, yeasts, fungi and bacteria posses varied size and shapes. Similarly, the morphology of phyto-synthesized Ag NPs was documented using scanning electron microscopy wherein the particles were relatively spherical to irregular shape which indicates that the Ag NPs were distributed uniformly in the colloidal form.
The phyto-synthesized Ag NPs showed strong larvicidal and pupicidal activity against developmental stages (I–IV instar larvae and pupa) of An. stephensi and Ae. aegypti. In An. stephensi, pupa was highly susceptible to treatment with Ag NPs, which was confirmed by values of LC50 = 0.050% followed by fourth to first instar ranging from 0.141% to 0.343%. But there was a twofold increase in LC50 and the concentration ranged between 0.066% and 0.722% (Pupa – I instar) on treatment with aqueous leaf extract alone. In Ae. aegypti, higher rate of susceptible range with values of LC50 = 0.160% was observed with pupa and it linearly increased to 0.460% in first instar treated with Ag NPs. On the other hand, second instar required a higher dose at 0.701% to cause lethal effect when tested with aqueous leaf extract alone after 24 h exposure, which is a twofold increased concentration required to cause mortality in larvae compared to Ag NPs treatment. Based on these observations, we found that developmental stages of An. stephensi were more susceptible to Ag NPs derived from A. nilagirica aqueous leaf filtrate compared to Ae. aegypti. This is in accordance with our earlier report that Ae. aegypti is susceptible to synthesized Ag NPs (LC50:0.046–0.054%) using stem filtrate of Pedilanthus tithymaloides on developmental stages (I–IV instars and pupa) compared to aqueous stem extracts (LC50:1.529–1.455%) alone [Citation36]. Similar findings were observed with Ag NPs produced from aqueous leaf filtrate of Catharanthus roseus and Cissus quadrangularis against parasites Plasmodium falciparum and Hippobosca maculata and Rhipicephalus (Boophilus) microplus [Citation27,Citation39] . Interestingly, the survival ability and adult emergence of Ag NPs treated larvae and pupa of both vectors were observed periodically, where delay in pupation and abnormal adult development with poorly developed wings were also noted and the further investigation is in process.
The beginning of this research is mainly focused on the production of biogenic nanosilver and finding an effective reducing agent and its potential application in the control of mosquito vectors as well as host parasites. The exact mechanisms of larvicidal effect of Ag NPs are unknown. Interestingly, penetration of Ag NPs through treated larval membrane due to interaction with cell molecules resulting in death of larvae was also reported [Citation36]. In addition, after Ag NPs reach their midgut epithelial membrane, the enzymes were inactivated and generate peroxide leading to cell death [Citation40,Citation41] . It is believed with report of Guzman et al. [Citation42] that during anti-bacterial effect of biosilver, the particles highly interacted with sulfur and phosphorus, which are found in abundance throughout the cell membrane and utilize these essential elements for displaying antibacterial properties. Based on this study we revealed that, aqueous leaf filtrate of A. nilagirica (Asteraceae) is very good bioreductant for the synthesis of Ag NPs and synthesized nanoparticles showed their highly potential against dreadful disease causing vectors An. stephensi and Ae. aegypti causative of malaria and dengue, respectively.
5 Conclusion
Phyto-synthesized Ag NPs using a weed, A. nilagirica aqueous leaf filtrate exhibits a rapid synthesis, time consumable, quick process for large scale production, cost-effective, stable, viable, non-toxic, bio-degradable, recyclable and environmentally acceptable green approach and serves as a strong larvicidal and pupicidal agent against developmental stages of An. stephensi and Ae. aegypti. This novel approach would be a boon for the development of alternative biopesticide by utilizing natural resources and an affordable method useful in preventing filariasis, malaria, chikungunya and dengue vectors of public health importance.
Acknowledgements
The authors are thankful to The Management, Karpagam University, Karpagam Academy of Higher Education, Coimbatore for providing all facilities required during this study.
References
- World Health Organization, World Malaria Report, 10 facts on malaria. <www.who.int/features/factfiles/malaria/en/index.html>. 2011; [Accessed 14 March 2013].
- World Health Organization, Action against dengue: Dengue Day Campaigns Across Asia. WHO library cataloguing in publication data. 2011;92.
- C.SundaravadivelanM.NaliniP.SivaprasathL.KishmuBiosynthesized silver nanoparticles from Pedilanthus tithymaloides leaf extract with anti-developmental activity against larval instars of Aedes aegypti L. (Diptera; Culicidae)Parasitol Res1122013303311
- S.G.SalokheS.G.DeshpandeS.N.MukherjeeEvolution of the insect growth regulator Lufenuron (Match®) for control of Aedes aegypti by simulated field trialsParasitol Res111201213251329
- VinayachandraR.ShwethaK.R.ChandrashekarLarvicidal activities of Knema attenuate (Hook.f.&Thomson) Warb. (Myristicaceae) extracts against Aedes albopictus Skuse and Anopheles stephensi ListonParasitol Res109201116711676
- K.MuruganK.KovendanS.VincentD.R.BarnardBiolarvicidal and pupicidal activity of Acalypha alnifolia Klein ex Willd. (Family: Euphorbiaceae) leaf extract and microbial insecticide, Metarhizium anisopliae (Metsch.) against malaria fever mosquito, Anopheles stephensi Liston. (Diptera:Culicidae)Parasitol Res110201222632270
- A.AmerH.MehlhornLarvicidal effects of various essential oils against Aedes, Anopheles and Culex larvae (Diptera, Culicidae)Parasitol Res992006466472
- K.SamiduraiA.SaravanakumarMosquitocidal properties of nereistoxin against Anopheles stephensi, Aedes aegypti and Culex quinquefasciatus (Diptera: Culicidae)Parasitol Res109201111071112
- J.Sivakama ValliB.VaseeharanBiosynthesis of silver nanoparticles by Cissus quadrangularis extractsMater Lett822012171173
- C.UdayasoorianK.Vinoth KumarR.M.JayabalakrishnanExtracellular synthesis of silver nanoparticles using leaf extract of Cassia auriculataDigest J Nano Biostruct62011279283
- M.Mano PriyaB.Karunai SelviJ.A.John PaulGreen synthesis of silver nanoparticles from the leaf extracts of Euphorbia hirta and Nerium indicumDigest J Nano Biostruct62011869877
- N.RoyA.BarikGreen synthesis of silver nanoparticles from the unexploited weed resourcesInt J Nanotech Appl4201095101
- D.JainH.Kumar DaimaS.KachhwalaS.L.KothariSynthesis of plant-mediated silver nanoparticles using papaya fruit extract and evaluation of their antimicrobial activitiesDigest J Nano Biostruct42009557563
- N.SoniS.PrakashEfficacy of fungus mediated silver and gold nanoparticles against Aedes aegypti larvaeParasitol Res1102012175184
- R.B.SalunkheS.V.PatilC.D.PatilB.K.SalunkeLarvicidal potential of silver nanoparticles synthesized using fungus Cochliobolus lunatus against Aedes aegypti (Linnaeus, 1762) and Anopheles stephensi Liston (Diptera; Culicidae)Parasitol Res1092011823831
- A.V.KirthiA.A.RahumanC.JayaseelanL.KarthikS.MarimuthuT.Santhoshkumaret al.Novel approach to synthesis silver nanoparticles using plant pathogenic fungi, Puccinia graminisMater Lett8120126972
- M.Motamedi JuibariS.AbbasalizadehGh.Salehi JouzaniM.NoruziIntensified biosynthesis of silver nanoparticles using a native extremophilic Ureibacillus thermosphaericus strainMater Lett65201110141017
- S.V.OtariR.M.PatilN.H.NadafS.J.GhoshS.H.PawarGreen biosynthesis of silver nanoparticles from an actinobacteria Rhodococcus spMater Lett7220129294
- P.M.ShafiM.K.Geetha NambierR.A.CleryY.R.SarmaS.S.VeenaComposition and antifungal activity of the oil of Artemisia nilagirica (Clarke) PampJ Essent Oil Res162004377379
- A.R.AhameethunisaW.HopperAntibacterial activity of Artemisia nilagirica leaf extracts against clinical and phytopathogenic bacteriaBMC Comp Altern Med10201016
- C.PanneerselvamK.MuruganK.KovendanKumar P.MaheshMosquito larvicidal, pupicidal, adulticidal, and repellent activity of Artemisia nilagirica (Family: Compositae) against Anopheles stephensi and Aedes aegyptiParasitol Res111201222412251
- J.BanerjeeR.T.NarendhirakannanBiosynthesis of silver nanoparticles from Syzygium cumini (L.) seed extract and evaluation of their in vitro antioxidant activitiesDigest J Nano Biostruct62011961968
- C.KrishnarajE.G.JaganS.RajasekarP.SelvakumarP.T.KalaichelvanN.MohanSynthesis of silver nanoparticles using Acalypha indica leaf extracts and its antibacterial activity against water borne pathogensColloids Surf B7620105056
- S.MohantyS.MishraP.JenaB.JacobB.SarkarA.SonawaneAn investigation on the antibacterial, cytotoxic and antibiofilm efficacy of starch-stabilized silver nanoparticlesNanomedicine:NBM9201119
- F.Martinez-GutierrezE.P.ThiJ.M.SilvermanC.C.de OliveiraS.L.SvenssonA.V.Hoeket al.Antibacterial activity, inflammatory response, coagulation and cytotoxicity effects of silver nanoparticlesNanomedicine:NBM82012328336
- M.R.PapasaniG.WangR.A.HillGold nanoparticles: the importance of physiological principles to devise strategies for targeted drug deliveryNanomedicine:NBM82012804814
- S.PonarulselvamC.PanneerselvamK.MuruganN.AarthiK.KalimuthuS.ThangamaniSynthesis of silver nanoparticles using leaves of Catharanthus roseus Linn. G. Don and their antiplasmodial activitiesAsian Pac J Trop Biomed2012574580
- R.RamanibaiK.VelayuthamBioactive compound synthesis of Ag nanoparticles from leaves of Melia azedarach and its control for mosquito larvaeRes Vet Sci9820158288
- World Health Organization, Report of the WHO informal consultation on the evaluation on the testing of insecticides.CTD/ WHO PES/IC/96.l. WHO, Geneva, 1996; 69.
- SPSS (2007) SPSS for Windows. Version 13.0. SPSS, Chicago.
- R.MaheswaranS.SathishS.IgnacimuthuLarvicidal activity of Leucas aspera (Willd.) against the larvae of Culex quinquefasciatus Say. and Aedes aegypti LInt J Integr Biol22008214217
- V.Gopiesh KhannaK.KannabiranG.RajakumarA.Abdul RahumanT.SanthoshkumarBiolarvicidal compound gymnemagenol isolated from leaf extract of miracle fruit plant, Gymnema sylvestre (Retz) Schult against malaria and filariasis vectorsParasitol Res109201113731386
- K.VelayuthamR.RamanibaiLarvicidal activity of synthesized silver nanoparticles using isoamyl acetate identified in Annona squamosa leaves against Aedes aegypti and Culex quinquefasciatusJ Basic Appl Zool7420161622
- C.RajakumarRahuman A.AbdulLarvicidal activity of synthesized silver nanoparticles using Eclipta prostrate leaf extract against filariasis and malaria vectorsActa Trop1182011196203
- N.A.BegumS.MondalS.BasuR.A.LaskarD.MandalBiogenic synthesis of Au and Ag nanoparticles using aqueous solution of black tea leaf extractsColloids Surf B712009113118
- C.SundaravadivelanM.NaliniBiolarvicidal effect of phyto-synthesized silver nanoparticles using Pedilanthus tithymaloides (L.) Poit stem extract against the dengue vector Aedes aegypti L. (Diptera; Culicidae)Asian Pac J Trop Biomed201218
- S.MarimuthuA.Abdul RahumanG.RajakumarT.SanthoshkumarA.V.KirthiC.Jayaseelanet al.Evaluation of green synthesized silver nanoparticles against parasitesParasitol Res108201115411549
- L.SintubinW.VerstraeteN.BoonBiologically produced nanosilver: current state and future perspectivesBiotechnol Bioeng2012115
- T.SanthoshkumarA.Abdul RahumanA.BagavanS.MarimuthuC.JayaseelanA.Vishnu Kirthiet al.Evaluation of stem aqueous extract and synthesized silver nanoparticles using Cissus quadrangularis against Hippobosca maculate and Rhipicephalus (Boophilus) microplusExp Parasitol1322012156165
- M.RaffiF.HussainT.M.BhattiJ.I.AkhterA.HameedM.M.HasanAntibacterial characterization of silver nanoparticles against E. coli ATCC-15224J Mater Sci Technol242008192196
- G.D.ShockmanJ.F.BarrettStructure, function, and assembly of cell walls of gram-positive bacteriaAnn Rev Microbiol371983501527
- M.GuzmanJ.DilleS.GodetSynthesis and antibacterial activity of silver nanoparticles against gram-positive and gram-negative bacteriaNanomedicine:NBM820123745