Abstract
The objective of this study is to analyze the efficiency of different antennas proposed for microwave ablation therapy using numerical simulation and experimental approach. The simulation was done with COMSOL MULTIPHYSICS® software to design antenna prototypes and evaluate its reflection coefficient, power dissipation distribution, power dissipation density, specific absorption rate and temperature distribution in tissue. Antennas were fabricated from a 50 Ω RG405/U semi-rigid coaxial cable to match the geometric prototypes generated during simulation. Ex vivo bovine livers were ablated with the fabricated antennas using 50 W for 5 and 10 min. Ablation diameters, ablation lengths and aspect ratios were determined. Sleeved antenna produced lowest reflection coefficient, high power dissipation, low power dissipation density, high SAR and high temperature in the simulation. Sleeved antenna provides excellent localization, large ablative diameter, low backward heating and high aspect ratio than single slot, dual slot and monopole antennas.
1 Introduction
The potential applications of thermal therapies such as microwave ablation, radiofrequency ablation, high intensity focused ultrasound and cryoablation in tumor treatments have been extensively reviewed in literature [Citation1Citation[2]Citation[3]–Citation4] . Microwave ablation is emerging as an attractive modality for thermal therapy of large soft tissue within a short period of time, making it particularly suitable for tumors in different tissues [Citation3,Citation5] . Microwave ablation produces rapid temperature elevation sufficient to cause instant tissue coagulation and necrosis and it is capable of propagating through all types of biological tissues irrespective of tissues’ relative permittivity and effective conductivities. Tissue destruction occurs when tissues are heated to lethal temperatures with a microwave source. Microwave energy radiates into the tissue through an interstitial antenna that functions to couple energy from MW generator to the tissue. As a result of the radiative nature of the antenna, direct heating occurs in a volume of tissue around the antenna.
The basic microwave system consists of three components: a generator, a power distribution system and antenna. Antenna is the most important component of the system that governs microwave energy distribution during tissue ablation. Antennas such as monopole [Citation6], dipole [Citation7,Citation8] , helical [Citation9], triaxial [Citation10], choked [Citation11], sleeved [Citation12,Citation13] and double slot [Citation14] have been proposed in the field of microwave thermal therapy. Microwave antennas are mostly fabricated from a thin coaxial cables of diameters between 1.5 and 2.5 mm [Citation15]. Concerted efforts are ongoing by several researchers to produce antenna capable of producing large tumor ablation with low backward heating along the antenna shaft in the clinical application of microwave ablation in tumor management. Problems such as backward heating along antenna shaft, poor microwave energy distributions, and small ablation coverage have been reported to associate with most of these proposed antennas [Citation16Citation[17]Citation[18]Citation[19]–Citation20] .
Theoretical modelling have been playing significant roles in the design and optimization of antennas by serving as a quick, convenient and inexpensive tool to evaluate the performance and efficiency of designed antennas [Citation13Citation[14]–Citation15,Citation21,Citation22] . Numerical techniques like the finite element method (FEM), finite integration technique (FIT), finite–difference time-domain (FDTD) and method of moments (MoM) are widely used to discretize the partial differential equations (PDEs) in time and space and solve for the temperature profile in tissue [Citation23]. COMSOL Multiphysics software is equipped with FEM to provide all the tools needed to build and run simulation applications pertaining to antenna geometry, biological materials, meshing, physics components selection (electromagnetic and heat transfer), and a good platform for results evaluation [Citation24]. In this study, we investigate the characteristics and features of proposed antennas for microwave ablation therapy to compare their efficiencies using numerical and experimental techniques.
2 Materials and method
The materials used for this study are COMSOL Multiphysics software version 4.4 (Stockholm, Sweden), a 2.45 GHz solid-state microwave generator (SAIREM SAS 200 W, Neyron Cedex, France), semi-rigid coaxial cable (RG405 Coax, Pasternack Enterprises Inc, Los Angeles), excised bovine liver, Teflon tape and ImageJ software (NIH) for analysis of acquired images.
2.1 Simulation of antennas
The antenna simulation was carried out with COMSOL multiphysics using “heat” and “radiofrequency” modules. The antennas were made from 50 Ω RG405 semi-rigid coaxial cable of 2.2 mm diameter.
The inner conductor (0.51 mm thick) was silver-plated copper clad steel with low-loss solid polytetrafluoroethylene (PTFE) as dielectric (1.16 mm thick) and copper as an outer conductor (0.52 mm thick). COMSOL Multiphysics version 4.4 software allows easy specification of the antenna geometries and to solve Maxwell’s and bio-heat equations in the surrounding tissues [Citation21]. Each antenna is 3.4 mm in diameter which include the Teflon tape to act as catheter (and a floating sleeve where necessary) to provide uniform geometrical structure. The catheter is made of polytetrafluorethylene (PTFE) for hygienic and guidance purpose. The antennas operate at frequency of 2.45 GHz a widely frequency used in microwave ablation. The geometries and dimensions of the studied antennas are represented in .
Bovine liver was modelled as a cylindrical geometry of 40 mm radius and 80 mm in height. The tissue was also considered to be homogeneous with its biological properties taken from literature and compared with the built-in one available in the software [Citation25,Citation26] . Each antenna was modelled using the dimensions shown in along with its specifications indicated in the manufacturer technical sheet of the RG405 semi rigid coaxial-cable. After the antennas have been designed, microwave energy was set at the upper end of dielectric component of the antenna. An electromagnetic wave propagating in an antenna, is characterized by transverse electromagnetic fields (TEM) while in the tissue, an electromagnetic wave is characterized by transverse magnetic fields (TM). Thus, the simulation helped to predict specific absorption rate (SAR), temperature profile and tissue necrosis distributions in the liver using these antennas. Also determined were reflection coefficient, maximum SAR, maximum temperature and total power deposition. Aspect ratio was obtained using 50 °C isothermal contour line for a duration of 10 min. Image J (NIH, version 1.47) was used to determine lesion diameter and longitudinal length of designed and simulated antennas as well as the aspect ratio.
2.2 Experimental validation
The modelled antennas were fabricated from 50 Ω semi-rigid coaxial cable (RG405 Coax, Pasternack Enterprises Inc, Los Angeles) to replicate the geometrical prototypes described in the simulation section (). Slots were created for the emission of the microwave energy with the tip end soldered where necessary. Four antennas (monopole, single slot, dual slot and sleeved antennas) of different geometries were fabricated from the semi-rigid coaxial cables. The antennas were covered with Teflon tape for easy removal from the liver after ablation. Large blood vessels were also avoided during the insertion of the antenna to minimize microwave energy loss from ablating regions. Microwave energy was delivered at input powers of 20, 30, 40 and 50 W for 5 min and 10 min respectively for each of the power selected. At the end of each procedure, the ablated tissue was sliced along the antenna insertion to evaluate ablation diameter and ablation length. Each sliced tissue was photographed for further analysis with ImageJ software. Ablation length, ablation diameter and aspect ratio of the necrosed tissues were also determined.
2.3 Statistical analysis
Differences in ablation diameters, ablation lengths and aspect ratios of the four antennas were analyzed to study the relationship between them using ANOVA (Statistical Package for Social Sciences, SPSS). P-value <.05 was considered to be statistically significant. Also, the percentage difference of ablation coagulation diameter and length of antennas were compared with monopole antenna.
3 Results
Reflection coefficient, power dissipation, SAR distribution, ablation diameters, ablation lengths and aspect ratios are the major parameters to predict efficiency of a suitable antenna meant for microwave ablation therapy. presents reflection coefficient, power dissipation, maximum power dissipation density, maximum specific rate (SAR), maximum temperature (at 50 W, 10 min), ablation diameter, ablation length and aspect ratio of designed and simulated antennas. From the table, sleeved antenna produced the lowest reflection coefficient (−24.2 dB), lowest power dissipation density (125.4 MW/m3) and lowest ablation length (4.93 cm) in comparison with monopole, single slot and dual slot antennas. Also, highest power dissipation (48.9 W), SAR (20.6 kW/kg), temperature (305.0 °C), ablation diameter (4.44 cm) and aspect ratio (0.90) were recorded when compared with other antennas.
Table 1 Comparison of simulation results with different antennas.
The electric field contours in sleeved antenna are more constrained at the distal end due to the presence of the sleeve (). Temperature and necrotic distribution pattern in the liver during simulations (Figs. 3 and 4) are similar to electric field distribution in the modelled tissue (). shows the ablation distribution patterns of the ablated ex vivo bovine livers using different antennas, sleeved antenna produced the largest ablation region than the other antennas. Ablation lengths, ablation diameters and sphericity indexes of the ablated tissues were computed from using different input powers and ablation durations. Generally, inclusion of sleeved into dual slot antenna reduces reflection of microwave along antenna shaft thereby increases microwave energy deposited into the ablated tissue.
Fig. 2 Electric field distribution in tissue using (A) monopole, (B) single slot, (C) dual slot and (D) sleeved antennas.
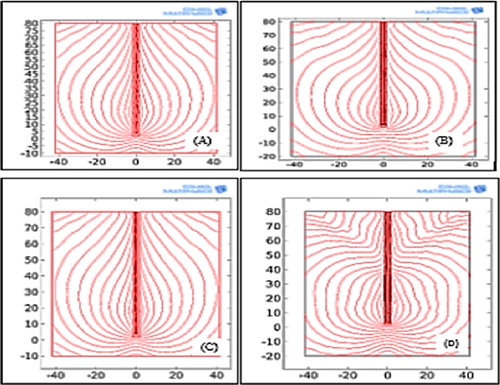
Fig. 3 Temperature distribution in tissue using (A) monopole, (B) single slot, (C) dual slot and (D) sleeved antennas.
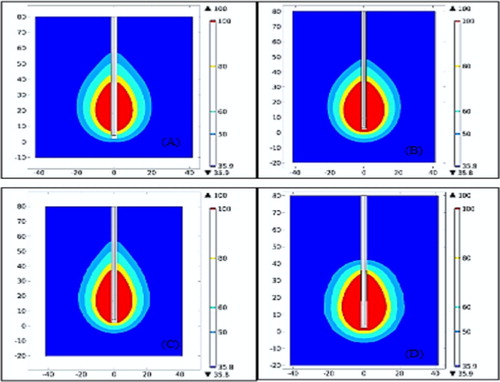
Fig. 4 Necrotic distribution in tissue using (A) monopole, (B) single slot, (C) dual slot and (D) sleeved antennas.
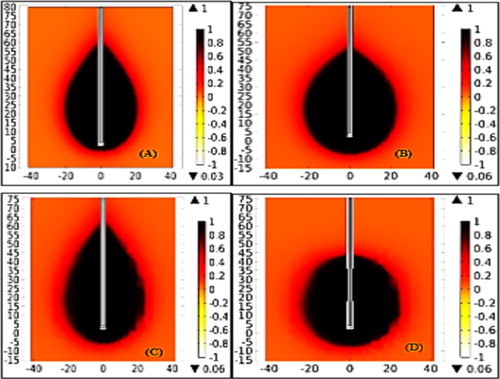
Fig. 5 Experimental validation results with ex vivo liver using (A) monopole, (B) single slot, (C) dual slot and (D) sleeved antennas.
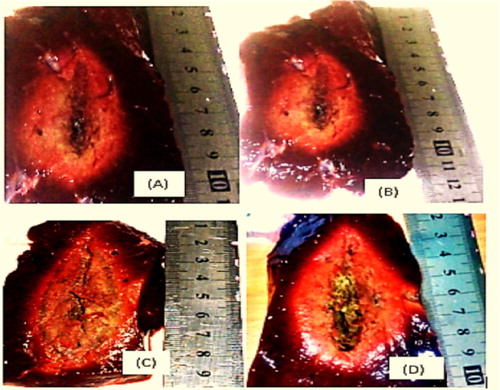
Figs. 6Fig. 7Fig. 8Fig. 9Fig. 10–11 show ablation diameter, ablation length and aspect ratio distributions of ablated bovine ex-vivo liver with input powers of 20, 30, 40, and 50 W at 5 and 10 min. Sleeved antenna produced larger ablation diameter than monopole, single slot and dual slot antennas (Figs. 6 and 7). Ablation length was shorter with sleeved antenna than the others (Figs. 8 and 9). This is an indication that the sleeved antenna has potential of reducing backward heating along shaft during the ablation procedures. Sphericity index of ablated tissue was higher with sleeved antenna than monopole, single slot and dual slot antennas (Figs. 10 and 11). Statistical analysis indicates that, no significance difference was observed in ablation diameter for ablation duration at 5 min (p = .41) and 10 min (p = .53). Likewise, no statistical difference in the mean of the ablation length at 5 min (p = .87) and 10 min (p = .24). Meanwhile, significance difference was observed in their aspect ratios at 5 min (p = .01) and at 10 min (p = .01).
From , by considering ablation diameter, monopole versus sleeved antennas produce the highest percentage difference (32.3%) for 5 min ablation duration and single slot versus sleeved antennas also produced (27.3%) for 10 min ablation duration. Also for ablation length, sleeve versus monopole antennas produced the lowest percentage difference (−15.7%) for ablation duration of 5 min while monopole versus sleeved antennas produce the lowest percentage difference (−15.0%) for 10 min duration. Comparison in the aspect ratios indicate that, monopole versus sleeve antennas produce the highest percentage difference of 45.5% and 40.2% at 5 min and 10 min ablation duration respectively.
Table 2 Average percentage differences in ablation diameters, lengths and aspect ratios of the four antennas.
4 Discussion
This study presents the efficiencies of monopole, single slot, dual slot, and sleeved antennas in microwave ablation technology using numerical and experimental methods. Generally, ablations for five minutes give larger necrosis diameter for all applied input powers compare to 10 min duration. Backward heating was generally lowest in sleeved antenna when compared with the monopole, single slot and dual slot antennas. Aspect ratio was generally high with sleeved antenna compared to other antennas. For ablation duration of 10 min at different input powers; ablation diameter, ablation length and aspect ratio produced by a dual slot with sleeved are higher than those of monopole, single slot and double slot antennas. It was generally observed that ablation diameter and ablation length are input power and ablation duration dependent (Figs. 4Fig. 5Fig. 6Fig. 7Fig. 8–9).
Antenna efficiency in microwave ablation has been reported to depend on reflection coefficient, power dissipation and SAR distribution pattern in the ablated tissue [Citation16]. Meanwhile, high reflection coefficient usually indicates low power dissipation into the tissue and an evidence of impedance mismatch between the ablated tissue and the antenna [Citation15]. Many authors have reviewed antennas meant for microwave ablation with emphasis on their strengths and shortcomings which have also be substantiated in this study [Citation15,Citation16] . The results obtained in this study corroborate with previous reports on features of monopole antenna such as high reflection coefficient, low power dissipation, low aspect ratio and elongated SAR along antenna shaft [Citation6,Citation16] . The major advantage of monopole antenna is its ability to be easily fabricated from semi rigid coax-cable as well as simple geometries (). Single slot antenna with a small ring slot cut close to the short-circuited distal tip has been suggested and reviewed by many authors [Citation16]. Like monopole, single slot antenna still suffers from backward heating and insertion depth problems, notwithstanding it has ability to deposit more microwave energy into the tissue than the monopole [Citation16]. Dual slot antenna was introduced to overcome shortcomings exhibited by monopole and single slot antennas. Previous reports showed that dual slot antenna is more efficient in high power localization than the previous designed antennas even though the backward heating was not totally eliminated [Citation14]. Sleeved antenna was introduced to increase power localisation efficiency and minimize backward heating along the antenna shaft to the barest minimum [Citation12,Citation13] . Although, its geometrical configuration is complex as well as its fabrication procedures yet ability to localize microwave energy into tissue makes it the best choice for ablation among the proposed antennas.
This study established that, monopole and slot antennas will be suitable for palliative and percutaneous procedures because of their small diameters despite being characterized by elongated heating patterns along antenna shaft [Citation15]. Sleeved antenna was found to confine more electric field, microwave energy and temperature to its distal end than monopole, single slot and dual slot antenna [Citation19,Citation20] . The choice of an antenna and its application for microwave ablation depends mostly on clinical indications such as Tumor site, location, adjacent organ and morphology [Citation14,Citation15,Citation22Citation[23]Citation[24]Citation[25]Citation[26]–Citation27] .
5 Conclusion
The outcomes of this study clearly proved that, sleeved antenna exhibits greatest ability to localize microwave energy into tissues by producing highest sphericity index and temperature distribution with the lowest backward heating effect than the other antennas studied. This study has also provided greater insight into the features and characteristics of proposed antennas for microwave ablation using both numerical and experimental procedures.
Funding
This research did not receive any specific grant from funding agencies in the public, commercial, or not-for-profit sectors.
References
- R.W.Y.HabashR.BansalD.KrewskiH.T.AlhafidThermal therapy, Part III: ablation techniquesCrit Rev Biomed Eng35200737121
- C.J.SimonD.E.DupuyW.W.Mayo-SmithMicrowave ablation: principles and applicationsRadioGraphic2520056983
- M.AhmedC.L.BraceF.T.LeeS.N.GoldbergPrinciples of and advances in percutaneous ablationRadiology2582011351369
- L.ZhangN.WangQ.ShenW.ChengG.-J.QianTherapeutic efficacy of percutaneous radiofrequency ablation versus microwave ablation for hepatocellular carcinomaPLoS ONE8102013e76119
- Ahmedet al.Image-guided tumor ablation: standardization of terminology and reporting criteria–a 10-year updateJ Vasc Interv Radiol2511201416911705
- S.LabonteH.O.AliL.RoyMonopoles for microwave catheter ablation of heart tissueIEEE Trans Microw Theory Tech11995303306
- W.HurterF.ReinboldW.J.LorenzA dipole antenna for interstitial microwave hyperthermiaIEEE Trans Microw Theory Tech39199110481054
- Yang D. Measurements, antenna design and advanced modeling for microwave ablation. PhD thesis. University of Wisconsin, Department of Electrical Engineering; 2005. <www.rf-ablation.engr.wisc.edu/papers/Yang_Thesis.pdf>.
- H.LuyenS.C.HagnessN.BehdadA balun-free helical antenna for minimally invasive microwave ablationIEEE Trans Antennas Propag632015959965
- L.C.BraceW.D.van der WeideT.F.LeeF.P.LaesekeL.SampsonAnalysis and experimental validation of a triaxial antenna for microwave tumor ablationIEEE MTTS Int Microw Symp3200414371440
- Bertram JM, Yang D, Converse MC, Webster GJ, Mahvi DM. Antenna design for microwave hepatic ablation using an axisymmetric electromagnetic model. Biomed Eng Online 2006; 5: 15. Available: <http://www.biomedical-engineering-online.com/content/5/1/15>.
- D.YangJ.M.BertramM.C.ConverseJ.G.WebsterS.C.HagnessJ.A.Willet al.A floating sleeve antenna yield localized hepatic microwave ablationIEEE Trans Biomed Eng532006533537
- Z.A.IbitoyeE.O.NwoyeM.A.AwedaA.A.OremosuC.C.AnnunobiO.N.AkanmuOptimization of dual slot antenna using floating metallic sleeve for microwave ablationMed Eng Phys372015384391
- C.L.BraceDual-slot antennas for microwave tissue heating: parametric design analysis and experimental validationMed Phys38201142324240
- C.L.BraceMicrowave tissue ablation: biophysics, technology and applicationsCrit Rev Biomed Eng3820106578
- J.M.BertramD.YangM.C.ConverseJ.G.WebsterD.M.MahviA review of coaxial-based interstitial antennas for hepatic microwave ablationCrit Rev Biomed Eng342006187213
- K.SaitoT.TaniguchiH.YoshimuraK.ItoEstimation of SAR distribution of a tip split array applicator for microwave coagulation therapy using the finite element methodIEICE Trans Electron842001948954
- L.HamadaBasic analysis of SAR distribution of coaxial-slot antenna array for interstitial microwave hyperthermiaIEICE Trans Electron78199516241631
- K.ItoK.SaitoMicrowave antennas for thermal therapyTherm Med2320072330
- C.L.BraceMicrowave ablation technology: what every user should knowCurr Probl Diagn Radiol3820096167
- P.PrakashTheoretical modeling for hepatic microwave ablationOpen Biomed Eng J420102738
- M.G.LubnerC.L.BraceJ.Louis HenshawF.T.LeeJrMicrowave tumor ablation: mechanism of action, clinical results, and devicesJ Vasc Interv Radiol212010192203
- J.W.HandModelling the interaction of electromagnetic fields (10MHz-10GHz) with the human body: methods and applicationsPhys Med Biol532009246286
- COMSOL Multiphysics, Users’ Guide. Electromagnetic Module and Heat Transfer Module, Version 4.4, Sweden; 2013. <www.comsol.com/support/download>.
- Andreuccetti D, Fossi R, Petrucci C. An internet resource for the calculation of the dielectric properties of body tissues in the frequency range 10 Hz–100 GHz. IFAC-CNR, Florence, Italy; 1997. Available: <http://www.niremf.ifac.cnr.it/tissprop> [accessed 19 November, 2015].
- Hasgall PA, Di Gennaro F, Baumgartner C, Neufeld E, Gosselin MC, Payne D, et al. IT’IS database for thermal and electromagnetic parameters of biological tissues; 2015. Available: <http://www.itis.ethz.ch/database> [accessed 12 December, 2015].
- J.ChiangK.A.HynesM.BedoyaC.L.BraceA dual-slot microwave antenna for more spherical ablation zones: ex vivo and in vivo validationRadiology2682013382389