Abstract
The present study aims to survey exopolysaccharides (EPSs) production in 29 bacterial strains isolated from the sediments around the mangrove trees in Ras Mohamed area, Red Sea Coast, Sinai Peninsula, Egypt. Two of the strains were able to produce EPSs. A higher yield of EPS was obtained from isolate No. 12. Strain identification resulted in a close similarity with Bacillus altitudinis. The produced EPS was characterised as a heteropolysaccharide containing mannouronic acid, glucose, and sulphate. A gel permeation chromatography was used to estimate the EPS molecular weight which found to be 4.23 × 105 Dalton. The typical pattern of polysaccharide absorbance was supported by the infrared spectrum. The EPS appears significant in vitro antitumor activities against two cancers cells EACC and lung cancer A-549. Furthermore, a broad range of bacteria and fungi were inhibited with purified EPS.
1 Introduction
Exopolysaccharides (EPSs) are long chains of high molecular mass polymers produced by different microorganisms, including bacteria, fungi and blue green algae [Citation1]. EPSs produced by bacteria exhibit significant structural diversity with novel biological properties are considered as valuable sources of natural polymers with multiple biotechnological applications. Microbial EPSs are prevalent in the extreme marine environment where they are essential for microbial existence. Most of the functions attributed to EPSs are of a protective nature and their accurate roles are dependent on the ecological functions in which the microorganisms live. They could support the microbial communities to suffer extremes of temperature, salinity and nutrient accessibility, construct a boundary between the bacterial cell and its included environment.
Bacteria in marine environments were forced with osmotic stress to produce EPSs with unique composition were studied for prospective application in various sectors [Citation2] and for discovery of novel macromolecules [Citation3]. Recently, evidences for antioxidant, immune-modulation, antitumor and antimicrobial properties of EPSs producing microorganisms have increased [Citation4,Citation5] .
Serious side effects of clinically used antitumor drugs direct the attention towards investigation of novel agents with a higher potency and fewer effects [Citation6]. Human pathogens, as well, have resistance formed from the abnormal use of commercial antimicrobial drugs. This resistance with the undesirable side effect of certain antibiotics motivated the scientists to look for new antimicrobial substituent from many sources [Citation7].
The aim of this study is to deal with the isolation and characterisation of EPS producing bacteria from the marine sediments around the mangrove trees in Ras Mohamed area, Red Sea Coast. The in vitro activities of purified EPS as antitumor agent against Ehrlich Ascites carcinoma and lung cancer cell lines as well as the antimicrobial activities against Gram positive and Gram negative bacteria, yeast and fungi were also studied.
2 Materials and methods
2.1 Isolation of bacteria and production of EPSs
Marine sediment was collected from the rhizosphere of a mangrove trees (Avicennia marina) from Ras Mohamed, Sinai Peninsula, Egypt. The sample was serially diluted in 90 ml sterile water and plated on a medium containing (in g/l) [glucose (20); yeast extract (0.1); NH4NO3 (0.8); CaCO3 (1); K2HPO4 (0.6); KH2PO4 (0.5); MgSO4·7H2O (0.05), MnSO4·4H2O (0.1) and agar (15)] which dissolved in 750 ml seawater and 250 ml distilled water [Citation8]. EPSs producing bacterial isolates were selected based on colonies phenotype (smooth and mucoid).
Pure strains were then inoculated in 250 ml conical flasks contain 50 ml of previous medium and incubated at 37 °C in a rotary shaker at 120 rpm for 48 h. After centrifugation at 5000 rpm for 15 min, supernatant was mixed with four volumes of chilled ethanol, while the collected pellets were washed twice with acetone and diethyl ether [Citation5] and then drying at 50 °C to obtain constant weight. EPSs formation were estimated by the phenol-sulphuric acid method [Citation9].
2.2 Identification of the strain
The strain with the highest EPS production was identified according to their morphology, physiology and biochemistry features such as nitrate reduction, catalase, oxidase, Voges-Proskauer test, acid production from glucose [Citation10] and 16S rRNA sequence. The 16S rRNA gene was amplified and sequenced using universal primers described by Weisburg et al. [Citation11]. The sequences were compared with the GenBank data base using BLAST [Citation12].
2.3 Extraction and purification of EPS
The selected strain was grown in a fermentation medium containing (g/l) [peptone (4.0), yeast extract (2.0), and sucrose (20.0)] which dissolved in 750 ml seawater and 250 ml distilled water (pH 7–7.5) at 37 °C for three days in an incubator shaker at 120 rpm to produce EPS [Citation13]. To precipitate proteins, 5% trichloroacetic acid was added to cell free culture supernatant and incubated at 4 °C for 24 h. After centrifugation at 5000 rpm for 15 min, supernatant pH was adjusted to 7 and dialyzed against distilled water using dialysis tube (MWCO 2000). After dialysis, absolute ethanol were added to supernatant and incubated at 4 °C for 24 h. The precipitated EPS was dissolved, dialysed against deionized water and filtered using 0.45 mm filter, then applied to a DEAE-cellulose column (1.5 cm × 70 cm). Elution was carried out using continuous gradient NaCl solution (0.2–3.0 M). Subsequently, a further purification step was carried out for the collected fractions using a Sephadex G-200 column (2 cm × 80 cm) which was eluted with 0.1 M NaCl at a flow rate of 0.5 ml/min. The fractions with EPS were collected in one fraction which dialyzed and lyophilized for further analysis.
2.4 Chemical analyses of EPS
Protein, uronic acid and monosaccharide sulphate were estimated for the produced EPS according to methods described by many authors [Citation10,Citation14Citation[15]–Citation16] respectively. A high performance liquid chromatography (HPLC) was used to analyse the monosaccharide content using a Shimadzu Shim-Pack SCR-101N column (7.9 mm × 30 cm) and deionized water as mobile phase at flow rate 0.5 ml/min utilizing acid hydrolysis technique [Citation17].
2.5 Molecular weight estimation
The molecular weight of EPS was estimated by gel permeation chromatography (GPC) on a Sephadex G-200 column (80 cm × 2 cm). Standard dextrans (40,000; 500,000 and 2,000,000) Daltons were used for calibration and the molecular weight was determined by plotting against standard graph [Citation18].
2.6 Fourier-transform infrared spectroscopy (FT-IR)
The dried EPS was mixed to KBr powder, ground and pressed into a 1 mm pellets for FT-IR measurements in the range of 400–4000 cm−1 using Bruker scientific FT-500-IR spectrophotometer [Citation19].
2.7 Periodate oxidation and Smith degradation
Periodate oxidation and Smith degradation were determined by dissolving desulfated EPS (16 mg) in distilled water (6 ml) and then added to 0.1 M NaIO4 (50 ml) in round bottom flasks which kept at 4 °C in dark [Citation20]. Aliquots (0.1 ml) were taken every 24 h and diluted with distilled water (25 ml) which read in spectrophotometer at 223 nm.
Periodate consumption was calculated based on absorbance change [Citation21] and formic acid products were monitored by the phenol-sulphuric acid method [Citation9]. Ethylene glycol (2 ml) was added and the solutions were dialyzed against distilled water for 48 h. After that NaBH4 (100 mg) is added and the mixture was left for 24 h temperature and then ice cold acetic acid (4N) is added to stop the reaction. The solutions were again dialyzed as described above and lyophilized [Citation22]. The resulting polyalcohol was hydrolyzed with HCOOH 90% for 5 h and the produced sugars and sugar alcohols were analyzed by HPLC.
2.8 Biological activity of EPS
2.8.1 Radical scavenging activity of EPS towards DPPH radical
The free radical scavenging activity of EPS was estimated according to method describing by Yang et al. [Citation23] using 1,1-diphenyl-2-picryl-hydrazyl (DPPH). Five ml of DPPH in ethanol was added to 1 ml of purified EPS with concentrations (50–300 µg/ml). The mixture was incubated in dark for 30 min. and measured at 517 nm using spectrophotometer UV–Visible 2401PC (Shimadzu, Japan).
The DPPH absorbance goes down with a high free radical scavenging ability. The free radicals scavenger capacity was calculated based on this equation: Scavenging ability (%) = [(A517 of control − A517 of sample)/A517 of control] × 100. The EC50 value is considered as the effective concentration (µg) of EPS at which the DPPH absorbance was reduced by 50%.
2.8.2 Antitumor activity against Ehrlich Ascites Carcinoma Cells (EACC)
Tumor cells viability was measured with modified cytotoxic trypan blue exclusion technique as described by Yang et al. [Citation23]. Tumor cells proliferation inhibition was calculated based on this equation: [(A−B/A] × 100%, where A and B are the average number of viable tumor cells of the control and the samples, respectively.
2.8.3 Antitumor activity against lung cancer cell line A-549
Cytotoxicity of purified EPS was measured against A-549 cell line using the MTT cell viability assay [Citation24]. Percentage of relative viability was calculated using the equation (Absorbance of treated cells/Absorbance of control cells × 100). Then the half maximal inhibitory concentration IC50 was calculated from the graph and BJ-1 normal human cell line was used as control.
2.8.4 Antimicrobial activity
The antimicrobial potential of the purified EPS was tested against a wide set of microorganisms including: (i) Gram positive bacteria (Bacillus subtilis NRRL B-941 and Staphylococcus aureus NRRL B-767), (ii) Gram negative bacteria (Pseudomonas aeruginosa NRRL B-23 and Escherichia coli NRR-B 210), (iii) yeast (Saccharomyces cerevisiae Y-2034 and Candida albicans NRRL Y-477) and (iv) fungi (Aspergillus niger NRRL-3 and Fusarium oxysporum NRRL 26406). The inhibition zones produced by different concentration of EPS (75, 100, 150, and 200 μg/disc) were determined. Rimactane as antibacterial (200 μg/disc) and flucoral as antifungal (200 μg/disc) were used as control [Citation25].
3 Results and discussion
3.1 Isolation, screening, and identification of the EPS producing bacteria
Many marine bacteria produce EPSs for their growth to adhere to solid surfaces and to overcome extreme conditions. In the present study, 29 bacterial strains were isolated from the rhizosphere sediment of mangrove trees in Ras Mohamed area, Red Sea; Sinai, Egypt. Two of them had the ability to produce EPSs. The highest production of EPS (10.33 g/l) was detected at strain No. 12. Liu et al. [Citation26] reported in a study that 19 strains of bacteria produced EPSs in 2.28–9.02 g/l, where they selected a strain because of its highest EPS production and identified it as Bacillus licheniformis.
Colonies of strain No. 12 showed mucoid appearance on solid medium. The cells were Gram positive, rod shaped, motile and spore forming when observed under phase contrast microscope. In nitrate reduction, Voges-Proskauer tests and acid production from glucose, catalase and oxidase testes, the strain was positive, while negative for gas production from glucose. Hence, strain No. 12 was temporary identified as Bacillus sp. particularly, the partially sequenced 16S rRNA genes showed 99% similarity with Bacillus altitudinis, so strain No. 12 was identified as Bacillus altitudinis MSH2014 with accession No. (KY550404) ().
3.2 Characterisation of EPS
3.2.1 Purification of EPS
The crude EPS produced from Bacillus altitudinis MSH2014 were purified using DEAE-cellulose anion exchange chromatography column (). Elution profile showed one major peak (fraction 100–120). Such fraction was collected, dialyzed and lyophilized to get EPS which applied for further analysis.
3.2.2 Molecular weight determination of EPS
The molecular weight of the EPS produced by Bacillus altitudinis MSH2014 determined by a gel filtration technique was 4.23 × 105 Da. EPS appeared as a single symmetrical narrow peak (), which represent the homogeneity of EPS. Previous reports of the molecular weight of the EPS produced by Bacillus licheniformis was 2.826 × 104 Da [Citation26].
3.2.3 Chemical composition of EPS
The purified EPS produced a negative response by Bradford test indicating the absence of protein. Monosaccharide analysis by HPLC revealed that EPS composed of mannuronic acid and glucose with molar ratio 1:2.2. The chemical composition of EPS indicated the presence of uronic acid (14.26%) and sulphate (15.47%).
It was found that bacteria isolated from deep sea which produced EPS was typically acidic due to containing 10–40% uronic acid [Citation27] and polyanionic due to uronic acids, ketal-linked pyruvate or inorganic residues such as PO4− or SO3− existence [Citation28]. According to the literatures, the presence of SO3− group enhanced the medical potential of EPS [Citation29]. The sugar composition had an important role in the biological activities [Citation30].
The FT-IR spectrum of EPS from Bacillus altitudinis MSH2014 showed a typical pattern for polysaccharide absorbance (). The broad band at 3435.6 cm−1 is due to –OH groups of carbohydrate residues stretching vibration [Citation31]. The 2360.0 cm−1 band is due to C–H stretching vibration. The weak absorbance peak at 1630 cm−1 was due to the stretching vibration of C=O that may be associated with the mannuronic acid and internal hydrogen bonds [Citation32]. Band at 1340 cm−1 is due to the presence of S=O and/or C–O–S bonds [Citation33] and the band at 863 cm−1 indicates the α-configuration which is simultaneously present in EPS.
3.3 Periodate oxidation and Smith degradation
Periodate oxidation was done to both sulphated and desulphated EPS where in case of sulphated EPS did not produce formic acid and consumed periodate due to the presence of sulphate groups on the carbon atom 2 or 3 which impede the process of periodate oxidation. But desulfated exopolysaccharide consumed periodate (0.942 mol) to produce formic acid (0.000801 mol) per one mole of anhydrosugar. HPLC analysis of EPS from Bacillus altitudinis MSH2014 shows erythritol, glycerol, and erytheric acid presence (). The presence of a small amount of glycerol and free erythritol in large amount partially demonstrate existence of (1 → 4) linkages between monosaccharides units and sulphate groups on the C2 and/or C3 in EPS. Erythritol was produced from C3, C4, C5 and C6 of the (1 → 4) glycosidic linkages of glucose after hydrolysis of the backbone, while erytheric acid was liberated from C3, C4, C5 and C6 of the (1 → 4) glycosidic linkage of mannouronic acid. The presence of relatively small quantities of glycerol from the glucose units and erytheric acid from the mannouronic unit in the hydrolysis of polysaccharides, gave the information that glucose may be found at the non-reducing end. These outcomes are in good agreement with those mentioned by other workers [Citation34,Citation35] .
Table 1 HPLC results of Smith's degradation of EPS produced by Bacillus altitudinis MSH2014.
3.4 Biological activity of EPS produced by Bacillus altitudinis MSH2014
3.4.1 Free radical scavenging activities of EPS
The free radical scavenging activity was estimated with DPPH [Citation36]. The scavenging activity of EPS illustrates in showed a concentration-dependent manner, with an EC50 value of 150 (µg/ml). It was reported that EPS was isolated from Bacillus coagulans RK-02 and purified by size exclusion chromatography which showed in vivo antioxidant activities [Citation37]. Agili and Mohamed [Citation38], also, mentioned that EPSs from Padina pavonica had antioxidant activities. The antioxidant properties of polysaccharides are mainly correlated to their confirmation, structure, molecular mass and their monosaccharides components [Citation39].
3.4.2 Antitumor activity
3.4.2.1 Antitumor activity against Ehrlich Ascites Carcinoma Cells (EACC)
As shown in , the EPS exerted inhibitory activity on EACC in concentration dependent manner. The viability of tumor cells after incubation with purified EPS drastically decreased from 47 to 20% and then gradually decreased further to 10% by increasing the concentration of EPS from 100 to 500 till 1500 µg/ml respectively, which is in agreement with the results obtained by Ahmed and Ahmed [Citation40].
3.4.2.2 In vitro antitumor activity against lung cancer cell
Lung cancer cell line A-549 was treated with the purified EPS at different concentrations (12.5, 25, 50 and 100 µg/ml) and showed in . The data shows a gradual decrease in tumor cells viability with high concentrations of EPS. The highest cells viability (71%) was found at 12.5 µg/ml which decreases to 63%, 51% and 39% at 25 µg/ml, 50 µg/ml, 100 µg/ml of EPS, respectively, and IC50 was 51.94 µg/ml. While in case of normal cell line the viability of cells reached 95%, 90%, 88% and 77% when treated with the different concentrations of EPS 12.5, 25, 50 and 100 µg/ml respectively.
Fig. 7 Antitumor activity against lung cancer of the purified EPS from Bacillus altitudinis MSH2014.
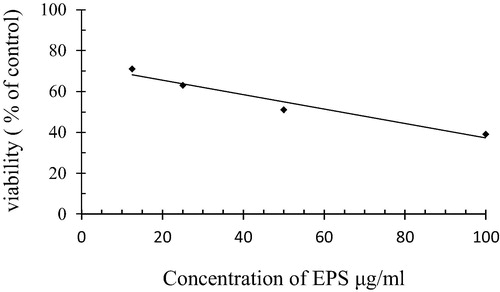
The differences in the antitumor activities of EPS may be due to their different physicochemical properties such as chain shape, molecular weight and monosaccharide composition [Citation41]. EPS molecular weight may affect its bioactivities, as the high molecular weight showed more antitumor activity [Citation42].
3.4.3 Antimicrobial activities
The antimicrobial activities () demonstrate that the purified EPS possessed antimicrobial activities against all of the tested microorganisms and by increasing the concentration of EPS the inhibition zone increased which ranged from 6.3 to 24.9 mm. Orsod et al. [Citation43] isolated two EPSs producers marine bacteria and screened their antimicrobial activities against both Gram positive bacteria (Lysinibacillus and Paenibacillus sp.) and Gram negative bacteria (Pseudomonas sp., Escherichia coli) had shown presence of inhibition zones.
Table 2 Inhibition zones of microbial growth by different concentrations of the EPS from Bacillus altitudinis MSH2014.
The mechanism of the antimicrobial activity of the purified EPSs were studied by various investigators and they mentioned that the antimicrobial activity of anionic polysaccharides such as sulphated polysaccharide occurs by several mechanisms through its chelation activities and the deprivation of metal, trace elements or essential nutrients which limit the growth of microorganisms [Citation44].
4 Conclusion
Although EPSs are one of the abundant bioactive components, their study still remains an interesting scope for their high molecular diversity, complexity and high capability for drugs design and preparation. In addition, microorganisms producing EPSs are promising sources that meet actual industrial demand, especially, marine microorganisms which offer great opportunities for new bioactive compound discovery.
Conflict of interest statement
The authors declare that there is no conflict of interest with any financial organization or corporation or individual that can inappropriately influence this work.
References
- S.GuoW.MaoY.LiJ.TianJ.XuStructural elucidation of the exopolysaccharides produced by fungus Fusarium oxysporum Y24Carbohydr Res3652013913
- M.C.A.NicholsJ.GuezennecJ.P.BowmanBacterial exopolysaccharides from extreme marine environments with special consideration of the Southern Ocean, Sea Ice, and Deep-Sea hydrothermal ventsMarine Biotechnol72005253271
- I.FinoreP.Di DonatoV.MastascusaB.NicolausA.PoliFermentation technologies for the optimization of marine microbial exopolysaccharides productionMar Drugs12201430053024
- Y.ZhangH.KongY.FangK.NishinariG.O.PhilipsSchizophyllan: a review of its structure, properties, bioactivity and recent developmentsBioact Carbohydr Dietary Fibre120135371
- W.LiJ.JiX.RuiJ.YuW.TangX.Chenet al.Production of exopolysaccharides by Lactobacillus helveticus MB2-1 and its functional characteristics in vitroLWT-Food Sci Technol592014732739
- Z.H.ZhangJ.H.TangZ.L.ZhanX.L.ZhangH.H.WuCellular toxicity of isoniazid together with rifampicin and the metabolites of isoniazid on QSG-7701 hepatocytesAsian Pac J Trop Med52012306309
- M.RamachandranT.ThirumalaiP.VinothkumarAntibacterial activity of various leaf extracts of Merremiae marginata EK ElumalaiAsian Pac J Tropical Biomed12011406408
- Y.O.KimH.K.KimK.S.BaeJ.H.YuT.K.OhPurification and properties of a thermostable phytase from Bacillus sp. DS11Enzyme Microb Technol22199827
- M.DuboisK.A.GillesJ.K.HamiltonP.A.RebersF.SmithColorimetric method for determination of sugars and related substancesAnal Chem281965350356
- J.G.HoltM.E.SharpeS.T.WilliamsBergey’s manual of systematic bacteriology1989Williams & WilliamsBaltimore, London
- W.G.WeisburgS.M.BarnsD.A.PelletierD.J.Lane16S ribosomal DNA amplification for phylogenetic studyJ Bacteriol1731991697703
- K.TamuraJ.DudleyM.NeiS.KumarMolecular evolutionary genetic analysis (MEGA) software version 4.0Mol Biol Evol24200715961599
- Z.D.JiangP.R.JensenW.FenicalA.LobophorinsNew anti-inflammatory macrolides produced by a tropical marine bacteriumBioorg Med Chem Lett9199920032006
- M.M.BradfordA rapid and sensitive method for the quantitation of microgram quantities of protein utilizing the principle of protein–dye bindingAnal Biochem721976248254
- T.M.C.Filisetti-CozziC.CarpitaMeasurement of uronic acids without interference from neutral sugarsAnal Biochem1971991157162
- K.S.DodgsonR.G.PriceA note on the determination of the ester sulfate content of sulfated polysaccharidesBiochem J1962106110
- H.J.KwonJ.KimDetermination of monosaccharides in glycoproteins by reverse-phase high- performance liquid chromatographyAnal Biochem2151993243252
- D.LuoIdentification of structure and antioxidant activity of a fraction of polysaccharide purified from Dioscorea nipponica MakinoCarbohydr Polym742008544549
- B.RayPolysaccharides from Enteromorpha campressa: isolation, purification and structural featuresCarbohydr Polym662006408416
- W.YalinP.YuanjingS.CuirongIsolation, purification and structural investigation of a water-soluble polysaccharide from Solanumlyratum lyratum ThunbInter J Biolog Macromol362005241245
- A.JeansA.WilhanPeriodic oxidation of DextranJ Amer Chem Sci72195026552657
- S.MondalI.ChakrabortyM.PramanikD.RoutS.IslamStructural studies of water soluble polysaccharides of an edible mushroom, Termitomyces eurhizus (Areinvestigation)Carbohydr Res339200411351140
- Z.F.YangY.H.ZhengS.F.CaoEffect of high oxygen atmosphere storage on quality, antioxidant enzymes, and DPPH-radical scavenging activity of Chinese bayberry fruitJ Agric Food Chemist572009176181
- M.B.HansenS.E.NielsenK.BergRe-examination and further development of a precise and rapid dye method for measuring cell growth/cell killJ Immunol Methods1191989203210
- A.BauerW.KirbyJ.SherrisM.TurckAntibiotic susceptibility testing by standardising single disc methodAm J Clin Pathol361966493496
- C.LiuJ.LuL.LuY.LiuF.WangM.XiaoIsolation, structural characterization and immunological activity of an exopolysaccharide produced by Bacillus licheniformis 8–37-0-1Bioresour Technol101201055285533
- P.PriyankaA.ArunP.RekhaSulfated exopolysaccharide produced by Labrenzia sp. PRIM-30, characterization and prospective applicationsInter J Biolog Macromol692014290295
- A.PoliG.AnzelmoB.NicolausBacterial exopolysaccharides from extreme marine habitats: production, characterization and biological activitiesMar Drugs8201017791802
- K.SenniJ.PereiraF.GuenicheC.Delbarre-LadratC.SinquinJ.RatiskolMarine polysaccharides: a source of bioactive molecules for cell therapy and tissue engineeringMar Drugs9201116641681
- P.Jong-MinH.Ki-BaikK.Sang-OhK.Eun-HeeThe anti-inflammatory effects of acidic polysaccharide from Artemisia capillaris on Helicobacter pylori infectionJ Cancer Preven182013161168
- W.Brock-NeelyInfrared spectra of carbohydratesAdvances in carbohydrates chemistry121957Academic Press IncNew York1333
- A.SynytsyaK.MickovaA.SynytsyaI.JablonskyJ.SpevacekV.Erbanet al.Glucans from fruit bodies of cultivated mushrooms Pleurotus ostreatus and Pleurotus eryngii: Structure and potential prebiotic activityCarbohydr Polym762003548556
- M.HasuiM.MatsudaK.OkutaniS.ShigetaStructural analysis of the lactate associated galactan sulfate produced by Gymnodinium sp.A3T.YasumotoY.OshimaY.Fukuyo1996Armful and toxic algal booms. Inc., Inter Governmental Oceanographic Commission of UNESCO
- M.Abdel-AkherJ.K.HamiltonR.MontgomeryF.SmithA new procedure for the determination of the fine structure of polysaccharidesJ Am Chem Soc74195249704971
- J.DanishefkL.R.WhistlerF.A.BettelheimIntroduction to polysaccharide chemistryP.WaidH.DprekH.AnthoryThe carbohydrates chemistryVol. IIA1970Academic Press IncNew York and London375411
- S.E.LeeH.J.HwangJ.S.HaH.S.JeongJ.H.KimScreening of medicinal plant extracts for antioxidant activityLife Sci732003167179
- V.P.KodaliR.S.PeraliR.SePurification and partial elucidation of the structure of an antioxidant carbohydrate biopolymer from the probiotic bacterium Bacillus coagulans RK-02J Nat Prod742011692697
- F.A.AgiliS.F.MohamedPolysaccharides from Padina pavonica: chemical structural and antioxidant activityAust J Basic Appl Sci62012277283
- C.O.BamigboyeJ.K.OlokeJ.F.DamesBiological activity of extracellular and intracellular polysaccharides from pleurotus tuber-regium hybrid and mutant strainsJ Food Nutrit Res42016422428
- O.M.AhmedR.R.AhmedAnti-Proliferative and apoptotic efficacies of Ulvan polysaccharides against different types of carcinoma cells in vitro and in vivoJ Cancer Sci Ther62014202208
- Q.WuH.M.TunF.C.C.LeungN.P.ShahGenomic insights into high exopolysaccharides producing dairy starter bacterium Streptococcus thermophilus ASCC 1275Sci Rep4201418
- Y.PengL.ZhangF.ZengF.J.KennedyStructure and antitumor activities of the water soluble polysaccharide Ganoderma tsugae myceliumCarbohydr Polymer592005385392
- M.OrsodM.JosephF.HuyopCharacterization of exopolysaccharides s produced by Bacillus cereus and Brachybacterium sp. isolated from Asian Sea Bass (Latescalcarifer). MalaysianJ Microbiol82012170174
- K.Skalicka-WoźniakJ.SzypowskiR.LosM.SiwulskiK.SobieralskiK.GlowniakA.MalmEvaluation of polysaccharides content in fruit bodies and their antimicrobial activity of four Ganoderma lucidum (W Curt.: Fr.) P. Karst. Strains cultivated on different wood type substratesActa Soci Botani Poloniae8120121721