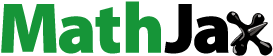
Abstract
The microbes can hydrolyze urea by urease enzyme to produce ammonium as well as carbonate ions and in the presence of calcium ions which can precipitate calcium carbonate; this process is called “biocalcification” or microbial induced calcite precipitation (MICP).This technology is environmentally friendly not only because it gives strength to sand body, but also it allows water to penetrate to sand body, which is unlike silicate cement that will destroy the ecosystem of the earth. Calcium carbonate precipitated by bacteria acts as a binding material to sand particles, so incompact sand will be consolidated. Calcium chloride, calcium acetate and calcium nitrate (1 M) as calcium sources were tested for their ability to consolidate sand by mixing with urea (1 M) and bacteria cells (one optical density, 1 OD). The key point of this study aimed to choose the suitable calcium source which produces higher compressive strength and lower water absorption. The results showed that the degree of crystallinity and amount of precipitated calcium carbonate, as well as the consequent increase in strength of consolidated sand, in case of calcium chloride medium are higher than those precipitated in case of calcium acetate as well as calcium nitrate media. In addition, consolidated sand by calcium chloride was also used for cement mortar crack remediation.
Introduction
Microorganisms and microbially mediated mineralization processes are active in almost every environment on earth [Citation1,Citation2]. In natural environments, chemical CaCO3 precipitation () is accompanied by biological processes, both of which often simultaneously or sequentially occur. Microbes from soils and aqueous media have been frequently reported to induce the precipitation of calcium carbonate mineral phases in both natural and laboratory settings [Citation3]. Because of this, microbial activity is regarded as an important player in the formation of carbonate sediments and soil carbonate deposits [Citation4]. A number of studies have investigated carbonate mineralization induced by microbes [Citation5] including that by soil bacteria [Citation6–Citation8].
Microorganism-induced sand bioconsolidation has been previously considered for sand improvement [Citation9,Citation10]. The best studied mechanism is the ureolytic pathway in which microorganisms degrade urea by the intracellular enzyme urease, producing and NH3. The latter is converted into
, giving alkalinization of the medium, and
is converted into
. When the calcium ion is present and oversaturation to calcite is reached, calcium carbonate precipitation is induced by these microorganisms. The cohesion of sand particles by bioconsolidation is insufficient for use in ordinary constructional components. However, it can be very useful in geotechnical engineering to prevent or stabilize erosion and produce increased slope stability [Citation10]. Sporosarcina pasteurii [Citation11] is the most commonly used bacterial species for sand bioconsolidation.
For crack repair, a variety of techniques is available but traditional repair systems have a number of disadvantageous aspects such as different thermal expansion coefficient compared to concrete and environmental as well as health hazards. Therefore, bacterially induced calcium carbonate precipitation has been proposed as an alternative and environmentally friendly crack repair technique.
Microbiologically enhanced crack remediation (MECR) utilizes CaCO3 as a biological byproduct, which has shown a wide range of application potential as a sealant [Citation12,Citation13]. Its prospective applications include remediation of surface cracks and fissures in various structural formations, in-base and sub-base stabilization, as well as surface soil consolidation.
The objective of this investigation is to study the effect of different calcium sources on the physico-mechanical properties of microbial sand consolidation to facilitate utilization in soil stabilization and mortar crack remediation.
Experimental
Materials
Microorganisms
Bacteria species which is called S. pasteurii (previously known as Bacillus pasteurii) NCIMB 8841, obtained from the National Collection of Industrial and Marine Bacteria, England was used in this study. The aerobic organism is a moderately alkalophilic (growth optimum pH 9.25) and it was shown that sufficient activity for biocementation could be cultivated in non sterile conditions with a minimum of upstream and downstream processing. S. pasteurii, is a common bacterium naturally occurring in the subsurface, such as aerobic microorganism. In addition, cells of S. pasteurii are not aggregate; this ensures a high cell surface to volume ratio, a condition that is essential for efficient cementation initiation.
Culture media
S. pasteurii was cultivated under aerobic batch conditions in 20 g/l yeast extract and 10 g/l ammonium chloride. pH medium was adjusted to 9 by 4 N NaOH before autoclaving.
Sand and cement
The materials used in this investigation are ordinary Portland cement (OPC) which was provided by Helwan Cement Company, Egypt, Sand from El-Wasta Area, Beni Suif governorate, Egypt. The chemical and mineralogical compositions are given in and .
Table 1 Chemical composition of sand and cement, wt.%.
Methods of investigation
Sand consolidation
The S. pasteurii cells required for the treatment were initially grown on solid nutrient medium and transferred twice onto fresh medium to ensure purity of the culture. A single colony was then transferred to liquid medium, for 24 h at 37 °C under agitation, the cells were spun down in a centrifuge at 4000 cycle/min for 10 min. After centrifuging, the supernatant was removed. Cores for cementation consisted of 50 ml plastic syringe that were dry packed with 150–300 μm Si-sand under continuous vibration to give an even density then up flushed with water and tapped to remove air pockets. After flushing with water, the volume occupied by sand decreased due to the lubrication effect between the sand particles and stopper was adjusted to maintain a confining pressure. 100 ml of bacteria solution (1 OD) was firstly injected into the sands from top of syringe and a beaker was put under the injector to take it; when the solution was fully exudative, the mixture of (1 M) urea and (1 M) of each of calcium chloride, calcium acetate or calcium nitrate solutions followed. It is obvious that the solution in the beaker was still of use, at least was worked for a second time. Four successive treatments were done after 24, 48, 72 and 96 h for the first, second, third and fourth treatments, respectively.
Crack remediation
Cement mortar cubes with dimensions of 50.8 × 50.8 × 50.8 mm (2 × 2 × 2 in.) were cast according to the ASTM [Citation14] specification. A thin copper plate of 0.3 mm thickness was introduced in the fresh mortar paste up to a depth of 20 mm and length of 50 mm. Sand was injected into artificial cracks of cement mortar after 28 days curing at room temperature. During next 48 h, 10 ml of cells (1 OD) and equimolar (1 M) of urea/CaCl2 were injected into artificial crack at 12 h intervals.
Water absorption
The cores of consolidated sand were removed from syringe after four treatments then cured under tap water for 24 h. The water absorption measurements were done by weighing the saturated specimens (W1) and dried specimens in oven at 80 °C for 24 h (W2). The water absorption was calculated from the following equation: ASTM [Citation15]
Compressive strength
This test was carried out for four specimens following the procedure described by ASTM [Citation16]. The surface of the specimens was carefully polished on fine paper in order to remove irregularities. To help transmit the load to the specimen’s faces uniformly pieces of cardboard 2 mm thickness were placed on both faces. Compressive strength measurements were carried out using five tons German Brüf Pressing Machine with a loading rate of 100 kg/min.
X-ray diffraction (XRD)
The crystalline phases present in the prepared samples and mixes were identified using the X-ray diffraction technique. Nickel-filtered Cu-kα radiation at 40 kV and 20 mA was used throughout a Philips PW3050/60 diffractometer, provided with a proportional counter. A scanning speed of 2θ/min, and a chart speed of 10 mm/min, were used in all cases.
Thermo gravimetric analysis (TGA)
The apparatus used in the present study was TGA-50 Thermal Analyzer (Schimadzu Co. Tokyo, Japan). A dried sample of about 50 mg (−53 μm) was used with a heating rate of 20 °C/min under dynamic nitrogen atmosphere.
Differential thermal analysis (DTA)
Differential thermal analysis (DTA) was carried out by heating the sample in nitrogen atmosphere up to 1000 °C with a heating rate 20 °C/min using a DT-50 Thermal Analyzer (Schimadzu Co-Kyoto, Japan). The results were compared with DTA standard data [Citation17].
Scanning electron microscopy (SEM)
The scanning electron microphotographs were obtained with Inspect S (FEI Company, Holland) equipped with an energy dispersive X-ray analyzer (EDAX). It is used to examine the microstructure of the fractured composites at the accelerating voltage of 200 V to 30 kV and power zoom magnification up to 300,000×.
Results and discussion
Sand consolidation
Water absorption
The water absorption of consolidated sand by mixing with (1 OD) of bacterial cells and (1 M) equimolar urea/calcium chloride, calcium acetate or calcium nitrate after four treatments is graphically represented in . Comparing water absorption values of consolidated sand, it can be said that water absorption value of sand consolidated by bacterial cells and calcium chloride is lower than that consolidated by bacterial cells and calcium acetate or calcium nitrate. This is due to that the amount of calcium carbonate precipitated by bacterial cells and calcium chloride is greater than those precipitated in case of calcium acetate or calcium nitrate which leads to more filling of open pores and, therefore, decreases the water absorption. This result is confirmed by the values of weight loss obtained by TGA as reported later in this study –.
Compressive strength
The compressive strength of consolidated sand mixed with (1 OD) of bacterial cells and (1 M) equimolar of urea/calcium chloride, calcium acetate or calcium nitrate after four treatments graphically plotted against type of calcium source in . Considering the effect of calcium source on the compressive strength of microbial consolidated sand, the compressive strength value of consolidated sand treated by calcium chloride is greater than that treated with calcium acetate or calcium nitrate, respectively. This is attributed to the fact that, bacterial cells precipitated more amount of rhombohedral calcium carbonate in case of calcium chloride medium, as previously discussed and as shown later in the SEM micrographs of this investigation (), which binds sand particles together more than that of spherical and little amounts of rhombohedral calcium carbonate that precipitated in case of calcium acetate and calcium nitrate media. On the other hand, the consolidated sand in calcium nitrate medium gives the lower compressive strength than that of calcium chloride and calcium acetate; this is due to that the nitrate ion acts as an inhibitor for urease enzyme activity [Citation18] which reduces amounts of precipitated calcium carbonate on sand matrix and, therefore, decreases the compressive strength. Also, the compressive strength in case of calcium acetate is greater than that in case of calcium nitrate due to the amount of precipitated calcium carbonate in case of calcium acetate is greater than precipitated in case of calcium nitrate.
Fig. 3 Compressive strength of consolidated sand mixed with (1 OD) of bacterial cells and (1 M) equimolar of urea/calcium chloride, calcium acetate or calcium nitrate after four treatments.
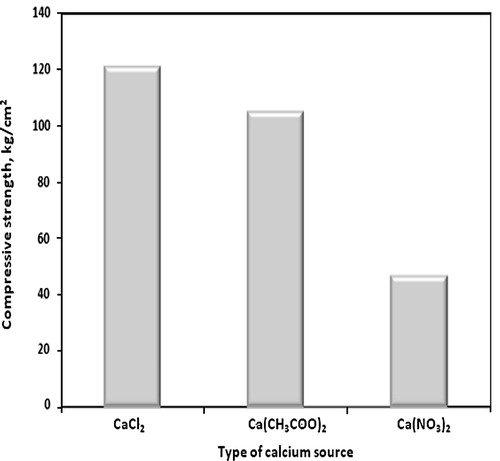
Fig. 8 SEM micrographs of consolidated sand by mixing with (1 OD) of bacterial cells and (1 M) of urea and (1 M) of different calcium sources; calcium chloride (a), calcium acetate (b) or calcium nitrate (c) at two different magnifications [(300 μm for a1, b1 and c1) and (50 μm for a2, b2 and c2)] after four treatments.
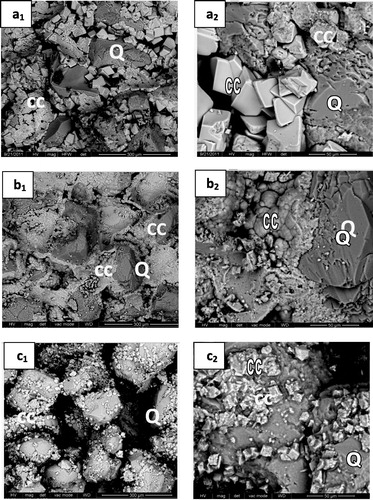
XRD analysis
presents the XRD diffractogram of consolidated sand by (1 OD) of bacterial cells and (1 M) equimolar of urea/calcium chloride, calcium acetate or calcium nitrate. However, the results of SEM examination (reported later in ) indicated that the amount of calcite crystals precipitated by bacterial cells and calcium acetate is more than that precipitated by bacterial cells and calcium nitrate; meanwhile, the XRD diffractogram () showed that the calcite peak of calcium carbonate precipitated by bacterial cells and calcium nitrate is more intense than that precipitated by bacterial cells and calcium acetate. This might be attributed to the higher degree of crystallinity of calcium carbonate formed by bacteria and calcium nitrate; XRD analysis gives an indication for the crystalline part of calcium carbonate, while the amorphous part is weakly detected. On the other hand, the intensity of the peaks characteristic for calcium carbonate crystals precipitated by bacterial cells and calcium chloride is stronger than that precipitated by bacterial cells and calcium nitrate; this is due to the decrease in activity of urease enzyme as retarded by nitrate ions [Citation18] leading to a decrease in the amounts of precipitated calcium carbonate crystals and, a consequent decrease in peak intensity of calcium carbonate.
Thermogravimetric analysis (TGA)
Figs. , and show the TGA thermograms for consolidated sand by (1 OD) of bacterial cells and (1 M) equimolar of urea/calcium chloride, calcium acetate or calcium nitrate, respectively. The TGA results showed that, the weight loss of consolidated sand calcium chloride set (12.66%) is greater than those of calcium acetate set (10.42%) or calcium nitrate set (6.68%). This is attributed to the larger amounts of calcium carbonate precipitated by bacterial cells and calcium chloride as compared to those precipitated by bacterial cells and calcium acetate or calcium nitrate.
Differential thermal analysis (DTA)
Figs. , and illustrate the DTA thermograms for consolidated sand by (1 OD) of bacterial cells and (1 M) equimolar of urea/calcium chloride, calcium acetate or calcium nitrate, respectively. The endothermic peaks at 47.12–63.46 °C are related to moisture in the consolidated sand; the endothermic peak located at 584.88–585.35 °C is mainly attributed to α → β quartz transformation. The exothermic peaks located at 342.93 and 427.83 °C are mainly due to decomposition of the organic acetate and the endothermic peaks at 215.17 as well as 472.50 °C are related to decomposition of organic matter from culture media [Citation19]. The endothermic peaks located at 730.63–812.87 °C are mainly attributed to decomposition of calcium carbonate. Obviously, the endotherms characteristic for calcium carbonate indicated that the precipitated calcium carbonate content of consolidated sand by bacterial cells and calcium chloride is higher than those of consolidated by bacterial cells and calcium acetate or calcium nitrate; this is clearly understood from differences in the shape and intensity of calcium carbonate endotherms shown in Figs. , and . In addition, the endothermic peaks of calcium carbonate are decomposed at a wider temperature range (730.63–812.87 °C) in case of calcium chloride set than those in case of calcium acetate set (791.15–807.17 °C) and calcium nitrate set (739.17 °C). This means that the calcium carbonate precipitated in case of calcium chloride is more crystalline than that formed in case of calcium acetate or calcium nitrate. This tends to decrease the water absorption and therefore, increase compressive strength. The exothermic peaks located at 918.63–930.52 °C is related to decomposition of pseudo-wollastonite (CS) phase [Citation17].
Scanning electron micrographs (SEM)
illustrates the SEM micrographs of consolidated sand (Q) by mixing with 1 OD of bacterial cells and (1 M) equimolar of urea/different calcium sources [CaCl2, Ca(CH3COO)2 or Ca(NO3)2]. The SEM micrographs displayed major variations in the morphology and microstructure of the calcite formed with different calcium sources. In case of calcium chloride the bacterial precipitated rhombohedral calcium carbonate (CC) as shown in at two different magnifications (a1:300 μm and a2:50 μm); in case of calcium acetate, spherical crystals of calcium carbonate (b1 and b2) were formed by enzymatic bacteria. Finally, little amounts of microcrystalline rhombohedral calcite crystals could be distinguished on the surface and within sand grains in calcium nitrate medium (c1 and c2); little holes also appeared on the surface of CaCO3 rhombohedral crystals (). These holes were interpreted to be the casts of former bacteria. It can be seen that, the number of open pores in case of calcium nitrate is greater than that in case of calcium chloride and calcium acetate. This is due to the fact that the activity of bacterial cells was retarded by nitrate ions [Citation18] which leads to the precipitation of little amounts of calcium carbonate and, therefore, increase the extent of open pores and the subsequent decrease in compressive strength as shown in . On the other hand, the size of calcite crystals formed in case of calcium chloride is greater than those formed in case of calcium acetate and calcium nitrate. This leads to precipitate more calcium carbonate in the open pores as previously discussed.
Mortar crack remediation
The compressive strength of untreated (C-1) and treated (C-2) crack mortar specimens, by mixing with (1 OD) of bacterial cells and equimolar (1 M) of urea/(1 M) of calcium chloride, and control cement mortar (C-0) is graphically represented in . It was found that compressive strength of untreated crack (C-1) mortar is lower than that of control sample (C-0) by 43% which improved after remediation (C-2) leading to a lowering in strength of 10% than that of control sample. This is mainly due to chemical bonding between CaCO3 precipitated by bacterial cells and sand particles which consolidate the crack space.
Conclusion
1. | The consolidated sand by bacterial cells and urea/calcium chloride gives best physico-mechanical properties (higher compressive strength and lower water absorption) than those consolidated by bacterial cells and urea/calcium acetate or urea/calcium nitrate. | ||||
2. | The size, morphology and degree of crystallinity of precipitated calcite by bacterial cells depend on the calcium source used. | ||||
3. | The amount and degree of crystallinity of precipitated calcite by bacterial cells in case of calcium chloride are greater than those precipitated in case of calcium acetate or calcium nitrate. | ||||
4. | Nitrate ions cause retardation of urease activity of bacterial cells. | ||||
5. | The phenomena of sand consolidation by bacterial cells can be utilized in mortar crack remediation. |
Notes
Peer review under responsibility of Housing and Building National Research Center.
References
- P.Lopez-GarciaJ.KazmierczakK.BenzeraraS.KempeF.Guyot MoreiraBacterial diversity and carbonate precipitation in the giant microbialites from the highly alkaline Lake Van, TurkeyExtremophiles92005263274
- Y.ShenR.BuickD.E.CanfieldIsotopic evidence for microbial sulfate reduction in the early Archaean eraNature41020017781
- B.LianQ.HuJ.ChenJ.JiH.H.TengCarbonate biomineralization induced by soil bacterium Bacillus megateriumGeochim. Cosmochim. Acta70200655225535
- M.A.RivadeneyraG.DelgadoA.Ramos-CormenzanaR.DelgadoBiomineralisation of carbonates by Halomonas eurihalinain solid and liquid media with different salinities: crystal formation sequenceRes. Microbiol.1491998277287
- D.T.WrightA.OrenNon-photosynthetic bacteria and the formation of carbonates and evaporates through timeGeomicrobiology2220052753
- P.CacchioC.ErcoleG.CappuccioA.LepidiCalcium carbonate precipitation by bacterial strains isolated from a limestone cave and from a loamy soilGeomicrobiology2020038599
- M.A.RivadeneyraG.DelgadoA.Ramos-CormenzanaR.DelgadoPrecipitation of carbonates by Deleya halophila in liquid media: pedological implications in saline soilsArid Soil Res. Rehabil.1119973549
- S.Stocks-FischerJ.K.GalinatS.S.BangMicrobiological precipitation of CaCO3Soil Biol. Biochem.3111199915631571
- V.S.WhiffinL.van PaassenM.P.HarkesMicrobial carbonate precipitation as a soil improvement techniqueGeomicrobiology242007417423
- J.T.DeJongB.M.MortensenB.C.MartinezD.C.NelsonBio-mediated soil improvementEcol. Eng.362010197210
- P.M. Harkes, J.L. Booster, L.A. van Paassen, M.C.M. van Loosdrecht, Conference on Bio-Geo-Civil Engineering, Netherlands, 2008, pp. 37–41.
- S.S.BangJ.K.GalinatV.RamakrishnanCalcite precipitation induced by polyurethane-immobilized Bacillus pasteuriiEnzyme Microb. Technol.282001404409
- KimVan TittelboomNeleDe BelieWillemDe MuynckWillyVerstraeteUse of bacteria to repair cracks in concreteCem. Concr. Res.402010157166
- ASTM C 305. Standard Practice for Mechanical Mixing of Hydraulic Cement Pastes and Mortars of Plastic Consistency, 2011.
- ASTM C1506. Standard Test Method for Water Retention of Hydraulic Cement-Based Mortars and Plasters, 2009.
- ASTM C109M. Standard Test Method for Compressive Strength of Hydraulic Cement Mortars, 2007.
- V.S.RamachandranApplication of Differential Thermal Analysis Cement Chemistry1969Chemical Publishing Company, Inc.New York pp. 55–80
- V.S. Whiffin, Microbial CaCO3 Precipitation for Production of Biocement, Ph.D. Thesis, Mourdoch University, Australia, 2004.
- Márcia AikoShirakawaKatia KaoriKaminishikawaharaVanderley MoacyrJohnHenriqueKahnMarcos MassaoFutaiSand bioconsolidation through the precipitation of calcium carbonate by two ureolytic bacteriaMater. Lett.65201117301733