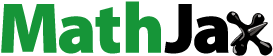
Abstract
Solid solutions of (1−x)BaTiO3-xBaSc1/2Nb1/2O3 (BT-BSN) with x = 0.025, 0.05, 0.075, 0.1 and 0.125 were prepared by a high temperature solid-state reaction technique. The effects of the Ba(Sc1/2Nb1/2)O3 addition on the phase composition, dielectric properties, as well as polarization-electric field (P-E) loops of the BT-BSN solid solution were investigated. The room-temperature X-ray diffraction analyses of all the ceramics revealed a perovskite phase after sintering at 1350 °C with a composition-dependent symmetry. Temperature and frequency dependence of the dielectric permittivity and losses have been explored: ceramics of compositions x ≤ 0.075 showed normal ferroelectric behavior, while ceramics with x ≥ 0.1 were of relaxor type. The degree of diffuseness and the relaxor effect increase while the transition temperature (TC or Tm) decreases when both scandium and niobium are introduced in the BaTiO3 lattice. Ceramics of composition x = 0.125 exhibited interesting relaxor characteristics at 10 kHz: ΔTm = 20 K, ɛr = 12,000, and Tm = 140 K. In addition, modeled using Vogel–Fülcher relation, this same composition showed the fitting parameters: Ea = 0.0503 eV, f0 = 1.129 × 1014 Hz and TVF = 166.85 K.
1 Introduction
Materials with a perovskite structure of general formula ABO3 have been found to be very interesting owing to their applications in different solid-state devices. The incorporation of other ions in the ABO3 lattice gives rise to perovskite compounds of complex formula, such as AA′BO3, ABB′O3, AA′BB′O3, etc. (A and A′ are monovalent, divalent or trivalent ions located in the dodecahedral site; B and B′ are divalent, trivalent, tetravalent or pentavalent ions located in the octahedral site). Numerous authors have devoted their investigations to the lead complex perovskite with disordered cation arrangements such as Pb(Mg1/3Nb2/3)O3-PbTiO3 (PMN-PT), Pb(Sc1/2Nb1/2)O3-PbTiO3 (PSN-PT), etc. These so-called relaxor ferroelectrics are distinguished by their particular dielectric performance, characterized by a broad maximum with frequency dispersion, and the temperature of the dielectric permittivity maximum shifts to higher values with increasing frequency. This relaxor behavior is related to the existence of ions with different valence states at the B-sites of the ABB′O3 perovskite structure. The statistical fluctuations of ions at the B-sites lead to local heterogeneity and large variations in the Curie temperature [Citation1,Citation2]. In addition, these materials present an interesting region, the so-called morphotropic phase boundary (MPB) [Citation3]. The subsistence of ferroelectric properties close to or within the MPB made these materials excellent candidates for applications as capacitors and actuators [Citation4,Citation5]. Actually, the majority of relaxor materials are lead-based ceramics. The latter present a drawback due to toxicity and volatility of PbO. Therefore, to develop environment friendly materials, a great deal of lead-reducing compositions and lead-free materials was examined. In this view, partial substitution of Pb2+ by Ba2+ has shown interesting dielectric performances, as observed for instance in the Ba(B1/3B′2/3)O3-PbTiO3 or Ba(B1/3B′2/3)O3-PbTiO3 solid solutions [Citation6–Citation14]. Among them, solid solution (1−x)Ba(Mg1/3Nb2/3)O3-xPbTiO3(BMN-PT) [Citation7] and (1−x)Ba(Sc1/2Nb1/2)O3-xPbTiO3(BSN-PT) [Citation13] have revealed typical relaxor ferroelectric behavior. The MPB, in which the rhombohedral phase coexists with the tetragonal phase, changes from 70–73 mol% PT (BMN-PT) to 61–65 mol% PT (BSN-PT), and the dielectric permittivity is enhanced greatly by increasing the PT content. However, these compositions present relatively high lead content and therefore were not suitable for environmental devices. Thus, in order to develop environment-friendly materials, various studies of lead-free comparable systems have been achieved [Citation15–Citation21].
In this work, we present a similar system with fully lead-free compositions. Our study concerns the (1−x)BaTiO3-xBaSc1/2Nb1/2O3 solid solution with x = 0.025, 0.05, 0.075, 0.1 and 0.125, further denoted, respectively, as BT-BSN25, BT-BSN50, BT-BSN75, BT-BSN100 and BT-BSN125. The choice of Ba(Ti1−xScx/2Nbx/2)O3 compositions was stimulated by their likeness to numerous lead-based relaxor ferroelectrics in which the degree of order in A(B1/2B′1/2)O3 complex perovskites depends on the differences between the ionic charges and the radii of B and B′ cations [Citation22]. In our case, the difference in the charge and ionic radius of Sc3+ (r = 0.745 Å), Nb5+ (r = 0.640 Å) and Ti4+ (r = 0.605 Å) [Citation23] introduces a compositional heterogeneity favorable to relaxor behavior. Contrary to BSN-PT or BMN-PT systems, we have explored lead-free compositions close to BaTiO3. The structural, dielectric and ferroelectric properties were investigated and discussed.
2 Materials and methods
The materials under investigation were synthesized via the conventional solid-state reaction route. The starting materials were powders of BaCO3, TiO2, Nb2O5 and Sc2O3 (all Sigma–Aldrich, USA). Stoichiometric amounts of constituent chemicals were milled in isopropanol medium in attrition mill at 600 rpm for 2 h, using polyethylene vial and yttrium-stabilized zirconia (YSZ) millings with a diameter of 3 mm. The homogenized powder mixtures were dried for 3 h at 100 °C.
The mixture was then calcined in oxygen atmosphere at 1300 °C for 2 h at a heating and cooling rate of 5 °C/min. Prior to further processing the as-calcined powders were again attrition-milled at 600 rpm for 3 h. The sintering curve of these powders was recorded using a heating-stage microscope (LeitzWetzlar, Germany) in the temperature range between 25 and 1400 °C at a heating rate of 10 °C/min in air. The obtained powders were isostatically pressed at 300 MPa into compact disks of about 8 mm diameter. The resulting powder compacts were sintered in oxygen flow at 1350 °C for 2 h at heating and cooling rates of 5 °C/min in a platinum crucible.
The densities of sintered ceramics were determined from the liquid displacement technique based on the Archimedes principle.
To determine phase composition, symmetry and unit cell parameters, X-ray powder diffraction was used. Diffraction data were collected at room temperature by a PaNalytical X’Pert PRO MPD diffractometer (Almelo, Netherlands) using CuKα1 radiation (λ = 1.5406 Å) in the 2θ range from 18° to 108° in steps of 0.02° with 10 s counting time for each step.
For electrical characterization, Cr/Au electrodes with diameter of 6 mm were magnetron sputtered (5 Pa) on approximately 1-mm thick samples. The dielectric permittivity and the dielectric losses were measured on cooling in the 102–106 Hz frequency range, using an HP4192A meter (Yokogawa-Hewlet-Packard, Tokyo). Amplitude of the probing AC electric field was 1 V. Ferroelectric hysteresis loops were measured at room temperature with a conventional computer interfaced Sawyer–Tower circuit at a frequency of 30 Hz.
3 Results and discussion
3.1 Sintering and structural analysis
To specify the optimal sintering temperature, a sintering curve of the BT-BSN100 powder compact was measured (). The highest sintering rate was observed between 1300 and 1400 °C; therefore, for all the samples the sintering temperature was found to be equal, at 1350 °C. Relative densities of the sintered ceramics obtained by the Archimedes method are presented in . The theoretical density was calculated from the known phase composition and unit cell volume. The densities of the specimens are in the range between 96% and 98% of the theoretical density.
Table 1 Structure and lattice parameters of different compositions in the (1−x) BaTiO3-xBaSc1/2Nb1/2O3 system.
The X-ray diffraction profiles of all the samples have revealed that no parasite phase exists. Only peaks corresponding to the perovskite phase are present (). The obtained XRD patterns were subjected to cell and profile parameters refinement using a global profile-matching method with “fullprof” software [Citation24]. The structure and lattice parameters for (1−x)BaTiO3-xBaSc1/2Nb1/2O3 are listed in . It is notable that as Sc/Nb doping in BaTiO3 increases, the c lattice parameter decreases and the a lattice parameter increases, while the unit cell volume increases steadily. In addition, the c/a values are found to become smaller by the partial substitution of Ti4+ by Sc3+ and Nb5+, indicating the formation of a more typical cubic phase. These evolutions could be related to the values of ionic radii: both () and (
), which are quite higher than (
) [Citation23].
3.2 Dielectric studies
The dielectric properties, e.g., dielectric permittivity (ɛr) and loss (tan δ) of the solid solution (1−x)BaTiO3-xBaSc1/2Nb1/2O3 (x = 0.05, 0.075, 0.1 and 0.125), were measured as functions of both temperature and frequency. The thermal and frequency variations of the dielectric permittivity and dielectric losses as well as the temperature variation of 1/ɛr at 10 kHz exhibited different behaviors depending upon the substitution rate (–).
For the composition BT-BSN50, three phase transitions are clearly observed in the dielectric permittivity vs. temperature curve ((a)), most probably corresponding to the phase transitions from rhombohedral to orthorhombic (254 K), orthorhombic to tetragonal (292 K) and tetragonal to cubic (338 K), respectively. All these change sequences were distinctive of the classical (or normal) ferroelectric materials. The dielectric peak at the Curie temperature (TC = 338 K) is very sharp and was not dependent on the frequency.
Fig. 3 Temperature and frequency dependence of the dielectric permittivity and loss tangent of the compositions with (a) x = 0.05 and (b) x = 0.075.
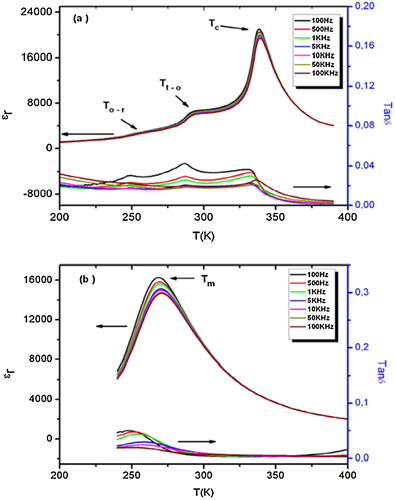
For BT-BSN75 (i.e., niobium and scandium content increased), there is only one transition at 269 K ((b)), which could be related to the ferroelectric–paraelectric phase transition.
The compositions with x = 0.1 and 0.125 (i.e., BT-BSN100 and BT-BSN125) show broad peaks of dielectric permittivity with frequency dispersion ((a) and (b)). With the increase in frequency, the maximum of dielectric permittivity decreases, while the resultant maximum temperature shifts towards high values, indicative of relaxor ferroelectric behavior.
Fig. 4 Temperature and frequency dependence of the dielectric permittivity and loss tangent of the composition with (a) x = 0.1 and (b) x = 0.125.
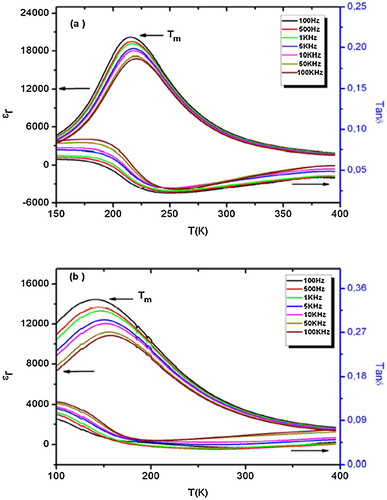
The thermal variation of dielectric constant above the Curie temperature (in paraelectric phase region) can be described generally by a Curie–Weiss law, Eq. Equation(1)(1)
(1) :
(1)
(1) where T0 is the Curie–Weiss temperature and C is the Curie–Weiss constant. In fact, shows the plot of the inverse of ɛr as a function of temperature at 10 kHz and the fit according to the Curie–Weiss law. The fitting parameters (C and T0) are given in .
Table 2 Summary of some physical parameters of the BT-BSN ceramics.
Fig. 5 Thermal variation of 1/ɛr at 10 kHz for compositions (a) x = 0.05, (b) x = 0.075, (c) x = 0.1 and (d) x = 0.125 (blue curve are the experimental data; red line, the fitting to the Curie–Weiss law).
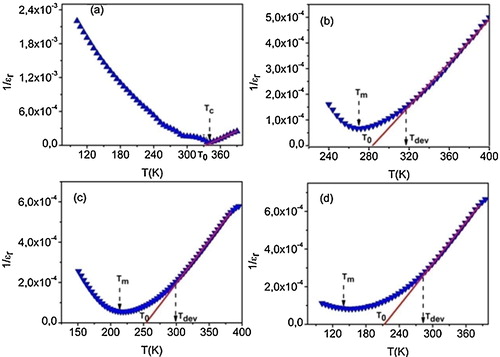
It can be seen that the temperature dependence of 1/ɛr for BT-BSN50 ((a)) is in good agreement with the Curie–Weiss law in the paraelectric region (T > TC). However, the broadened peaks for 0.075 ≤ x ≤ 0.125 indicated that the transitions were of the diffuse type, which is confirmed by the deviation from the Curie–Weiss law in these compositions ((b)–(d)), the value of the Curie–Weiss temperature T0 was greater than that of Tm. The dielectric permittivity follows the Curie–Weiss law at T > Tdev (Tdev represents the temperature at which ɛr starts to deviate from the Curie–Weiss law). Furthermore, the relaxor character appears at low temperatures and the values of the maximum permittivity decrease with the increase in Sc/Nb concentration. Nevertheless, these values remain relatively important, probably owing to the contribution of electronic and ionic conductivities in these materials.
Moreover, the investigation on the ferroelectric relaxor process has given rise to various physical and/or structural interpretations. In our compositions, the relaxor behavior can be explained on the basis of compositional fluctuation and/or random local electric fields and stress-induced phase transition due to Sc3+, Nb5+ and Ti4+ ions distributed randomly in the B-site [Citation25,Citation26]. Thus, a modified Curie-Weiss law involving a parameter noted γ was used to describe the diffuseness of the broadened peaks in the paraelectric phase [Citation27,Citation28]; it is defined by Eq. Equation(2)(2)
(2) below:
(2)
(2)
In our case, the plots of ln(1/ɛr − 1/ɛm) vs. ln(T − Tm) at 10 kHz for the compositions x ≥ 0.05 showed nearly linear variation (). For different compositions x, the mean value of the diffusivity γ was extracted from these plots by fitting a straight-line equation. We noted that γ increases from 1.40 to 1.92 with the increased doping level (). This result indicated the existence of relaxor behavior for compositions with x ≥ 0.1.
Moreover, to describe the diffuseness of the phase transition, a parameter ΔT was used [Citation27]; Eq. Equation(3)(3)
(3) :
(3)
(3) The values of ΔT for BT-BSN compositions are reported in . ΔT increases with the rise in x and then reaches the highest value for BT-BSN125 (ΔT = 144 K), which confirms the composition-induced diffuse phase transition behavior in the BT-BSN ceramics with x ≥ 0.1.
Moreover, to quantify frequency dispersion of Tm (or relaxation degree), a parameter ΔTm was defined by Eq. Equation(4)(4)
(4) [Citation29]:
(4)
(4) The values of ΔTm for all the studied samples are determined from the permittivity data and presented in . These data further confirmed that the compositions with x ≥ 0.1 belong to the relaxor ferroelectrics.
All the characterizations done above on the basis of the Curie–Weiss law and the value of the empirical parameters such as ΔT, γ and ΔTm have confirmed that the ceramics with x ≤ 0.075 were normal ferroelectrics, while those with x ≥ 0.1 were of the relaxor type. On the contrary, increase in ΔTm has been observed and consequently, the relaxor effect was amplified when the composition has deviated from BaTiO3. Such results have also been obtained for other lead-free perovskite relaxors [Citation30,Citation31].
The relaxor ferroelectric behavior could be equally described by the Vogel–Fülcher relation [Citation25,Citation32]; Eq. Equation(5)(5)
(5) :
(5)
(5) where f0 is the attempt frequency, Ea is a measure of average activation energy, kB is the Boltzmann constant and TVF is the freezing temperature.
shows the plot of ln(f) vs Tm for the sample BT-BSN125. The experimental curve was fitted using the above Vogel–Fülcher formula. The fitting parameters of Vogel–Fülcher relation were Ea = 0.0503 eV, f0 = 1.129 × 1014 Hz and TVF = 166.85 K. As shown in this figure, the model accounts well for the data, suggesting that the relaxor behavior in the present composition is similar to that of a spin glass model with polarization fluctuations beyond the static freezing temperature. One of the characteristics of the dipolar glass system as well as relaxor ferroelectrics is the appearance of a low-frequency dielectric loss peak below Tm. The loss peaks shift to lower frequencies on decreasing the temperature, indicating the thermally activated nature of the dielectric relaxation [Citation29]. This phenomenon can be observed clearly for BT-BSN0.125 composition (). By analogy with spin glasses, relaxor behavior is related to the existence of the broad range of relaxation times. In fact, the Debye model is characterized by a single relaxation time, whereas in relaxor there is a distribution of relaxation times. Therefore, the local environment seen by individual dipoles differs from site to site. This leads to a very large dispersion, which is reminiscent of that found for orientational glasses.
Fig. 7 Plot of log (f) as a function of Tm for BT-BSN125 sample (the symbols: experimental data; the red dashed curve: fitting to the Vogel–Fülcher relation).
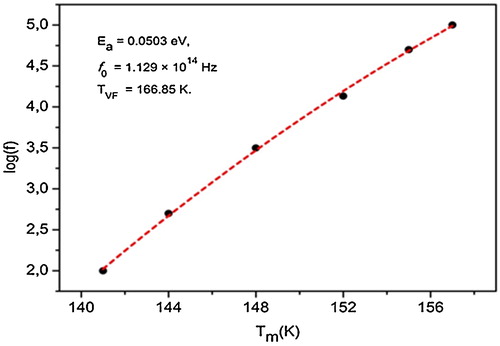
Although BT-BSN and BSN-PT systems seem to be similar, they present some important differences:
- | For lead BSN-PT compositions, i.e. [(1 − y)BaSc1/2Nb1/2O3 − yPbTiO3], the ferroelectric and particularly the relaxor effect (0.53 ≤ y ≤ 0.58) appear for the high concentration of BSN and PT. Therefore, despite their interesting dielectric characteristics, they were not suitable for environmental devices. |
- | The lead-free BT-BSN compositions, i.e. [(1 − x)BaTiO3-xBaSc1/2Nb1/2O3], exhibit ferroelectric and relaxor behavior for a weak content of BSN (0.025 ≤ x ≤ 0.125). |
3.3 Ferroelectric properties
Polarization-electric field (P-E) measurements of the BT-BSN ceramics were performed at room temperature and 30 Hz (). It is clearly evident that the shape of the P-E loops and the polarization values are significantly influenced by the ceramic compositions.
The loop of the BT-BSN50 sample shows larger hysteresis compared to the loops of the BT-BSN75, BT-BSN100 and BT-BSN125 samples. The values of remnant polarization Pr and coercive field Ec can be evaluated from .
The composition BT-BSN50 exhibits a well-saturated hysteresis loop with a remnant polarization Pr of 8.057 μC/cm2. As x increases, the loop starts to tilt and becomes slim. In these compositions (BT-BSN100 and BT-BSN125), the remnant polarization is practically zero. These results are in agreement with the crystal symmetry of the samples at room temperature, which is tetragonal in the BT-BSN50 and paraelectric cubic phase with Tm below room temperature for the compositions with x ≥ 0.075.
4 Conclusions
Dense and XRD phase-pure (1−x)BaTiO3-xBaSc1/2Nb1/2O3 ceramics, with x ranging from 0.025 to 0.125, have been synthesized using the conventional solid-state reaction route. The room temperature XRD data refinement revealed a tetragonal and cubic perovskite-type structure. For x ≤ 0.075, a typical ferroelectric to paraelectric phase transition is observed, while for x ≥ 0.1 the phase transition becomes diffuse and shows a relaxor behavior. The maximum of the permittivities (about 12,000) was higher than that of BSN-PT or BMN-PT compositions. In return, the temperature of the maximum dielectric permittivity Tm was lower and decreased with increasing values of x. The temperature of the maximum dielectric permittivity Tm decreased with increasing values of x. For the composition BT-BSN125, the frequency dependence of Tm is in good agreement with the Vogel–Fülcher model. This behavior may be attributed to the microscopic cluster disorder originating from the simultaneous substitution of Ti by Nb and Sc.
The optimal remnant polarization Pr of 8.057 μC/cm2 was obtained at x = 0.05. In particular, Pr decreases with increasing (Sc-Nb) content and tends to be equal to zero for the compositions with x ≥ 0.075.
Such lead-free ceramics are of interest for environmental preservation and device applications.
Acknowledgement
The authors wish to express their sincere thanks to the Electronic Ceramics Department of Jožef Stefan Institute for the use of their facilities.
Notes
Peer review under responsibility of The Ceramic Society of Japan and the Korean Ceramic Society.
References
- L.E.CrossFerroelectrics761987241267
- G.A.SmolenskiiA.I.AgranovskayaSov. Phys. Solid State1195914291437
- B.JaffeW.R.CookH.JaffePiezoelectric Ceramics1971Academic PressLondon
- L.E.CrossFerroelectrics1511994305320
- K.UchinoFerroelectrics1511994321330
- E.D.PolitovaV.F.ChuprakovNeorg. Mater.211985473
- X.LongZ.G.YeChem. Mater.19200712851289
- X.LongZ.-G.YeAppl. Phys. Lett.902007112905
- X.F.LongZ.-G.YeJ. Appl. Phys.10120071
- X.F.LongZ.-G.YeIEEE Trans. Ultrason. Ferroelectr. Freq. Control5420072595
- Y.C.LiouY.C.ShihC.J.ChuangY.T.ChenCeram. Int.34200814131418
- Z.J.WangZh.X.LiQ.WeiX.F.LongZ.-G.YeJ. Appl. Phys.1042008046102
- Q.WeiZ.WangX.LiX.LongZ.G.YeChem. Mater.2132009506510
- Z.WangX.LiX.LongZ.G.YeScr. Mater.602009830833
- Z.AbdelkafiN.AbdelmoulaH.KhemakhemO.BidaultM.MaglioneJ. Appl. Phys.1002006114111
- Z.AbdelkafiN.AbdelmoulaH.KhemakhemR.Von Der MuhllL.BihJ. Alloys Compd.4272007260266
- L.KhemakhemA.MaalejA.KabadouA.Ben SalahA.SimonM.MaglioneJ. Alloys Compd.4522008441445
- L.KhemakhemA.KabadouA.MaalejA.Ben SalahA.SimonM.MaglioneJ. Alloys Compd.4522008451455
- Z.AbdelkafiN.AbdelmoulaH.KhemakhemA.SimonM.MaglionePhys. Status Solidi205200829482952
- F.BoujelbenF.BahriC.BoudayaA.MaalejH.KhemakhemA.SimonM.MaglioneJ. Alloys Compd.4812009559562
- Z.WangX.M.ChenSolid State Commun.1512011708711
- A.A.BokovFerroelectrics18319966573
- R.D.ShannonActa Crystallogr.A321976751767
- J.Rodriguez-CarvajalProgram FullProf, Laboratoire Léon Brillouin, (CEA-CNRS), Version 3.5d, LLB-JRC1998
- H.VogelZ. Phys.221921645646
- X.G.TangX.X.WangK.ChewH.L.W.ChanSolid State Commun.13620058993
- K.UchinoS.NomuraFerroelectr. Lett. Sect.4419825561
- T.BadapandaS.K.RoutS.PanigrahiT.P.SinhaCurr. Appl. Phys.92009727731
- T.BadapandaS.K.RoutS.PanigrahiT.P.SinhaBull. Mater. Sci.312008897901
- A.SimonJ.RavezFerroelectrics240200016011608
- A.KerfahK.TaïbiA.Guehria-LaïdoudiA.SimonJ.RavezSolid State Sci.82000613618
- G.FulcherJ. Am. Ceram. Soc.81925339355