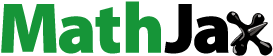
Abstract
Bismuth chalcogenides (Bi2X3, X = S, Se, Te) were synthesized from the elements under hydrothermal condition. Single phases of Bi2S3 and Bi2Se3 were produced at 240 °C. Bi2Te3 was obtained as an almost single phase with a small amount of Bi2TeO5. From the results of control experiments without water, the hydrothermal condition in the production of Bi2S3 was more effective than that of Bi2Se3. The particles of obtained Bi2S3 were spherical, with a diameter of ∼1 μm. The Bi2Se3 particles were in a plate-like form, with a width of ∼1 μm and a thickness of ∼0.2 μm.
1 Introduction
Bismuth chalcogenides (Bi2X3, X = S, Se, Te) are semiconductor compounds that have gained attention for use in photovoltaics [Citation1], thermoelectric devices [Citation2–Citation4], photoconductive materials [Citation5], electrochemical hydrogen storages [Citation6,Citation7], and topological insulators [Citation8]. In conventional synthesis techniques, elemental bismuth and chalcogens are reacted at high temperature under vacuum-sealed conditions, or hydrogen chalcogenide is purged into the aqueous metal salt solutions.
However, in recent years, synthesis using a hydrothermal method is becoming more common. For example, a Bi2S3 nanowire has been successfully synthesized using the hydrothermal method with thioglycolic acid [Citation9]. It was also reported that the synthesized Bi2S3 nanowire was very long, being a few millimeters in length and 60 nm in diameter [Citation10]. The synthesis of nano-structured flowers of Bi2S3 particles is also possible using the hydrothermal reaction of bismuth nitrate pentahydrate and thiourea in nitric acid [Citation11]. Furthermore, it has also been reported that the shape of a particle of Bi2S3, synthesized under hydrothermal conditions, is largely dependent on the type of sulfur source [Citation12]. For Bi2Se3, it has been reported that, to synthesize nanotubes and nanosheets, BiCl3 and hydrazine hydrate can be reacted in an H2SeO3 solution under hydrothermal conditions [Citation13]. Moreover, it has also been reported that reactions of NaOH, Se powder, hydrazine hydrate, Bi2(NO3)3•5H2O, and triethanol amine can be used to synthesize Bi2Se3 nanorods [Citation14]. For Bi2Te3, synthesis techniques for Bi2Te3 particles with nanostructures have been reported using the reaction of NaOH, Te powder, hydrazine hydrate, and BiCl3 [Citation15], or the reaction of Bi(NO3)3, TeCl4, and KBH4 [Citation16].
The hydrothermal synthesis technique can be used to synthesize substances at low temperatures, but, in many cases, the raw materials required are complex compounds, such as the metal salts described above. In contrast, we have studied a simple hydrothermal reaction using only an elemental metal and an elemental chalcogen as starting materials. In this manner, we have succeeded in synthesizing the binary metal sulfides of CuS [Citation17,Citation18], Cu2S [Citation18], Ag2S [Citation18], NiS [Citation19], Co9S8 [Citation19], Ni3S2 [Citation19], ZnS [Citation20], and CdS [Citation20], and the complex ternary metal sulfides of Ag3CuS2 [Citation21] and AgCuS [Citation21]. Among these sulfides, we have observed interesting particle shapes, such as polyhedral CuS and Cu2S. We considered whether this simple approach can possibly be applied to the synthesis of Bi2X3 (X = S, Se, Te).
Therefore, in this study, we attempted the synthesis of Bi2X3 (X = S, Se, Te) using the raw materials of elemental bismuth and elemental chalcogens under hydrothermal conditions. We also evaluated the products with X-ray diffraction (XRD) measurements and scanning electronic microscopy (SEM) observations.
2 Experimental
Bismuth, sulfur, selenium, and tellurium were used as starting materials. Bismuth (5 mmol) and chalcogen (7.5 mmol) powders were weighed and ground in an agate mortar. The mixtures were added to a Teflon-lined pressure vessel with a capacity of 23 mL (Parr, 4749), which was filled with 10 mL of distilled water. Then the pressure vessel was sealed and heated over a temperature range 180–240 °C (at intervals of 30 °C) for 10 h. After heating, the pressure vessel was cooled to room temperature. The precipitates were filtered and washed with distilled water several times. After drying in a desiccator for 12 h, the powder was collected for characterization. In order to clarify an effect of water, control experiments were performed without water. Powder XRD patterns were measured with Cu Kα radiation on a RINT2200 diffractometer (Rigaku) equipped with a graphite monochromator. The morphology and particle size of the products were observed via FE-SEM images, taken with an S-4500 system (Hitachi).
3 Results and discussion
3.1 Bi2S3
shows the XRD patterns of the products. In the 240 °C case, the Bi2S3 was produced as a single phase. The yield was 97%. Since the pH of the liquid after the reaction became rather acidic at 1.3, it is thought that part of the dissolved sulfur in the raw materials reacted with water like the following [Citation22]:(1)
(1)
Fig. 1 The XRD patterns of the Bi2S3 products produced at (a) 240 °C, (b) 210 °C, (c) 180 °C, (d) 240 °C without water, and (e) 210 °C without water. (f) The reported pattern of Bi2S3 (ICSD no. 617019). Inverted triangles and circles show positions of main peaks of Bi and S, respectively.
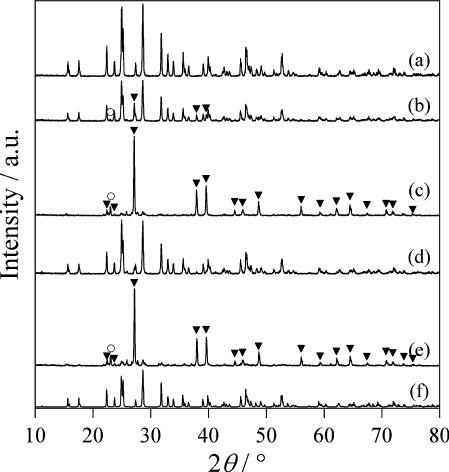
Even in the 210 °C case, although the Bi2S3 was mostly produced as a single phase, some peaks of unreacted starting materials, such as Bi and S were observed at the positions indicated by inverted triangles and circles, respectively, in . At 180 °C, the Bi2S3 was hardly generated; the raw materials of Bi and S were observed as the main components; hence, it was found that the reaction had barely progressed. Next, in order to clarify the effect of the hydrothermal conditions, we performed control experiments without water. The results are shown in . In the 240 °C case, even in the absence of water, the major product was Bi2S3, but some starting raw material of Bi was observed. At 210 °C, almost no Bi2S3 was generated when there was no water. From these results, we come to know that the hydrothermal conditions are effective in the production of Bi2S3. Similar results were obtained had in the cases of CuS [Citation17] and ZnS [Citation20].
shows SEM images of the products. At 240 °C, the Bi2S3 particles produced became spherical; the size was ∼1 μm. Even at 210 °C, since the produced Bi2S3 was mostly as a single phase, particles with similar shapes were seen. However, at 180 °C, Bi2S3 was not produced; the spherical particles seen at 210 °C and 240 °C were not found. We know that the shape of Bi2S3 is close to that of the particles of the Bi raw material. At 240 °C, in the control experiments without water, Bi2S3 was produced as the main component. However, the shape of the particle was found to be different from the nearly spherical particle shape under hydrothermal conditions. From this fact, it was clear that the hydrothermal conditions not only increased the reactivity, but also greatly changed the particle shape. This result was consistent with previous results for hydrothermal synthesis of CuS [Citation17]. Because unreacted Bi was the main component in the case of 210 °C without water, it is understood that it has a particle shape close to that of the Bi raw material, as in the hydrothermal case at 180 °C.
Fig. 2 SEM images of the Bi2S3 products produced at (a) 240 °C, (b) 210 °C, (c) 180 °C, (d) 240 °C without water, and (e) 210 °C without water. SEM images of the starting materials (f) Bi and (g) S.
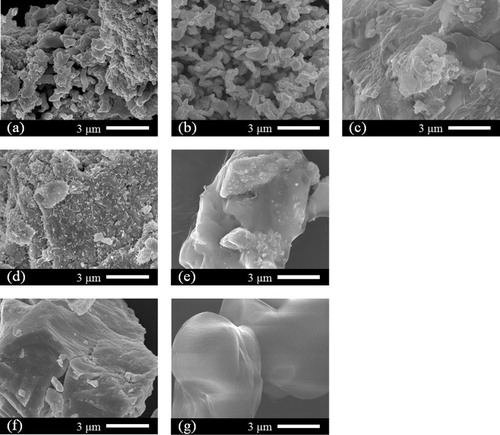
The role of water for the reaction between elemental copper and sulfur is known well [Citation17]. Copper reacts with sulfur to form CuS at near ambient temperatures (≥46 °C) [Citation23]. However, the reaction is restricted to the surface of the particles. The formation of surface CuS layers on the copper reactant limits interdiffusion of the copper and sulfur reactants, thus passivating the surface. Because unreacted metal copper is observed with CuS phase at low temperatures [Citation23], higher temperatures (>165 °C) are required to enhance diffusion and yield phase-pure CuS in the reaction. In water, however, the reaction proceeds to completion at much lower temperatures (60 °C for 10 h) [Citation17]. Here water provides a medium to continuously transport sulfur to the reactant copper surface, thereby avoiding passivation and completing the reaction at low temperatures. This passivity prevention and breakdown mechanism are common in metal–sulfur–water systems, and have been studied extensively by corrosion scientists [Citation24]. Similar corrosion reaction mechanism should be applicable to bismuth–sulfur–water system because water clearly promotes the reaction between bismuth and sulfur and the particle shapes of products are affected by the existence of water.
3.2 Bi2Se3
shows the XRD patterns of the products. In the 240 °C case, Bi2Se3 was produced as a single phase. The yield was 99%. Since the pH of the liquid after reaction was 6.5, which is nearly neutral, it is thought that there was almost no dissolution of the raw material in water. In the 210 °C case, a small unidentified peak is observed at 28.6°, but the Bi2Se3 was mostly produced as a nearly single phase. In the 180 °C case, Bi2Se3 was produced as the main component, but the peaks of the XRD pattern are wide; unidentified peaks for impurities were also seen at 23.4°, 28.8° and 32.7°. Next, in order to clarify the effects of the hydrothermal conditions, we conducted control experiments without using water. The results are shown in . In the 240 °C case, the major product was Bi2Se3; however, a small unidentified peak for the impurity is observed at 28.6°. At 210 °C, there is little change from the XRD pattern in the case with water. From this fact, it is thought that the hydrothermal conditions have less effect on the synthesis of Bi2Se3, compared to that of Bi2S3.
Fig. 3 The XRD patterns of the Bi2Se3 products produced at (a) 240 °C, (b) 210 °C, (c) 180 °C, (d) 240 °C without water, and (e) 210 °C without water. (f) The reported pattern of Bi2Se3 (ICSD no. 42545). Arrows show positions of unidentified peaks.
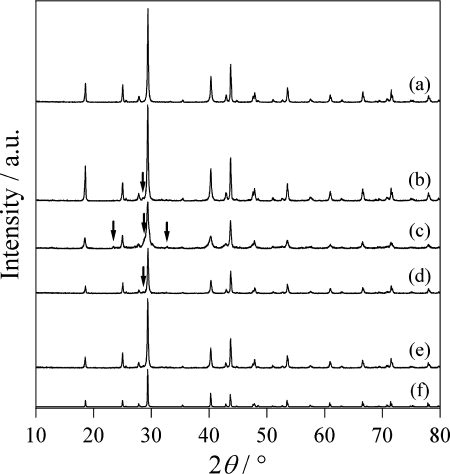
shows SEM images of the products. At 240 °C, the Bi2Se3 particles were produced in a plate-like form, with a width of ∼1 μm and a thickness of ∼0.2 μm. Even at 210 °C, since the Bi2Se3 was produced mostly as a single phase, particles with similar shapes were observed. However, at 180 °C, Bi2Se3 was produced and the plate-like particles were also observed, but many were observed to be thinner or smaller than those produced at 240 °C. The XRD patterns of the products in the control experiments without water did not change much with the hydrothermal conditions. However, for the particles of Bi2Se3 produced in cases without water, there were many grains observed to have grown isotropically instead of being plate-like. From these results, it can be said that, during the synthesis of Bi2Se3, water has less effect on the product, and more on the particle shape.
Fig. 4 SEM images of the Bi2Se3 products produced at (a) 240 °C, (b) 210 °C, (c) 180 °C, (d) 240 °C without water, and (e) 210 °C without water. SEM images of the starting materials (f) Bi and (g) Se.
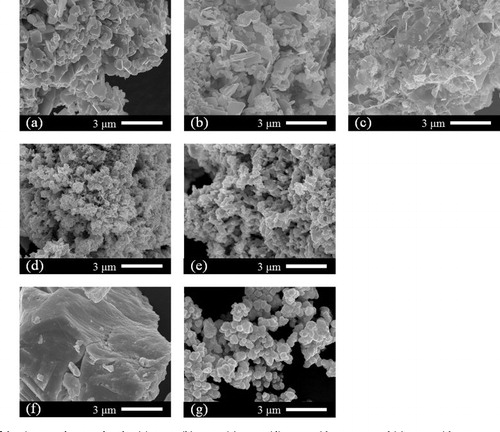
Generally, elemental sulfur is more reactive than elemental selenium because sulfur is easier to gain electrons, having higher electronegativity. Since standard Gibbs free energies of formation for Bi2S3, Bi2Se3, and Bi2Te3 are calculated to be −199, −140, and −78 kJ mol−1, respectively, from thermochemical data [Citation25], Bi2S3 is most stable. Thus, reactivity of selenium is expected to be worse than that of sulfur. However, in this study, almost pure Bi2Se3 phase was obtained in the water-free condition even at a low temperature of 210 °C. Furthermore, the difference in the products between conditions with and without water is less and the effect of water is reduced compared with the sulfur system. From these results, it is thought that the reactivity also depends on the particle size of the starting material. The particles of selenium (∼0.5 μm, (g)) are quite small compared with those of sulfur (>5 μm, (g)). Since the small particle promotes the reactivity and the diffusion, the role of water is less in the system.
3.3 Bi2Te3
shows the XRD patterns of the products. In the 240 °C case, the Bi2Te3 was mostly produced as a single phase; a small amount of Bi2TeO5 was produced as impurities. As the oxygen of the impurities was not included in the raw materials, it is thought that it originated from the dissolved oxygen in the water. The yield of the product was 99%. Since the pH of the liquid after the reaction was nearly neutral at 6.2, there was almost no dissolution of raw materials in water. In the 210 °C case, the peaks of the XRD patterns were slightly widened, but the proportions of the products were the same as in the case of 240 °C. Even at 180 °C, a substantially similar pattern was obtained, but a tiny peak for new unidentified impurities is seen at 30.3°. Next, we performed a control experiment without using water. The result is as shown in . In the 240 °C case, the major product was the Bi2Te3; the peaks for the unidentified impurities are observed at 26.2° and 29.9°. At 210 °C, almost no Bi2Te3 was produced; only the raw materials of Te and Bi were observed. From these results, the hydrothermal conditions were found to be quite advantageous for the synthesis of Bi2Te3.
Fig. 5 The XRD patterns of the Bi2Te3 products obtained at (a) 240 °C, (b) 210 °C, (c) 180 °C, (d) 240 °C without water, and (e) 210 °C without water. Reported patterns of (f) Bi2TeO5 (ICSD no. 36446), (g) Bi2Te3 (ICSD no. 158366), (h) Te (ICSD no. 96502), and (i) Bi (ICSD no. 53797). Arrows show positions of unidentified peaks.
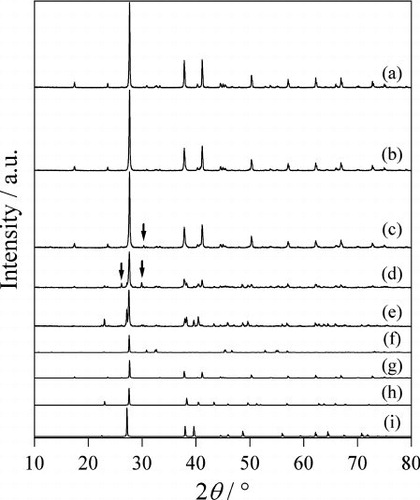
shows SEM images of the products. At 240 °C, the produced Bi2Te3 particles were rounded, with a diameter of ∼1 μm. At 180 °C, angulate particles around 1 μm wide and smaller particles approximately 0.5 μm wide were mixed. At 210 °C, there were many shapes of particles, as expected midway between 180 °C and 240 °C. For the Bi2Te3 particles produced in the case without water, no clear difference could be observed with those produced under hydrothermal conditions.
Fig. 6 SEM images of the Bi2Te3 products produced at (a) 240 °C, (b) 210 °C, (c) 180 °C, (d) 240 °C without water, and (e) 210 °C without water. SEM images of the starting materials (f) Bi and (g) Te.
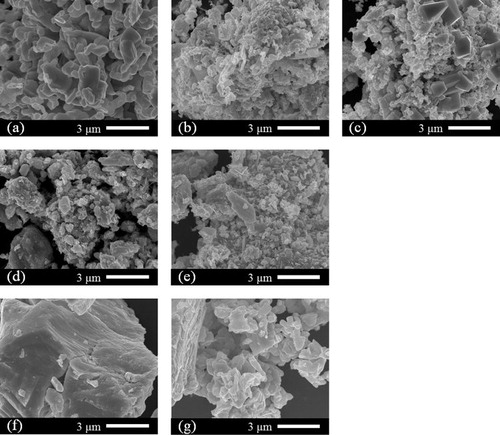
For the same reason as previously mentioned in the selenium system, the reactivity of tellurium is thought to be worse than that of sulfur. However, the reduction in the reactivity was not observed obviously. It is probably due to smaller particle of tellurium like the case of selenium, although the particles of tellurium are larger than those of selenium. On the other hand, the effect of water is remarkable and so the diffusion by water accelerates the reaction between bismuth and tellurium.
4 Conclusions
The synthesis of Bi2X3 (X = S, Se, Te) in the reaction of elemental bismuth and chalcogen (X) under hydrothermal conditions was successful. The yields of all Bi2X3 (X = S, Se, Te) products were also over 97%. The Bi2S3 was produced as a single phase at 240 °C. The obtained Bi2S3 particles were spherical, with a diameter of ∼1 μm. The Bi2Se3 was also produced as a single phase at 240 °C. The Bi2Se3 particles were plate-like, with a diameter of ∼1 μm and a thickness of ∼0.2 μm. Most of the Bi2Te3 was produced as a single phase and a small quantity of Bi2TeO5 as an impurity were observed in the 240 °C case. The Bi2Te3 particles were rounded, with a diameter of ∼1 μm.
The reactions in the bismuth–chalcogen–water system are understandable by the corrosion mechanism similar to the copper–sulfur–water system. In the sulfur and tellurium system, water drastically promotes the diffusion of particles and the reaction, while the effect of water is not observed obviously. The difference of the reactivities is thought to be due to the particle sizes of the starting materials, chalcogens.
Notes
Peer review under responsibility of The Ceramic Society of Japan and the Korean Ceramic Society.
References
- A.K.RathM.BernecheaL.MartinezG.KonstantatosAdv. Mater.23201137123717
- W.LiuK.LukasK.McEnaneyS.LeeQ.ZhangC.P.OpeilG.ChenZ.RenEnergy Environ. Sci.62013552560
- W.M.YimF.D.RosiSolid-State Electron.15197211211140
- J.P.FleurialL.GailliardR.TribouletH.ScherrerS.ScherrerJ. Phys. Chem. Solids49198812371247
- G.KonstantatosL.LevinaJ.TangE.H.SargentNano Lett.8200840024006
- B.ZhangX.YeW.HouY.ZhaoY.XieJ. Phys. Chem. B110200689788985
- Z.SunS.LiufuX.ChenL.ChenChem. Commun.46201031013103
- L.A.WrayS.XuY.XiaY.HorD.QianA.V.FedorovH.LinA.BansilR.J.CavaM.Z.HasanNat. Phys.62010855859
- H.ZhangY.JiX.MaJ.XuD.YangNanotechnology142003974977
- Y.YuC.H.JinR.H.WangQ.ChenL.-M.PengJ. Phys. Chem. B10920051877218776
- A.PhuruangratT.ThongtemS.ThongtemMater. Lett.63200914961498
- J.MaJ.YangL.JiaoT.WangJ.LianX.DuanW.ZhengDalton Trans.4020111010010108
- H.CuiH.LiuX.LiJ.WangF.HanX.ZhangR.I.BoughtonJ. Solid State Chem.177200440014006
- J.R.OtaP.RoyS.K.SrivastavaR.Popovitz-BiroR.TenneNanotechnology17200617001705
- H.T.ZhangX.G.LuoC.H.WangY.M.XiongS.Y.LiX.H.ChenJ. Cryst. Growth2652004558562
- M.Salavati-NiasariM.BazarganipourF.DavarJ. Alloys Compd.4892009530534
- K.TezukaW.C.SheetsR.KuriharaY.J.ShanH.ImotoT.J.MarksK.R.PoeppelmeierSolid State Sci.920079599
- K.TezukaR.KuriharaH.TakagiY.J.ShanH.ImotoTrans. MRS Jpn.322007131134
- K.TezukaH.TakagiR.KuriharaY.J.ShanH.ImotoJ. Ceram. Soc. Jpn.1152007929933
- K.TezukaH.TakagiY.J.ShanH.ImotoJ. Ceram. Soc. Jpn.11920115559
- Y.TokuharaK.TezukaY.J.ShanH.ImotoJ. Ceram. Soc. Jpn.1172009359362
- R.P.Rafal'skiiL.S.MedvedevaN.I.PrisyaginaV.A.AlekseevGeokhimiya51983665676
- R.BlachnikA.MullerThermochim. Acta36120003152
- P.MarcusCorrosion Mechanisms in Theory and Practice, second ed. (Corrosion Technology)2002Marcel DekkerNew York, NY287310
- M.BinnewiseE.MikeThermochemical Data of Elements and Compoundssecond ed.2002Woley-VCH Verlag GmbHWeinheim151855