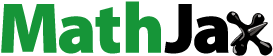
Abstract
A new easy-to-use process for obtaining fine powders of lanthanum-based perovskite-type oxides (La1−xAexMO3: Ae = Ca, Sr, M = Mn, Co, Fe) with a high specific surface area was successfully developed by the use of polyvinyl pyrrolidone (PVP) as a dispersant. This approach made it possible to prepare a single phase of perovskite by calcining at a lower temperature (600 °C) compared to conventional synthetic processes such as a solid state reaction and citrate process. SEM observations revealed that the oxide prepared with PVP was ca. 20–30 nm in size, while those prepared by the citrate process and solid state reaction were ca. 50 nm and 1 μm, respectively. Furthermore, the results of a thermal analysis in which we heated the precursors for the different reactions support the idea that PVP plays an important role in the formation of the perovskite phase at low temperature.
1 Introduction
Lanthanum-based perovskite-type oxides are well-known functional materials with a wide range of applications, and are used as electrocatalysts for alkaline fuel cells (AFC) [Citation1,Citation2] and metal air batteries [Citation3–Citation5], and as environmental catalysts [Citation6,Citation7] (e.g., oxidation of hydrocarbon and CO, and the reduction of NOx). Generally, an increase in the specific surface area of a perovskite-type oxide improves its catalytic activity by increasing the contact area between the catalyst and gas. However, particles of perovskite-type oxides are still not small enough, largely because the conventional synthesis processes require calcination at high temperature. Thus, the obtained perovskite particles are heavily agglomerated and sintered.
To date, several methods for the preparation of nano-sized perovskite-type oxides have been proposed to enhance the catalytic activity. Among these, the reverse micelle method [Citation8,Citation9] is one of the most effective approaches for the synthesis of nanocrystalline perovskite-type oxides with a high specific surface area (ca. 50 m2 g−1). However, this process is not particularly practical due to the low yield of the target material and the elaborate experimental conditions are required (surfactant, Rw value (molar ratio of [H2O] and [surfactant]), temperature, and calcination atmosphere). Thus, an easy-to-use approach for obtaining fine particles of perovskite-type oxides is required.
In addition, optimization of the constituent elements and their composition is also important for promoting catalytic activity because the perovskite-type structure is composed of a 12-coordinate cation (mainly, rare-earth or alkaline earth) site and a tetrahedrally coordinated transition metal cation site. Many studies have reported that the catalytic activity of La-based perovskite-type oxide (LaMO3) is drastically improved by doping the La3+ site with alkaline-earth metals. At a transition metal cation site, partial substitutions with different metals are often attempted to adjust the catalytic activity. Therefore, the desired perovskite-type oxides with high catalytic activity could be composed of several elements. In the preparation of a perovskite-type oxide with a high specific surface area and a suitable composition, the formation of impurities (in particular alkaline earth carbonate) can become a serious problem due to the low-temperature calcination. In fact, it is not easy to synthesize such materials by conventional low-temperature processes (e.g., citrate process, co-precipitation method).
It has been reported that the use of polyvinyl pyrrolidone (PVP) for the preparation of ceramic films is effective for controlling condensation and promoting structural relaxation of the materials under heat-treatment [Citation10]. This is also known to be advantageous for preparing materials with a high surface area and minimizing aggregation, and various oxides with a simple composition (i.e., ZnO [Citation11], NiO [Citation12], and CeO-based oxides [Citation13]) have already been reported. Since PVP has relatively high decomposition temperature, it should also be able to control particle aggregation.
In this study, a lanthanum-based perovskite-type oxide composed of 4 elements (La0.6Sr0.4Mn0.6Fe0.4O3) was prepared in the presence of PVP to increase the surface area of the perovskite-type oxide. For comparison, La0.6Sr0.4Mn0.6Fe0.4O3 was also synthesized by the conventional citrate process and solid state reaction. The characteristics of the resulting oxides were examined by BET (Brunauer–Emmett–Teller) specific surface area measurement, SEM observations and thermal analysis. Furthermore, perovskite-type oxides with various compositions (La1−xAexMO3: Ae = Ca, Sr, M = Mn, Co, Fe) were prepared using PVP to confirm the superiority of the present method.
2 Experimental
2.1 Sample preparation
The synthetic method of perovskite-type oxides using polyvinyl pyrrolidone (PVP) is as follows. A gel was obtained by stirring an aqueous solution of stoichiometric metal nitrates (La(NO3)3·6H2O (Wako, 99.9%), Sr(NO3)2 (Aldrich, 99.95%), Mn(NO3)2·6H2O (Wako, 99.9%), and Fe(NO3)3·9H2O (Wako, 99.9%)) and polyvinyl pyrrolidone (PVP; 3 times the total moles of cations) at 100 °C. By subsequently drying at 150 °C for 1 h, a precursor of perovskite-type oxide was obtained. The polymerized precursors were fired in air at 300–600 °C for 6 h in air, and perovskite-type oxides (La1−xAxMO3: A = Ca, Sr, M = Mn, Co, Fe) were obtained. In addition to the PVP method, La0.6Sr0.4Mn0.6Fe0.4O3 was also synthesized by conventional solid state reaction and citrate process for comparison. For the solid state reaction, La2O3 (Wako, 99. 5%), SrCO3 (Wako, 99. 9%), MnO2 (Aldrich, 99.99%), and Fe2O3 (Aldrich, 99.99%) were used as starting materials. The powders were thoroughly mixed in an agate mortar, and the mixture was sintered at 400–1300 °C for 10 h in air. For the citrate process, metal nitrates and citric acid monohydrate (C6H8O7·H2O (Wako); 2 times the total moles of cations) were dissolved in water, and the solution was stirred at 100 °C until a gel was formed. After drying the gel at 150 °C for 1 h, a precursor was obtained. The precursor was calcined in air at 400–800 °C for 10 h in air. For calcination, the temperature was increased at a constant rate of 20 °C min−1.
2.2 Characterization
The prepared perovskite-type oxides were identified by X-ray powder diffraction (XRD) analysis using Cu Kα radiation (RINT-Ultima+, Rigaku). The crystalline diameter was calculated from Scherrer's equation (Eq. Equation(1)(1)
(1) ):
(1)
(1) where D is the crystalline diameter (Å), K the Scherrer constant, λ the wavelength (m) and β is the FWHM.
The samples were also analyzed by scanning electron microscopy (SEM; JSM-6700F, JEOL), and wavelength dispersive X-ray fluorescence (XRF; Supermini, Rigaku). The specific surface areas of the oxides were calculated by the Brunauer–Emmett–Teller (BET) method (Bellsorp mini II, Bell Japan). Thermal analysis (TG-DTA) for the precursors of La0.6Sr0.4Mn0.6Fe0.4O3 was carried out in air (TG-DTA-200SA, Bruker) at a heating rate of 20 °C min−1.
3 Results and discussion
To determine the temperature at which a single phase of La0.6Sr0.4Mn0.6Fe0.4O3 forms completely, XRD patterns were measured for precursors of La0.6Sr0.4Mn0.6Fe0.4O3 after calcination at 300–1300 °C (). For the sample prepared using PVP, diffraction patterns of the perovskite structure can be observed over 350 °C, and the sample obtained by calcination of the precursor at 600 °C for 6 h was a single phase of the perovskite-type oxide. In contrast, the samples prepared by the citrate process and solid state reaction showed a perovskite structure and impurity phases such as SrCO3 and metal oxides after calcination at 600 °C for 10 h. For the citrate process and solid state reaction under the conditions in this study, a single-phase sample was confirmed after calcination at 800 °C for 10 h and at 1300 °C for 10 h, respectively. Thus, when PVP was used as a dispersing agent, a single phase of perovskite-type oxide could be synthesized at a lower temperature and a shorter heating time than in more conventional processes.
Fig. 1 XRD patterns of La0.6Sr0.4Mn0.6Fe0.4O3 obtained by heating precursors at 300–1300 °C ((a) PVP addition, (b) citrate process and (c) solid state reaction).
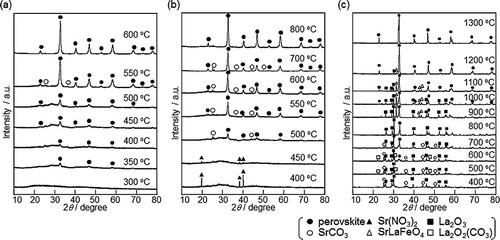
summarizes the composition, the BET specific surface area, and the crystalline diameter for the single phase of La0.6Sr0.4Mn0.6Fe0.4O3 prepared with the addition of PVP (600 °C), the citrate process (800 °C), and the solid state reaction (1300 °C). The composition of La0.6Sr0.4Mn0.6Fe0.4O3 samples determined by XRF was consistent with the theoretical values. The sample prepared using PVP was confirmed to have the highest BET surface area (31.1 m2 g−1) and the smallest crystalline diameter (23.5 nm) among the La0.6Sr0.4Mn0.6Fe0.4O3 samples prepared in this study.
Table 1 Sample composition, BET surface area, and crystalline diameter for La0.6Sr0.4Mn0.6Fe0.4O3 prepared by different processes.
SEM images of La0.6Sr0.4Mn0.6Fe0.4O3 particles are depicted in . The grain size for the sample prepared with the addition of PVP (a) was ca. 20–30 nm. In contrast, the samples synthesized by the citrate process (b) and solid state reaction (c) were definitely larger than that with the addition of PVP, with grain sizes of ca. 50–70 nm and ca. 1 μm, respectively.
Fig. 2 SEM images of La0.6Sr0.4Mn0.6Fe0.4O3 powders ((a) PVP addition (prepared at 600 °C), (b) citrate process (prepared at 800 °C) and (c) solid state reaction (prepared at 1300 °C)).
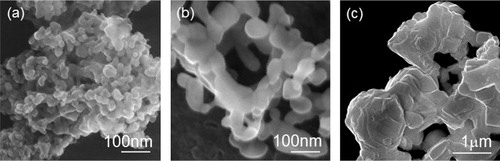
To confirm the superiority of the present PVP-addition method, perovskite-type oxides with different compositions (La1−xAexMO3: Ae = Ca, Sr, M = Mn, Co, Fe) were also prepared. The BET specific surface area and the crystalline diameter for the single-phase perovskite-type oxides prepared with the addition of PVP (calcination condition; 600 °C for 6 h) are shown in . All samples showed a single phase of perovskite-type oxide, as with La0.6Sr0.4Mn0.6Fe0.4O3. The samples synthesized using PVP have a relatively high BET surface area (ca. 30 m2 g−1) and a small crystalline diameter (20–30 nm).
Table 2 BET surface area and crystal diameter for perovskite-type oxides prepared by the PVP method.
Since heating at higher temperatures accelerates aggregation and sintering of the perovskite particles, the significant differences between these samples were considered to be due to the calcination temperature and heating duration. To investigate why a single phase of perovskite can be obtained at low temperature with the addition of PVP, thermogravimetric analysis (TG) and differential thermal analysis (DTA) were performed at a heating rate of 20 °C min−1.
shows the TG-DTA curves on heating La0.6Sr0.4Mn0.6Fe0.4O3 precursors in the PVP method, citrate process, and solid state reaction. For the precursor with PVP, large exothermic peaks with a dramatic weight loss were observed in the temperature range of 250–550 °C (peak temperature: 530 °C). The weight loss was completed at approximately 550 °C, and there was no further change in weight. While the precursor with the citric acid method also showed exothermic peaks with weight loss between 200 and 500 °C (peak temperature: 400 °C), the weight continued to gradually decrease at 500–700 °C. Since the precursor in the solid state reaction did not have such a large exothermic peak, the peaks in the PVP method and citrate process seem to correspond to decomposition and combustion of the polymerized precursor. These results suggest that the PVP method can be used to synthesize nano-sized perovskite-type oxide because the combustion reaction at higher temperature promotes the formation of oxides, and the rapid formation of perovskite after combustion inhibits the sintering of particles. In summary, the formation of a polymerized precursor with PVP plays a significant role in realizing this low-temperature synthesis due to the thermal property of PVP, and therefore, fine particles of perovskite-type oxide with complicated compositions could be easily obtained.
4 Conclusion
In this study, lanthanum-based perovskite-type oxides (La0.6Sr0.4Mn0.6Fe0.4O3) were synthesized by three methods, including the PVP-addition method, the citrate process, and the solid state reaction, and their particle sizes and specific surface areas were investigated. Among these synthetic approaches, the La0.6Sr0.4Mn0.6Fe0.4O3 obtained with the use of PVP showed the highest specific surface area and smallest particle size, most likely because calcination could be performed at a low temperature of 600 °C. In addition, since we confirmed that the present PVP method can be used for perovskite-type oxides with different compositions, it may be useful for obtaining electrocatalysts and environmental catalysts.
Notes
Peer review under responsibility of The Ceramic Society of Japan and the Korean Ceramic Society.
References
- D.B.MeadowcroftNature2261970847848
- Y.ShimizuK.UemuraH.MatsudaN.MiuraN.YamazoeJ. Electrochem. Soc.137199034303433
- M.BurcellM.PirjamaliY.KirosElectrochim. Acta47200216511660
- D.ThieleA.ZuttelJ. Power Sources1832008590594
- N.FujiwaraZ.SiromaS.YamazakiM.AsahiT.NagaiT.IoroiProceedings of the 80th meeting of Electrochemical Society of Japan20133A21
- T.IshiharaY.TsuruokaT.TodakaH.NishiguchiY.TakitaSolid State Ionics1522002709714
- T.IshiharaM.AndoK.SadaK.TakiishiK.YamadaH.NishiguchiY.TakitaJ. Catal.2202003104114
- M.HayashiH.UemuraK.ShimanoeN.MiuraN.YamazoeElectrochem. Solid-State Lett.161998268270
- M.A.HaiderA.J.CapizziM.MurayamaS.McIntoshSolid State Ionics19620116572
- H.KozukaM.KajimuraJ. Am. Ceram. Soc.83200010561062
- S.MaensiriP.LaokulV.PromarakJ. Cryst. Growth2892006102106
- D.TaoF.WeiMater. Lett.58200432263228
- T.MasuiH.ImazduN.MatsuyamaN.ImanakaJ. Hazard. Mater.176201011061109