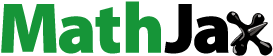
Abstract
Novel mesoporous silica sheets with surface amino-functional groups (sheet-NH2) were prepared for the efficient adsorption of aqueous metal ions. The sheet-NH2 were synthesized by a dual-templating process using Pluronic P123 and N-palmitoyl-l-alanine as templates. Two silicone regents were used to form the silica framework: tetraethoxysilane and 3-aminopropyltriethoxysilane. In the synthetic process, the as-prepared silicate was refluxed in ethanol to remove the organic templates remaining from the surface amino groups derived from APTES. The prepared sheet-NH2 showed widths of several micrometres and small thicknesses of approximately 50 nm. The pore diameter, pore volume and BET surface area of sheet-NH2 were determined by nitrogen adsorption–desorption isotherms to be 3.1 nm, 0.73 cm3 g−1 and 189.9 m2 g−1, respectively. TEM, XRD, FT-IR and TG–DTA analysis demonstrated that the sheet material had a disordered mesoporous structure and contained organic chains. The adsorptions of aqueous zinc(II) and copper(II) metal ions were examined and compared with amino-functionalized conventional mesoporous silica (MCM-NH2), calcined mesoporous silica sheets and silica beads with no porous structures. Notably, the sheet-NH2 exhibited the highest adsorption of both zinc and copper ions among the examined materials. In addition, the metal ion sorption equilibrium data of sheet-NH2 were fitted to the Langmuir isotherm model.
1 Introduction
Nowadays, the removal of heavy metal ions from aqueous solutions is one of the major problems for the treatment of industrial effluent because heavy metal ions in rivers and subterranean waters are largely toxic to animals, plants and human beings, even at low concentrations. The metal pollutants are disembogued from a variety of industries including mining, metal plating, textile refining and production, electric device manufacturing, painting and so on [Citation1 Citation2013;Citation3]. The major types of heavy metal ion contamination are copper, chrome, mercury, zinc, nickel and cadmium. The World Health Organization gives a value of 2.0 mg/L as the maximum copper concentration acceptable in drinking water [Citation4,Citation5]. Excess copper ion consumption can cause acute gastrointestinal effects and increase the environmental risks in humans. Heavy metals in waste waters are also significant resources for society. Therefore, it is very important to develop effective strategies for the removal of metal ions from water and industrial effluents, and researchers have paid considerable attention to this problem. Many techniques have been reported for the removal of metal ions; these include chemical precipitation, ion exchange, electrode deposition, various adsorbing materials and biological methods [Citation6 Citation2013;Citation8]. In this study, we focus on adsorption by inorganic materials, which is a widely used technique due to its simplicity and relatively low cost. Various materials including activated carbon, graphenes, zeolites, polymers, metal oxides, fly ash, cellulose, and silica materials are effective for the adsorption of metal ions [Citation9 Citation2013;Citation17].
Porous materials can adsorb metal ions, various molecules and nanoparticles on their surfaces. Kuroda et al. [Citation18] first reported the successful development of mesoporous silica (MPS) in 1990, and the sol–gel preparation method was reported by Mobil Co. researchers in 1992 [Citation19]. MPS is a silicon-based material in which the extremely high-surface area and large pore volume impart sufficient adsorption capacity. Moreover, MPS possesses beneficial characteristics including controllable pore size (2–50 nm), high-thermal stability, chemical stability, controllable composition and various porous structures such as two- and three-dimensional hexagonal, cubic and spherical structures [Citation20 Citation2013;Citation22]. MPS can be further surface functionalized by organic silane coupling agents. The optimization of the structure and surface characteristics of MPS facilitates its use as an adsorption material. For example, MCM-41, which is surface functionalized with glycidoxy groups, exhibited enhanced binding efficiency and stability as a protein carrier in antibody purification chromatography [Citation23]. Organo-functionalized MPS fabricated for biosensors enhances the catalytic activity and stability of immobilized enzymes [Citation24,Citation25]. These types of MPSs are often used as metal ion adsorption materials. Mureseanu et al. [Citation26] further demonstrated the use of amino- and salicylaldehyde-functionalized SBA-15 for heavy metal ion adsorption. Kang et al. [Citation27] reported the noble metal ion (Pt2+ and Pd2+)-selective adsorption using thiol-functionalized SBA-15. There is evidence that the organo-functionalization of MPS positively affects its adsorbent properties; however, the preparation process is complicated and expensive.
In our previous communication, enzymatic activity was improved by immobilization on an MPS sheet [Citation28]. As an adsorbent, MPS with sheet structure has the possibility to enhance the amount of adsorption of various metal ions. However, very few studies on the synthetic procedure for these materials have been reported [Citation29].
In the preparation process of the MPS sheet, we focused on the silica sources: tetraethoxysilane (TEOS) and 3-aminopropyltriethoxysilane (APTES). Calcination during the formation of the silicate framework removes not only organic templates but also the aminopropyl group from APTES. The silica product was then refluxed with excess ethanol to remove the organic templates. Wan et al. [Citation30] reported that the amount of extracted organic template depended on the reflux time in ethanol. High-adsorption capacities for various metal ions have been reported for amino-functionalized materials. Yoshitake et al. [Citation31] used mono-, di- and tri-amino-functionalized MCM-41 and SBA-1 to remove chromate and arsenate ions from water. Aguado et al. [Citation32] reported three types of aminopropyl-functionalized SBA-15 for Cu(II), Ni(II), Pb(II), Cd(II) and Zn(II) adsorption. Huang and Chen [Citation33] demonstrated the adsorption of Cu(II) and Cr(II) ions by an amino-functionalized magnetic Fe3O4 nano-adsorbent.
In this study, MPS sheets with aminopropyl groups (sheet-NH2) were prepared by the co-condensation method, and their ability to absorb copper and zinc ions was investigated. We focused on the morphology and surface characteristics of the adsorbents. For comparison with amino-functionalized MPS, conventional MCM-NH2 was prepared by similar synthetic conditions as those used to prepare the sheet-NH2. The metal ion adsorption ability between sheet-NH2, calcined mesoporous silica sheets (sheet-cal), commercially available silica beads and MCM-NH2 was compared, and the results were evaluated using the Langmuir and Freundlich isotherm models.
To the best of our knowledge, there are no reports on surface organo-functionalized MPS sheets. The purpose of this work is to determine the applicability of MPS sheets as a new technology for the removal of metal ions from water.
2 Experimental
2.1 Materials
Palmitoyl chloride, l-alanine, cetyltrimethylammonium chloride (CTAC), copper(II) acetate monohydrate, zinc acetate, acetone, sodium hydroxide and hydrochloric acid were purchased from Wako Pure Chemical Industries, Japan. Triblock copolymer Pluronic P123 (EO20PO70EO20; Mn ca. 5800 g mol−1) was obtained from Sigma–Aldrich, St. Louis, MO. TEOS and APTES were purchased from Shin-Etsu Chemical Co., Japan. NanoTek™ silicon oxide was obtained from C. I. KASEI Co., Japan. Metallo Assay LS (for copper, CU03M; for zinc, ZN01M) was supplied from Metallogenics Co., Japan. All other chemicals were analytical grade and used without further purification.
2.2 Preparation of mesoporous silica
According to our previous report, mesoporous amino silica sheets (sheet-NH2) were synthesized using a dual-templating approach [Citation28]. One of the templates, N-palmitoyl-l-alanine (C16-l-Ala), was prepared from palmitoyl chloride and l-alanine. Subsequently, 3.1 g of C16-l-Ala (9.5 × 10−4 mol) and 0.30 g of P123 (5.2 × 10−5 mol) were dissolved in 2.8 × 10−2 M sodium hydroxide aqueous solution (pH 12.5). After partial neutralization (pH 10) with 0.1 M HCl, 7.0 mmol of TEOS and 1.6 mmol of APTES were added to the mixture and stirred at 4 °C for 3 h. The reaction mixture was aged for 24 h at 25 °C and treated hydrothermally for 24 h at 100 °C. The resulting precipitate was refluxed in 200 mL of ethanol for 2 days to remove the templates. The molar ratio of the product was: C16-l-Ala/P123/TEOS/APTES = 18.3/1/135.6/24.4.
Mesoporous amino silica (MCM-NH2) was synthesized by the following process. CTAC (2.6 g) was dissolved in an aqueous HCl solution (pH 0.5) followed by the addition of 1.46 g of TEOS (7.0 mmol) and 0.28 g of APTES (1.6 mmol). After stirring for 5 h, 3.0 mL of ammonia (14.7 M) was added to the reaction mixture. After another 24 h of stirring, the mixture was centrifuged at 6000 rpm. The resulting precipitate was washed repeatedly with water and refluxed for 2 days with 200 mL of ethanol including 2 mL of conc. HCl to remove the template. The MCM-NH2 sample was collected by centrifugation and washed with water and ethanol.
2.3 Characterization
The morphologies of the prepared MPS materials were analysed using field emission scanning electron microscopy (FE-SEM; S4300, Hitachi, Japan) at an accelerating voltage of 10 kV and transmission electron microscopy (TEM; JEM 2010, JEOL, Japan) at an operating voltage of 200 kV. Small-angle X-ray diffractometry (XRD) patterns (RINT2100V/PC, Rigaku, Japan) were collected using Fe-Kα radiation operated at 40 kV and 30 mA. The Brunauer–Emmett–Teller (BET) specific surface areas and pore volumes of the samples were determined using nitrogen adsorption–desorption isotherms, and the pore size distribution curves were acquired by the Barret–Joyner–Halenda (BJH) method using a TriStar 3000 Micromeritics analyser (Shimadzu, Japan). Fourier transform-infrared spectra (FT-IR) were obtained using a MFT-2000 (Jasco Co., Japan). FT-IR peaks were analysed in the wavenumber range of 400–4000 cm−1 using a pellet made by 99% KBr and 1% sample. Thermogravimetry (TG) and differential thermal analysis (DTA) were performed on a Thermo Plus TG 8120 (Rigaku, Japan). The sample surface potential (zeta potential) in deionized H2O was measured by a zeta potential and particle size analyser (ELS-Z, Otsuka Electronics Co., Japan).
2.4 Adsorption of metal ions on mesoporous silica materials
Zinc acetate solution (1 mL; 10–150 μg/mL, pH 5.6) and copper(II) acetate solution (1 mL; 5–120 μg/mL, pH 5.4) were added to 2 mg of MPS materials (sheet-NH2 and MCM-NH2), respectively. After 3 days of stirring at room temperature, the metal ion adsorbate was separated by centrifugation at a speed of 12,000 rpm at 4 °C. To measure the amount of adsorbed metal ion on the silica materials, the UV intensity of the supernatant was analysed using the Metallo Assay LS. Zinc ion was determined by UV–vis absorbance (V-560, JASCO Co., Japan) at 560 nm, and copper ion was determined by a microplate reader (Wallac Arvo SX 1420 Multilabel Counter, Perkin Elmer, Inc., Waltham, MA) at 595 nm.
3 Results and discussion
3.1 Characterization of mesoporous silica materials
MPS sheets were prepared using dual organic templates (N-palmitoyl-l-alanine and Pluronic P123), and silica frames were formed using TEOS and APTES as silica sources (). The dual-templating surfactants had a role in determining the structural morphology of the sheet material, and the ratio of TEOS to APTES was a factor in determining the silica morphology [Citation28].
Fig. 1 Structures of organic templates and silica sources used in this study. (a) N-Palmitoyl-l-alanine (C16-l-alanine), (b) Pluronic P123 (polyethylene glycol-block-polypropylene glycol-block-polyethylene glycol), (c) tetraethoxysilane and (d) 3-aminopropyltriethoxysilane.
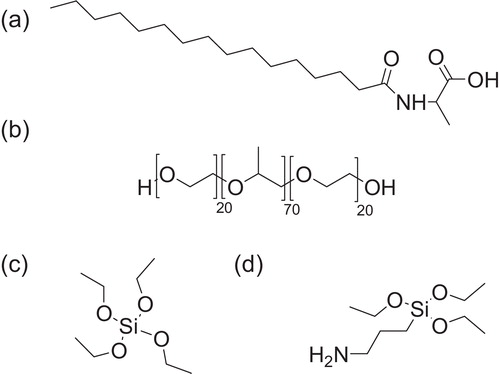
FE-SEM images of sheet-NH2 and MCM-NH2 are shown in . The thickness and width of sheet-NH2 are approximately 50 nm and 1–2 μm, respectively (). These results indicate that sheet silica materials with nano-sized thicknesses were prepared. The high-magnification SEM image of MCM-NH2 shows agglomerated structures with polyhedral particles mainly in the range of 200–500 nm (c and d).
shows the TEM images of sheet-NH2 and MCM-NH2. The sheet-NH2 sample indicated sheet structure (a and b). In c, MCM-NH2 sample exhibits mesopores.
Supplementary Fig. S1 can be found, in the online version, at doi:10.1016/j.jascer.2014.10.011.
Supplementary Fig. S1 FE-SEM image of sheet-NH2 by high magnification.
To prepare the MPS sheet, two organic surfactants (C16-l-Ala and P123) had a role in determining the structural morphology of the sheet material. Because of this combination of templates, sheet structure silica material can be formed. The thickness of the sheet depended on the molar ratio of P123. Phase separation is known to occur between the chiral surfactant (C16-l-Ala) and P123 triblock copolymer [Citation29,Citation34,Citation35]. The changes in micelle conformation and shape are induced by C16-l-Ala, leading to chiral micelles [Citation36]. We suggest that these two surfactants provide bi-layer walls that confine the sheet morphology to produce channels. The TEOS:APTES molar ratio was also an effective factor in determining the silica morphology. The positively charged site of APTES interacts electrostatically with the negatively charged head group of C16-l-Ala [Citation36]. APTES is co-condensed with TEOS, and the following reaction forms the silica framework.
The mesoporous structure of the sheet-NH2 and MCM-NH2 samples were investigated using small-angle XRD (). No sharp peaks were observed at 2θ = 0.5–12 in the XRD patterns of the sheet-NH2 and MCM-NH2 samples. This suggests that both materials have a disordered pore structure. Typically, MCM-41 exhibits the 2D-hexagonal (p6mm) structure; however, the synthetic process of MCM-NH2 using APTES as a silica source is influenced by the silica crystalline phase [Citation37].
The nitrogen adsorption–desorption isotherms of sheet-NH2 and MCM-NH2 are shown in a. Each curve is indicative of type IV sorption isotherms, and sheet-NH2 and MCM-NH2 exhibited type H4 and H1 hysteresis loops, respectively [Citation38,Citation39]. The pore distributions of sheet-NH2 and MCM-NH2 are illustrated in b. From b, sheet-NH2 sample indicated comparatively sharp peak. Thus, the sheet-NH2 suggests presence of mesopores. The textual properties derived from the BET and BJH methods are summarized in . The pore sizes of sheet-NH2 and MCM-NH2 were 3.1 and 2.7 nm, respectively. The pore volumes of sheet-NH2 and MCM-NH2 were calculated to be 0.73 and 0.39 cm3 g−1, respectively, and the BET surface areas were 189.9 and 364.6 cm2 g−1, respectively.
Table 1 N2 physisorption parameters and zeta potential of sheet-NH2 and MCM-NH2.
Fig. 5 Nitrogen adsorption–desorption isotherms (a) and pore distributions (b) of sheet-NH2 (black square) and MCM-NH2 (hollow diamond square).
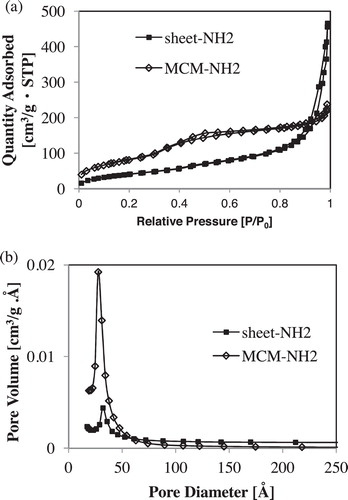
shows the FT-IR spectra of the samples. In the sheet-cal sample (curve a), the MPS sheet was calcined at 500 °C for 4 h to remove the template [Citation28]. The FT-IR spectrum of the sheet-cal exhibits a silanol group (SiOH) peak at 945 cm−1 and silica vibration (SiOSi) peaks at 1075 and 985 cm−1. The FT-IR spectrum of sheet-NH2 and MCM-NH2 (curves b and c) displays the silicate surface peaks along with CH2 bending peaks at 1460 cm−1. In addition, the FT-IR peaks at 2960 and 3300 cm−1 correspond to CH stretching and NH stretching, respectively. Thus, the spectral data sheet-NH2 and MCM-NH2 indicated the presence of amino groups derived from APTES. We demonstrated that the MPS sheets with NH2 surface groups were prepared by the simple process of refluxing with ethanol instead of calcination to remove the templates.
To analyse the amount of organic chain on the silica materials, TG–DTA curves were obtained (). The exothermic peaks of sheet-NH2 and MCM-NH2 occurred at 312 °C and 345 °C, respectively. These peaks are attributed to surface organic chains (with aminopropyl group) in the DTA curve (data not shown). The weight losses of the aminopropyl groups were estimated from the TG curves of sheet-NH2 and MCM-NH2 as 0.7 × 10−3 and 1.2 × 10−3 mmol/mg, respectively. These data indicate the presence of a similar amount of organic chain on sheet-NH2 and MCM-NH2.
The zeta potential of the samples are given in ; the zeta potential of sheet-NH2 was 9.15 mV, while that of MCM-NH2 was 16.59 mV. Generally, MPS is known to have a negative charge due to its surface silanol group. However, the sheet-NH2 and MCM-NH2 exhibited positive charges derived from their amino groups [Citation40,Citation41]. From these results, the surface functional mesoporous silica sheet is prepared without any modification.
3.2 Zinc and copper ion adsorption
shows the equilibrium isotherms for the adsorption of zinc and copper ions by sheet-NH2 and MCM-NH2. The sheet-NH2 sample exhibited a much higher maximum adsorption (qMax) of zinc ion (0.22 μmol/mg) compared to MCM-NH2 (under 1.0 × 10−2 μmol/mg; a). Sheet-NH2 also showed a significant qMax value for copper ion (0.18 μmol/mg; b). The calcined MPS sheet indicated a small amount of adsorption (<1.0 × 10−2 μmol/mg) of metal ions (data not shown). Similar to sheet-cal, the silica beads showed a trace amount of metal ion adsorption. Thus, the amino functional group on the material surface has a big influence on the adsorption of zinc and copper ions. Moreover, the mesoporous structure was significant for metal ion adsorption. Despite the presence of amino groups, the MCM-NH2 sample adsorbed a much smaller amount of metal ions than sheet-NH2. Expected mechanism of metal ion adsorption was illustrated in . Most of the metal ions will bind to the surface NH2 group on the carrier materials. Sheet-NH2 has better adsorption performance than MCM-NH2 because the metal ion binding site is highly exposed. In a simulation study, the amino groups were randomly oriented both inside and outside the silica matrix [Citation42]. It is possible that the adsorption site is more exposed in the porous nano-sheet structure compared to the cylindrical structure MPS (MCM type) due to its thinness. This result suggests that metal ions bind more easily to the surface of sheet-NH2. Thus, the amino-functionalized mesoporous nano-sheet structure was extremely effective for metal ion adsorption.
3.3 Isotherm modelling
The sorption equilibrium data describe how adsorbents interact with adsorbates and are important in the optimization of adsorbents. The Langmuir and Freundlich models are generally used to fit the experimental adsorption isotherm data. The Langmuir isotherm model is based on monolayer adsorption with equal energy and enthalpy for all adsorption sites. The equation is:where qe (mol/g) is the amount of metal ions adsorbed on the silica materials at adsorption equilibrium, C (mol/L) is the equilibrium metal ion concentration in the solution, kL (L/mol) is the Langmuir isotherm constant and qm (mol/g) is the amount of adsorbate adsorbed per unit mass of adsorbate corresponding to complete monolayer coverage. The linearized form can be given as follows:
The values of qm and k can be calculated from the slope and intercept of the linear plot of C/qe vs C.
The basic Freundlich equation is:where qe (mol/g) and C (mol/L) are defined as above, kF (L/g) is the Freundlich constant indicating adsorption capacity, and 1/n is a constant related to the sorption intensity. A linear form of the Freundlich equation can be expressed as follows:
The values of kF and 1/n were determined from the intercept and slope the linear plot of ln qe vs ln C.
3.4 Adsorption kinetics
In , the sorption data of sheet-NH2 were analysed in terms of the Langmuir and Freundlich isotherm models. The Langmuir constants qm and kL were 2.50 × 10−7 mol/g and 1.21 × 105 L/mol for zinc and 2.00 × 10−7 mol/g and 4.40 × 105 L/mol for copper, respectively. The Freundlich constants kF and 1/n were 7.71 × 10−7 L/g and 0.147 for zinc and 1.47 × 10−6 L/g and 0.211 for copper, respectively. The correlation coefficients of the Langmuir plot model were determined to be 0.9987 and 0.9834 for zinc and copper, respectively, and those for the Freundlich plot model were 0.9389 and 0.7504 for zinc and copper, respectively. The data demonstrates that the Langmuir isotherm model gives a much better fit than the Freundlich isotherm model. Thus, the adsorption of zinc and copper ions on the sheet-NH2 obeyed the Langmuir isotherm model. In other words, the surface of sheet-NH2 may be typically homogeneous, and the metal ions adsorb via the surface aminopropyl groups. The adsorption isotherms demonstrate that the MCM-NH2 sample was not fitted by the Langmuir or Freundlich models.
Table 2 Langmuir and Freundlich constants of sheet-NH2.
4 Conclusions
MPS sheet material sheet-NH2 was prepared by a dual-templating process using N-palmitoyl-l-alanine and Pluronic P123. These two surfactants had a role in determining the sheet structural morphology of the material. The sheet silica framework was formed by two silica sources: TEOS and APTES. The FE-SEM and TEM results indicated that the sheet silica material had a porous sheet structure with a thickness of approximately 50 nm thickness and a width of 1–2 μm. FT-IR spectra and TG–DTA curves confirmed the presence of surface amino groups on the MPS sheets. The pore size, pore volume and BET surface area of sheet-NH2 were 3.1 nm, 0.73 cm3 g−1 and 189.9 cm2 g−1, respectively.
For comparison with the sheet-NH2, conventional MCM-NH2 was prepared by a similar synthetic approach using TEOS and APTES. The sheet-NH2 exhibited the highest maximum adsorption for zinc ion (qMax = 0.22 μmol/mg) based on the equilibrium isotherms. Sheet-NH2 also possessed a remarkably high qMax value (0.18 μmol/mg) for copper ion adsorption. From the experimental adsorption isotherm data, zinc and copper ion adsorption on the monolayers of sheet-NH2 obeyed the Langmuir isotherm model.
We have demonstrated the improvement of amount of metal ion adsorption by functionalizing the surface of MPS sheets with aminopropyl groups (sheet-NH2). The mesoporous sheet structure significantly influences the adsorption capability by altering the exposure of adsorption sites, and the surface aminopropyl groups function as adsorption sites due to their positive charges. Thus, we propose sheet-NH2 as a material for the enhancement of metal ion adsorption. This material can be employed as a next-generation adsorbent to remove various metals from wastewater.
Supplementary Materials
Download MS Word (756 KB)Notes
Peer review under responsibility of The Ceramic Society of Japan and the Korean Ceramic Society.
References
- A.DemirbasJ. Hazard. Mater.1572008220229
- F.FuQ.WangJ. Environ. Manage.922011407418
- M.HuaS.ZhangB.PanW.ZhangL.LvQ.ZhangJ. Hazard. Mater.211–2122012317331
- World Health OrganizationGuidelines for Drinking-Water Quality2008WHOGeneva
- World Health OrganizationCopper in Drinking-Water2004WHO, SDE, WSH
- M.KarvelasA.KatsoyiannisC.SamaraChemosphere53200312011210
- S.HuangC.FanC.HouJ. Hazard. Mater.2782014815
- S.AhluwaliaD.GoyalBioresour. Technol.98200722432257
- M.RaoA.RameshG.RaoK.SeshaiahJ. Hazard. Mater. B1292006123129
- G.ZhaoJ.LiX.RenC.ChenX.WangEnviron. Sci. Technol.4520111045410462
- X.GuoB.DuQ.WeiJ.YangL.HuL.YanW.XuJ. Hazard. Mater.2782014211220
- S.KomarneniNucl. Chem. Waste Manage.51985247250
- C.LiuR.BaiL.HongJ. Colloid Interface Sci.303200699108
- S.BanerjeeM.JoshiR.JayaramSepar. Sci. Technol.39200416111629
- R.SalibaH.GauthierR.GauthierM.Petit-RamelJ. Appl. Polym. Sci.75200016241631
- A.KozawaJ. Inorg. Nucl. Chem.211961315324
- H.TranF.RoddickJ.O’DonnellWater Res.33199929923000
- T.YanagisawaT.ShimizuK.KurodaC.KatoBull. Chem. Soc. Jpn.631990988992
- J.BeckJ.VartuliW.RothM.LeonowiczC.KresgeK.SchmittC.ChuD.OlsonE.SheppardJ. Am. Chem. Soc.11419921083410843
- W.LiD.ZhaoChem. Commun.492013943946
- W.LiQ.YueY.DengD.ZhaoAdv. Mater.25201351295152
- J.KimY.SakamotoY.HwangY.KwonO.TerasakiS.ParkG.StuckyJ. Phys. Chem. B106200225522558
- K.NakanishiM.TomitaH.NakamuraK.KatoJ. Mater. Chem. B1201363216328
- Y.MasudaS.KugimiyaK.MuraiA.HayashiK.KatoColloids Surf. B10120132633
- K.MuraiK.KatoAppl. Surf. Sci.258201117251731
- M.MureseanuA.ReissI.StefanescuE.DavidV.ParvulescuG.RenardV.HuleaChemosphere73200814991504
- T.KangY.ParkJ.YiInd. Eng. Chem. Res.43200414781484
- K.NakanishiM.TomitaK.KatoRSC Adv.4201447324735
- Y.YehH.LinC.TangC.MouJ. Colloid Interface Sci.3622011345366
- M.WanW.LinL.GaoH.GuJ.ZhuJ. Colloid Interface Sci.3772012497503
- H.YoshitakeT.YokoiT.TatsumiChem. Mater.14200246034610
- J.AguadoJ.ArsuagaA.ArencibiaM.LindoV.GascónJ. Hazard. Mater.1632009213221
- S.HuangD.ChenJ. Hazard. Mater.1632009174179
- Y.YangM.SuzukiS.OwaH.ShiraiK.HanabusaJ. Mater. Chem.16200616441650
- Y.LiB.LiZ.YanZ.XiaoZ.HuangK.HuS.WangY.YangChem. Mater.252013307312
- S.CheZ.LiuT.OhsunaK.SakamotoO.TerasakiT.TatsumiNature4292004281284
- C.ChenH.LiM.DavisMicropor. Mater.219931726
- P.TanevL.VlaevJ. Colloid Interface Sci.1601993110116
- M.ThommesB.SmarslyM.GroenewoltP.RavikovitchA.NeimarkLangmuir222006756764
- K.BarquistS.LarsenMicropor. Mesopor. Mater.1162008365369
- J.RosenholmM.LindénJ. Control. Release1282008157164
- A.DoadrioJ.Sánchez-MonteroJ.DoadrioA.SalinasM.Vallet-RegíMicropor. Mesopor. Mater.19520144349