Abstract
We report a method to synthesize silica nanoparticles from bentonite clay. A series of thermal and acid treatment processes was performed on bentonite clay to lower the alumina and increase the silica content. The obtained silica rich clay was treated in two different concentrations (10 wt% and 40 wt%) with sodium hydroxide solution to form sodium silicate solutions (SSS). One type of SSS was hydrolyzed with three different concentrations (5 M, 10 M and 15 M) of nitric acid in the presence of ethanol as cosolvent while the other SSS was hydrolyzed with nitric acid in the presence of three different quantities (10 ml, 20 ml and 30 ml) of ethanol as cosolvent. A range of silica particle sizes from nanometer to micrometer was obtained by varying the contents of silica rich clay, HNO3, and ethanol. It was observed that the concentration of silica rich clay and HNO3 had a direct effect on the particle size. The increase in the quantity of ethanol from 10 ml to 20 ml produced bimodal particles of nanometer and micrometer size, which maintained at 30 ml. Inductively coupled plasma optical emission spectroscopy, atomic absorption spectroscopy, X-ray fluorescence, scanning electron microscopy and X-ray diffraction were utilized to characterize the clay, SSS and nanoparticles.
1 Introduction
Silica nanoparticles have found applications in a variety of fields including drug delivery systems, catalysis, biomedics, biological imaging, chromatography, sensors, liquid armors and as filler in composite materials [Citation1–Citation4]. A method for the production of spherical and mono-dispersed silica nanoparticles by the hydrolysis of tetraethyl orthosilicate (TEOS) in basic conditions was first reported in 1968 [Citation5]. Later, the effect of concentration of TEOS, water and alkali was also studied to find a correlation with the produced silica particle size [Citation6]. The shape and size of silica particles can also be tailored by controlling the reaction parameters such as time, temperature and solvent concentration [Citation7].
In addition to TEOS, sodium silicate solution (SSS) is another low cost precursor used for the synthesis of silica particles [Citation8,Citation9]. Rice husk, rice hull, bagasse ash and semi-burned rice straw ash are some of the waste materials used for the synthesis of SSS [Citation10–Citation13]. Silica particles are precipitated from SSS by using acids such as hydrochloric acid (HCl) [Citation14] as precipitating agent; carbon dioxide is also used [Citation15].
Bentonite clay is a potential source of silica, which contains smectite as a main clay mineral. As alumina component is present in clays along with silica, a range of acid and alkali hydrometallurgical methods are employed to isolate them from each other [Citation16–Citation19]. Usually acids are used for the processing of clay rather than alkalis [Citation20] and HCl is preferred over other acids due to the easy separation of filtrate from the residue [Citation21]. For effective removal of alumina from clays, calcination is a critical step; solubility of alumina increases after thermal treatment in the temperature range of 500–900 °C [Citation22,Citation23]. Previously, kaoline clay was studied for the production of aluminum sulfate and it was found that heating the clay to 700 °C for 1 h followed by acid leaching with sulfuric acid (H2SO4) were the optimum conditions to extract alumina [Citation18]. In another work, removal of alumina component from kaoline to produce pure alumina was studied and the recommended process involved the calcination of clay at 600 °C for 1 h followed by leaching with HCl. However, the synthesis of silica particles from bentonite clay has not been studied previously and demands investigation in this field.
In the present study, silica particles were synthesized from bentonite clay in nanometer and micrometer size range. Alumina content in bentonite clay was lowered by a series of thermal and acid treatments. SSS was derived from the clay and the solution was hydrolyzed with HNO3 in the presence of ethanol. The effect of concentrations of clay, acid and ethanol on the particle size and morphology of silica was studied.
2 Materials and methods
2.1 Materials
Bentonite clay of foundry grade was provided by a local foundry. Ethanol (C2H6O) was acquired from Lab-Scan and 37% hydrochloric acid (HCl) was purchased from Fisher Scientific. 65% nitric acid (HNO3) was provided by EMSURE®. Commercially available sodium hydroxide (NaOH) was purchased from local market while locally available distilled water was used during the entire process.
2.2 Methods
Bentonite clay was sieved through a mesh size of 100 μm. It was then heated at a temperature of 680 °C for 1 h in a muffle furnace. Later 100 g of clay was added in 1000 ml 2.5 M HCl solution and left for stirring at 90 °C for 2 h and filtered to separate the silica rich bentonite clay. It was then washed and filtered repeatedly until the pH was neutralized. Two different SSS were prepared by dissolving two different loadings (10 wt% and 40 wt%) of clay in 2 M NaOH solution at 90 °C for 2 h. The solution was filtered to separate residue and unwanted impurities. The obtained SSS was neutralized with HNO3 at 50 °C in the presence of ethanol as a cosolvent. The SSS prepared by dissolving 10 wt% clay was treated with three different concentrations of HNO3 (15 M, 10 M and 5 M) while keeping the amount of cosolvent constant. The SSS prepared by dissolving 40 wt% clay was treated with three different amounts of ethanol (10 ml, 20 ml and 30 ml) while keeping the acid concentration constant; the details are given in . The neutralized solutions were centrifuged and the obtained silica particles were washed repeatedly to remove the impurities including sodium. A graphical abstract of the process of synthesizing silica particles from bentonite clay is given in graphical abstract and the flow diagram of the process is represented in .
Table 1 Details of experiments including clay concentration and experimental conditions for the synthesis of silica particles.
2.3 Characterization
Compositional analysis of as-received bentonite clay and silica rich clay was performed by X-ray fluorescence (XRF) (WD XRD PANalytical). Elemental analysis of SSS obtained from silica rich clay was performed by inductively coupled plasma optical emission spectroscopy (ICP-OES) (IRIS ICP-OES, Thermo Jarrel Ash). Quantification of the sodium present in the SSS was performed by using atomic absorption spectroscopy (AAS). X-ray diffraction (XRD) of bentonite clay (before and after thermal treatment) and silica nanoparticles was performed for the phase analysis (Broker D8 Advance, CuKα, λ = 1.54184, Step size = 0.02045 and scan speed is 190 steps per minute). Before XRD analysis, the samples of silica particles were washed with 8 M HCl solution to remove the sodium and other impurities. Morphological examination and size measurement of silica particles was performed by using field emission gun scanning electron microscope (FEG-SEM) (Mira 3 TESCAN). For SEM, silica was dispersed in ethanol and then dropped on glass slide followed by carbon coating. For the average particle size and standard deviation, approximately 100 measurements were performed using SEM images of each sample.
3 Results and discussion
The composition of bentonite clay was determined by XRF and given in . The bentonite clay contains 58.1% SiO2 and 15.1% Al2O3 as major constituents. In a previous study, 36 samples of different types of bentonite clay were analyzed and up to a maximum of 66.8% SiO2 and 21% Al2O3 have been reported [Citation24]. To produce silica rich clay, the benonite clay was thermally treated at 680 °C for 1 h followed by acid leaching. An appreciable decrease in the amount of alumina was observed in silica rich clay, i.e. 6.83%, while SiO2 content increased to 65.05%; SiO2 content increased 11.9% while Al2O3 decreased 45.2% ().
Table 2 Composition of bentonite clay and silica rich clay analyzed by XRF.
shows the XRD patterns of pure bentonite clay and bentonite clay after thermal treatment but before acid leaching. The crystalline peaks of quartz can be clearly seen in both samples, which are showing no effect of temperature, i.e. 680 °C. Peaks of kaoline and montmrillonite can also be seen in the pattern of bentonite clay after thermal treatment. In bentonite clay after thermal treatment, an amorphous phase was observed in the range of 11°–14°. In pure bentonite, peak of kaoline exists in this region, which disappeared after the thermal treatment and substituted with an amorphous band, which indicates the transformation of kaoline to amorphous metakaolinite. Heating the bentonite clay causes its dehydration, which completes at 650 °C and further increase in the temperature leads to its dehydroxylation [Citation25]. The transformation of kaoline to metakaolinite is associated with dehydration of clay and it increases the clay reactivity for easily alumina extraction by acids or alkalis [Citation22,Citation26]. This argument is supported by XRF results as the amount of alumina was appreciably reduced by acid leaching the bentonite clay after heating ().
shows the elemental analysis of SSS after dissolving 10 wt% silica rich clay in 2 M NaOH. Silicon in the solution is 2069 μg/ml whereas sodium is 27,236 μg/ml. Aluminum is also present as a major impurity element in the solution. This analysis confirms the presence of silicon in the solution and formation of SSS. Other impurity elements present in SSS are zinc, titanium and boron.
Table 3 Composition of sodium silicate solution measured by ICP-OES.
shows the morphology and size of silica particles synthesized from SSS prepared by dissolving 10 wt% clay in NaOH and hydrolyzing the SSS with three different concentrations of HNO3 in the presence of ethanol as cosolvent. When 15 M HNO3 was used to hydrolyze SSS, spherical shaped silica nanoparticles with average size of 98 ± 20 nm were produced, as shown in (a and b). The size of silica nanoparticles reduced to 86 ± 11 nm when the SSS was hydrolyzed with 10 M HNO3. The size of the silica nanoparticles was further decreased to 69 ± 8 nm when 5 M HNO3 was used, as shown in (e and f). It can be inferred from the SEM images that particle size reduced by decreasing the acid concentration. Moreover, particle size distribution also becomes narrow by decreasing the acid concentration. Also, it can be clearly seen that the morphology of the particles is spherical. The reduction in acid concentration means the introduction of water in reaction system, which has resulted in smaller size of silica particles. In our previous investigation, SSS was diluted with water prior to the addition of acid and a decrease in silica particle size was observed and it was concluded that the addition of water leads toward production of more nuclei due to enhanced solubility of intermediate species, and the solubility of intermediates species is attributed to the high polarity of water [Citation27]. In the present study, water was added in acid instead of precursor and the amount of water was increased gradually in the form of decreasing molarity of acid. It is evident from the present results that amount of water in reaction system has direct relation with particle size as shown graphically in .
Fig. 3 SEM images of silica particles synthesized from SSS after dissolving 10 wt% clay. (a and b) 15 M HNO3, (c and d) 10 M HNO3 and (e and f) 5 M HNO3.
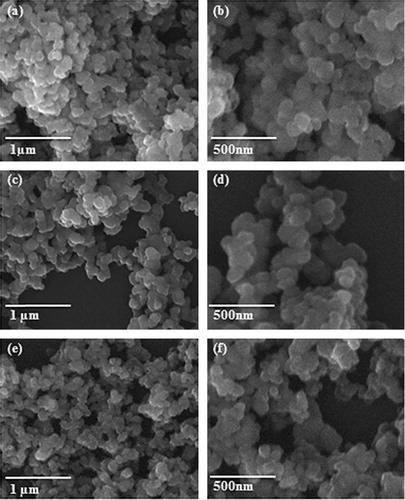
Fig. 4 Graph showing a decrease in silica particle size with decreasing acid concentration. Particles were produced from SSS after dissolving 10 wt% silica rich clay.
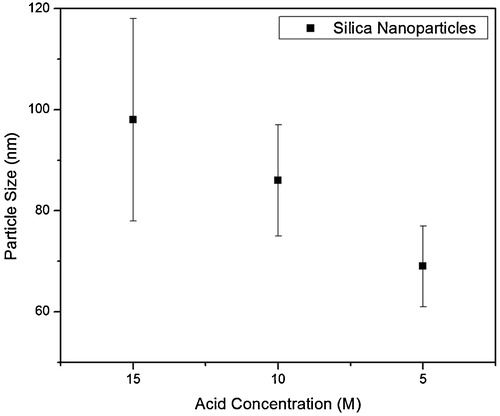
shows the SEM images of silica particles synthesized by mixing 40 wt% clay in NaOH solution and hydrolyzing the SSS with 15 M HNO3. Silica particles of 223 ± 68 nm were formed when 10 ml ethanol was used as cosolvent, as shown in (a–c). When the concentration of ethanol increased to 20 ml, bimodal particle size distribution was produced: one size of particles was in micrometer, i.e. 1.3 ± 0.2 μm and the other size was in nanometer range, i.e. 169 ± 53 nm ((d–f)). The presence of bimodal was also observed when the amount of ethanol was increased to 30 ml but with an increase in size of micrometer particles and decrease in size of nanometer particles, i.e. 1.4 ± 0.3 μm and 99 ± 23 nm ((g–i)). graphically shows the variation in particle sizes and modals with increasing amount of ethanol.
Fig. 5 SEM images of silica particles synthesized by using SSS after dissolving 40 wt% silica rich clay. (a–c) 10 ml ethanol, (d–f) 20 ml ethanol and (g–i) 30 ml ethanol.
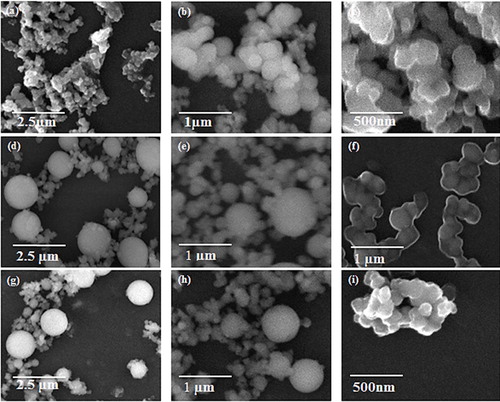
Fig. 6 Graph showing silica particles in nanometer and micrometer size range. Ethanol in concentration of 20 ml and 30 ml produced bimodal particle distribution.
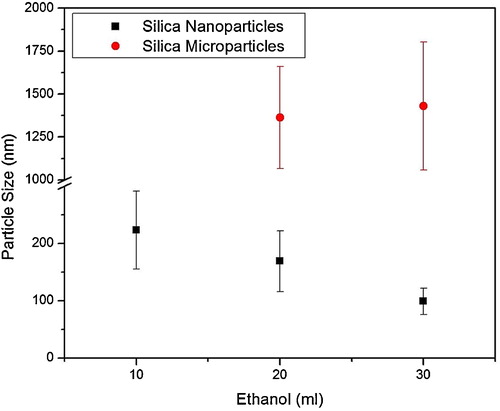
Bimodal distribution of silica particles was produced by increasing the quantity of ethanol from 10 ml to 20 ml and it was observed that the further rise in the quantity of ethanol (30 ml) did not disturb bimodal distribution. It has been reported that ethanol has a high miscibiliica precursor solution and it increases the formation of formation of ≡Si–OH and ≡Si–O–Si≡ resulting in the formation of large sized silica particles [Citation28,Citation29]. It was also observed that the amount of silica rich clay in NaOH to prepare SSS had a direct effect on silica particle size by keeping the quantity of ethanol (10 ml) and HNO3 (15 M) constant. For example, dissolving 10 wt% clay in SSS, the particle size was 98 ± 20 nm, which increased to 223 ± 68 nm by dissolving 40 wt% clay. The effect of concentration of silica source in precursor solution on particle size was observed in our previous study and it was observed that particle size and modal increases with increasing amount of white rice husk in NaOH [Citation27].
shows the XRD patterns of silica nanoparticles produced from SSS after dissolving 10 wt% silica rich clay. The pattern corresponds to characteristic amorphous nature of silica. Silica particles produced from SSS and rice hull show the amorphous nature and similar XRD pattern, as observed in the present investigation [Citation30,Citation31].
4 Conclusions
Silica particles were produced from bentonite clay in different sizes from nanometer to micrometer range. Silica rich clay was obtained from bentonite clay after a sequence of acid and thermal processes which was later treated with sodium hydroxide solution to prepare sodium silicate solution. Silica particles were produced by hydrolyzing sodium silicate solution with nitric acid in the presence of ethanol as a cosolvent. It was observed that the concentration of silica rich clay and nitric acid has a different effect on particle size, i.e. increased concentration produced particles of larger diameter. It was also found that bimodal distribution of silica particles was produced by increasing the amount of cosolvent. The silica particles were spherical in morphology and amorphous in nature, as confirmed by SEM and XRD, respectively.
Acknowledgement
The authors are thankful to Dr. Sajjad and Mr. Tahir Mehmood for their help in spectroscopic and microscopic analysis, respectively.
Notes
Peer review under responsibility of The Ceramic Society of Japan and the Korean Ceramic Society.
References
- P.NadrahO.PlaninšekM.GaberščekJ. Mater. Sci.492014481495
- K.W.GallisJ.T.AraujoK.J.DuffJ.G.MooreC.C.LandryAdv. Mater.11199914521455
- E.D.WetzelY.S.LeeR.G.EgresK.M.KirkwoodJ.E.KirkwoodN.J.WagnerAm. Inst. Phys. Conf. Ser.2004288293
- H.LyG.XlX.Z.H.ShG.XqJ.WqC.ZyAppl. Surf. Sci.252200687248733
- W.StöberA.FinkE.BohnJ. Colloid. Interface Sci.2619686269
- G.H.BogushM.A.TracyC.F.Zukoski IvJ. Non-Cryst. Solids104198895106
- H.S.MansurW.L.VasconcelosR.S.LenzaR.L.OréficeE.F.ReisZ.P.LobatoJ. Non-Cryst. Solids2732000109115
- E.R.EssienO.A.OlaniyiL.A.AdamsR.O.ShaibuJ. Met. Mater. Miner.212011712
- J.SchlomachM.KindJ. Colloid. Interface Sci.2772004316326
- T.WitoonM.ChareonpanichJ.LimtrakulMater. Lett.62200814761479
- T.LiT.WangMater. Chem. Phys.1122008398401
- R.R.ZakyM.M.HessienA.A.El-MidanyM.H.KhedrE.A.Abdel-AalK.A.El-BarawyPowder Technol.18520083135
- M.M.HessienM.M.RashadR.R.ZakyE.A.Abdel-AalK.A.El-BarawyMater. Sci. Eng. B16220091421
- A.A.E.-K.ZawrahM.F.ZawrahJ. Ovonic Res.52009129133
- M.ChoucairP.ThordarsonJ.A.StrideNat. Nano420093033
- J.A.EiseleD.J.BauerD.E.ShanksInd. Eng. Chem. Prod. Res. Dev.221983105110
- S.F.HulbertD.E.HuffClay Miner.81970337
- A.A.Al-ZahraniEng. Sci.152004
- A.A.Al-ZahraniM.H.Abdul-MajidJKAU: Eng. Sci.2020092941
- A.W.A.Al-AjeelS.I.Al-SindyIraqi Bull. Geol. Min.2.120066776
- D.J.O’ConnorAlumina Extraction from Non Bauxitic Materials1988Aluminium-Verlag
- C.V.PhillipsK.J.WillsHydrometallurgy919821528
- S.Y.GajamS.RaghavanMiner. Proc. Inst. Min. Met. C94C1985115120
- K.UferH.StanjekG.RothR.DohrmannR.KleebergS.KaufholdClays Clay Miner.562008272282
- S.żYmankowska-KumonM.HoltzerE.OlejnikA.BobrowskiMater. Sci.182012
- R.AjembaO.OnukwuliInt. J. Multidiscip. Sci. Eng.3201217
- U.ZulfiqarT.SubhaniS.Wilayat HusainJ. Non-Cryst. Solids42920156169
- C.Y.JungJ.S.KimT.S.ChangS.T.KimH.J.LimS.M.KooLangmuir26201054565461
- R.H.M.GodoiL.FernandesM.JafelicciJr.R.C.MarquesL.C.VarandaM.R.DavolosJ. Non-Cryst. Solids2471999141145
- S.MusićN.Filipović-VincekovićL.SekovanićBraz. J. Chem. Eng.2820118994
- G.NallathambiT.RamachandranV.RajendranR.PalaniveluMater. Res.142011552559