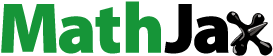
Abstract
Every day, several million tons of eggshells are being generated as bio-waste across the world. This study demonstrates the synthesis of HA powder using dicalcium phosphate dehydrate (CaHPO4·2H2O, DCPD) and eggshell powders via ball milling and subsequent heat treatment. The formation of HA phase can be initiated by sintering the 1 h milled sample at 1000 °C for 1 h, while pure HA phase can be obtained upon sintering the 10 h milled sample. Additionally, the final products composed of biphasic calcium phosphate (HA + β-TCP crystals) can easily be prepared by ball milling for 5 h followed by heat treatment at 1000 °C for 1 h. The carbonate peaks observed in the FTIR analysis of the as-prepared HA closely matched those of A- and B-type carbonates, which is typical of the biological apatite. The elemental composition of the as-synthesized HA showed the presence of Ca, P, Mg, and Sr.
1 Introduction
Hydroxyapatite (Ca10(PO4)6(OH)2, HA) is being actively investigated as a potential catalyst or a catalyst support for a variety of chemical reactions [Citation1,Citation2]. Besides, HA is also being analyzed as an adsorbent for environmental protection, owing to its strong affinity toward heavy metal ions [Citation3,Citation4]. Furthermore, synthetic HA finds application in various medical and dental applications as a promising material for healing damaged bones and teeth, for implant and scaffold, and as a drug delivery agent, due to its biocompatibility and bioactivity as well as its similarity to the inorganic component of the hard tissues in natural bones [Citation5,Citation6]. However, the properties of natural apatite crystals and conventional synthetic stoichiometric HA are significantly different [Citation7]. For example, the natural apatite in the human body contains significant amounts of carbonate and trace elements [Citation8,Citation9]. Natural bone is clearly a non-stoichiometric HA [Citation10]. The properties of synthetic HA are largely determined by its particle size, morphology, crystallinity, and composition, which in turn depend on the synthesis precursors and processing conditions [Citation11].
To this end, a variety of synthesis techniques, including sol–gel [Citation12], aqueous precipitation [Citation13], hydrothermal technique [Citation14–Citation16], and solid-state reactions [Citation17–Citation19] have been reported for the synthesis of HA. Over the past years, biologically derived natural materials, such as fish bone, bovine bone, corals, oyster shell, and eggshells, have been converted into useful biomaterials like HA. For instance, the study reported by Lemos et al. [Citation20] demonstrates the transformation of natural aragonite from cuttlefish bone into HA via hydrothermal treatment at 200 °C. Ooi et al. [Citation21] reported the synthesis of porous HA from bovine bone via heat treatment in the temperature range of 400–1200 °C. Sivakumar et al. [Citation22] have demonstrated the conversion of coral into monophasic HA via a low temperature hydrothermal process. A recent study has demonstrated the synthesis of HA from oyster shell powders mixed with calcium pyrophosphate (Ca2P2O7) or dicalcium phosphate dehydrate (CaHPO4·2H2O, DCPD) by ball milling and subsequently heat treatment [Citation18]. More recently, Ho et al. [Citation16] synthesized nanosized HA via hydrothermal treatment of eggshell and several biomolecules obtained from waste materials (pomelo, grape, and sweet potato peel extracts). These materials offer promising opportunities, given the fact that the raw materials are wastes. Besides, the use of ‘biological apatite’ containing some trace elements as bone substitutes, instead of ‘chemical apatite’, would be much beneficial for bone defect healing [Citation23].
Eggshells generated after breaking eggs represent a significant waste because they become typically useless after the use of egg contents and its derivatives. Such eggshell wastes are commonly disposed of in landfills without any pre-treatment. Such activities are highly undesirable to the environment, especially from the viewpoint of the odor generated during the biodegradation. Eggshells constitute 11% of the total weight of the egg and are mainly composed of calcium carbonate (CaCO3) [Citation24]. In the present investigation, an attempt has been made to synthesize pure and bone-like HA powder from eggshells. The eggshell powder, which was used as the Ca source, was combined with DCPD powder. In the typical process, the powder mixture was ball-milled for 1, 5, and 10 h, followed by heat treatment at 1000 °C for 1 h. Although there are several reports on the synthesis of HA from eggshells, most processes are done by wet chemical methods. Clearly, this technique is lengthy, complicated and require pH adjustment and control. To the best of our knowledge, no study has reported the synthesis of HA powder using DCPD and eggshell powders via ball milling and subsequent heat treatment. This study shows a great potential for the conversion of eggshell waste into highly valuable compounds using simple yet effective processes.
2 Materials and methods
In this study, DCPD (Yakuri Chemicals Co., Ltd., Japan) and eggshell powders were used as starting materials for the synthesis of HA. Raw membrane-bound hen eggshell was collected from a breakfast shop in the university campus and immediately stored in a refrigerator. The eggshells were pre-treated by stripping the membrane off the eggshell, followed by rinsing with water, drying, and then crushing and powdering using an agate mortar. The eggshell powders thus obtained were sieved using a 325-mesh sieve. Subsequently, the hand-ground eggshell powder and DCPD were homogeneously mixed with deionized water in a zirconia container. The ratio of eggshell powders to DCPD was 4:3 (mole ratio). The resulting mixture was wet-milled in a planetary ball-milling machine (QM-3SP4J, Nangjing, China) for 1, 5, or 10 h at a speed of 170 rpm in a zirconia bottle. After milling, the slurry was dried completely in a convection oven at 150 °C for 24 h. Following that, the dried powder was heated to 1000 °C at a rate of 10 °C/min, and subsequently held at that temperature for 1 h.
The crystalline phases of the ball-milled powders before and after heat treatment were analyzed by using powder X-ray diffraction with Cu Kα radiation (XRD; XRD-6000, Shimadzu, Japan). The phases were identified by comparing the experimental X-ray diffractograms with the standards compiled by the Joint Committee on Powder Diffraction Standards (JCPDS). The microstructure of the powders was observed using scanning electron microscope (SEM; JSM-6700F, JEOL, Japan) under secondary electron mode. Furthermore, the Fourier transform infrared (FTIR; Bio-Rad, FTS-40, USA) spectra of the powdered samples were obtained in the wavenumber range of 600–4000 cm−1. The composition of the synthesized powders was analyzed by using inductively coupled plasma-atomic emission spectroscopy (ICP-AES; ICAP 9000, Jarrell-Ash Co., USA).
From the XRD data, the crystallinity (Xc) of the HA particles was calculated according to the following equation [Citation25]:where I300 is the intensity of (300) diffraction peak and V112/300 is the intensity of the hollow between (112) and (300) diffraction peaks of HA.
The average crystallite size of the synthesized HA particles was calculated by using the Scherrer's formula as follows [Citation26]:where Xs is the average crystallite size (nm); λ is the wavelength of the X-ray used for the analysis (1.5406 Å); FWHM is the full width at half maximum for the diffraction peak under consideration (rad) and θ (°) is the Bragg's angle. In this study, the (002) diffraction peak was chosen for calculation of the crystallite size since it was isolated and sharper than the other peaks.
3 Results and discussion
3.1 Characterization of ball-milled powders
shows the XRD patterns of the DCPD and eggshell powder mixtures milled for 1, 5, and 10 h. As can be seen from the diffraction pattern of the 1 h milled powder, the peaks mainly correspond to dicalcium phosphate anhydrous (DCPA) and CaCO3 with trace amounts of β-TCP and HA phase. The DCPD precursor completely decomposed to DCPA because of the increased temperature and vigorous stirring during ball milling [Citation27]. Upon milling for 5 h, the intensities of DCPA and CaCO3 decreased with the obvious appearance of diffraction peaks corresponding to β-TCP phase, together with trace amounts of HA phase. With further increase in milling time to 10 h, the diffraction peaks indicated the formation of HA phase with small amounts of DCPA and CaCO3 from precursors. When compared to 5 h milled sample, the XRD pattern of the 10 h milled sample shows a decrease in β-TCP content, which indicates the gradual replacement of β-TCP by HA.
Fig. 1 XRD patterns of the powders produced by milling DCPD and eggshell powders for various durations (a) 1, (b) 5, and (c) 10 h.
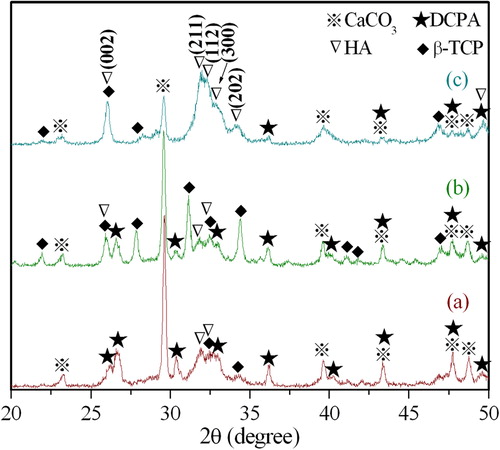
The microstructure of the DCPD and eggshell powders milled for 1, 5, and 10 h showed remarkable differences in size and shape (). The reduction in the particle size was greater with increase in milling time. The microstructure of the powders milled for 1 and 5 h showed larger particles of irregular shape and non-uniform size distribution, which could be related to the unreacted precursors. The submicron-sized particles observed in the 5 h milled sample could possibly be related to the β-TCP and HA phase. However, with increase in milling time to 10 h, the microstructure revealed smaller particles with relatively uniform size distribution.
3.2 Characterization of the powders after heat treatment
shows the X-ray diffraction patterns of the samples synthesized by milling DCPD and eggshell powders for 1, 5, and 10 h followed by subsequent heat-treated at 1000 °C for 1 h. The XRD pattern of the 1 h milled and sintered sample shown in a indicates the presence of unreacted Ca2P2O7 (pyrophosphate derived from DCPD after heating) and CaO (obtained from eggshell powders after heating). Besides, this stage had large amounts of β-TCP phase. However, with further increase in milling time to 5 h and subsequent heat-treatment, there were no peaks corresponding to CaO or Ca2P2O7. This indicates that the unreacted precursor has been incorporated into the HA and β-TCP lattice through high temperature diffusion. Furthermore, the intensity ratio, β-TCP(0210)/HA(211), also decreased with increase in ball-milling time, indicating that the HA phase is preferred over the β-TCP phase. With further increase in milling time to 10 h and subsequent heat treatment, the XRD peak corresponding to β-TCP phase disappeared completely and replaced by HA. The final products (10 h milled and heat-treated sample) were composed entirely of HA crystals. An earlier study [Citation28] has indicated the formation of HA powder via a one-step ball milling process without any heat treatment. However, in the current study, it seems that the milling time of 10 h is not sufficient to obtain a pure HA sample without sintering the powder at elevated temperatures. It is evident from that the formation of HA phase has been initiated through sintering the 1 h milled sample at 1000 °C for 1 h, while pure HA phase is formed only upon sintering the 10 h milled sample. The 1 and 5 h milled samples had additional XRD peaks corresponding to β-TCP phase.
Fig. 3 XRD patterns of the products synthesized by milling DCPD and eggshell powders for various durations (a) 1, (b) 5, and (c) 10 h, followed by heat treatment at 1000 °C for 1 h.
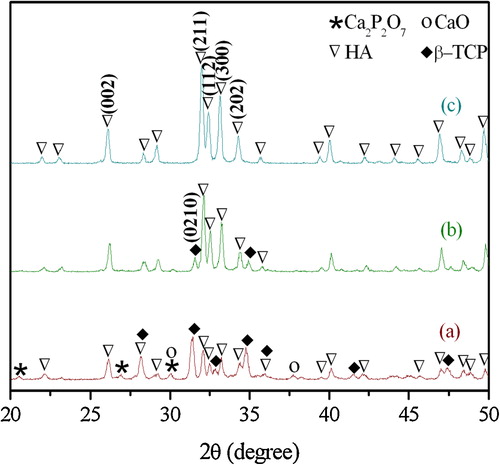
The final products composed entirely of HA crystals or biphasic calcium phosphate (HA + β-TCP crystals) can easily be prepared by varying the ball milling time, as shown in b and c. The formation of β-TCP phase under certain experimental conditions can be considered beneficial, although preparation of phase-pure HA from eggshell is the original intention of the present work. Recently, biphasic calcium phosphate (HA/TCP) composite has been studied to overcome the low biodegradability of HA. Tetsuya et al. [Citation29] demonstrated that HA ceramics containing TCP induced better osseointegration than pure HA ceramics when implanted in sheep femora. In addition, biphasic calcium phosphate ceramics have been evaluated in both osseous and non-osseous tissues [Citation30,Citation31]. Results have shown that the biphasic ceramics are biologically more active than pure HA ceramics, and that the biological behavior of the biphasic ceramics is superior in the formation of new bone.
shows the SEM images of the products synthesized from DCPD and eggshell powders milled for 1, 5, and 10 h and then heat-treated at 1000 °C for 1 h. As is seen, the powders have spherical shaped particles of nano to submicron size range. The average particle size decreased from 2.21 to 1.25 μm with increase in ball-milling time. Upon subsequent heat treatment, the agglomeration of the particles became more prominent due to high temperature sintering and high surface areas.
Fig. 4 SEM images of the products synthesized by milling DCPD and eggshell powders for various durations (a) 1, (b) 5, and (c) 10 h, followed by heat treatment at 1000 °C for 1 h.
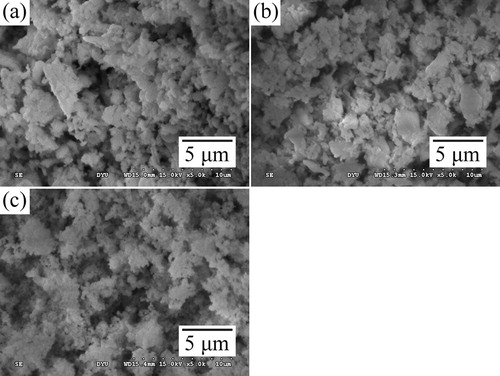
summarizes the crystallinity and crystallite size of the as-prepared HA powders milled for 1, 5, and 10 h, followed by heat-treatment at 1000 °C for 1 h. The crystallinity of the synthesized HA particles, as calculated from the XRD data, was found to increase with ball-milling time, although it was slightly lower than that of commercial HA. According to the XRD results (c), the as-synthesized HA powder exhibited good crystallinity (96.4%) and high phase-purity. The crystallite sizes of the HA particles, which were obtained by milling DCPD and eggshell powders for 1, 5, and 10 h followed by heat-treatment at 1000 °C for 1 h, were 37.1, 40.8, and 36.0 nm, respectively. According to previous reports, bone crystals can be 30–50 nm in length, 15–30 nm in width, and 2–10 nm in thickness [Citation32]. The nanostructure of bone-substituting materials is closely related to their good bioactivity and osteoconductivity. That said, synthetic HA composed of nanosized crystals could offer increased osteoblast functions [Citation33].
Table 1 Effect of ball milling on the crystallinity, crystallite size, and particle size of as-prepared HA powders obtained by milling for 1, 5, or 10 h, followed by heat treatment at 1000 °C for 1 h.
Furthermore, FTIR analysis was performed to identify the functional groups present in the HA particles. This in turn provided information about the constitution and phase composition of the products synthesized by milling DCPD and eggshell powders for 10 h followed by heat treatment at 1000 °C for 1 h. The FTIR spectra of the samples shown in had bands corresponding to OH−, PO43− and H2O. Further analysis of the IR spectrum revealed CO32− substitution, as identified by characteristic peaks of CO32− at around 888 and 1521 cm−1, which are attributed to the vibrational modes of the carbonate ions substituted at the hydroxide ion (A-type) [Citation34]. Moreover, the carbonate ion band at 1465 cm−1 can be ascribed to B-type carbonate substitution on phosphate ion sites [Citation35]. In this experiment, the carbonate peaks observed for the specimens closely matched those of A- and B-type carbonates. Indeed, anionic sites within the HA crystals are very susceptible to carbon dioxide originated from the atmosphere, and hence the CO32− generated from CO2 can substitute hydroxyls (A-type) or phosphates (B-type) in the crystal lattice [Citation36]. Accordingly, it can be concluded that the prepared HA is chemically and structurally analogous to apatite of natural bone. The apatite in natural bone contains significant amounts of carbonate ions, ranging from about 4 to 6 wt% [Citation32]. In recent years, several in vitro studies [Citation37,Citation38] have demonstrated the outstanding properties of carbonated HA for use as a bioresorbable bone substitute, as compared to pure stoichiometric HA. Also, in vivo study indicated that the dissolution rate of subcutaneously implanted sintered carbonated HA ceramics was intermediate between β-TCP and pure HA [Citation39]. The carbonated HA has been found to be more effective with a shorter post-operative rehabilitation program [Citation40]. Thus, among the variety of HA-based bioceramics, carbonated HA has attracted significant attention. Given this fact, the awareness of the contribution of carbonated HA to bone and dental enamel health has been greatly increasing [Citation41].
Fig. 5 FTIR spectrum of the HA prepared by milling DCPD and eggshell powders for 10 h, followed by heat treatment at 1000 °C for 1 h.
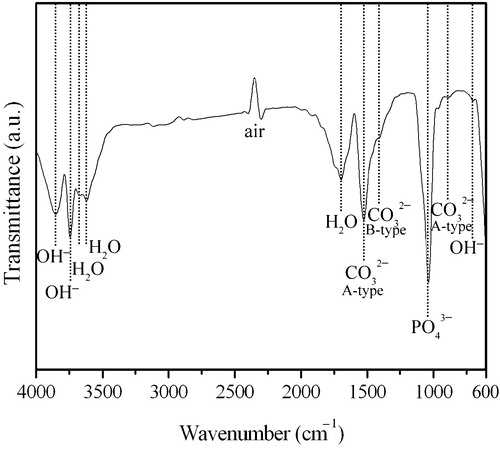
In the current study, pure HA is the main constituent of the product obtained from DCPD and eggshell powders ball-milled for 10 h followed by heat treatment at 1000 °C for 1 h (c). Elemental composition of the synthesized HA, as analyzed by using ICP-AES, showed the presence of Ca (39.2 wt%), P (16.8 wt%), Mg (0.648 wt%), and Sr (0.031 wt%), with an average Ca/P molar ratio of around 1.81. The higher Ca/P molar ratio observed in the present study, as compared to that of stoichiometric HA, can be attributed to the presence of carbonate ions substituting the phosphate. This type of HA is typical of the mineral phase of apatite in natural bone [Citation42], where the carbonates strongly contribute to the variation of Ca/P ratio. Other studies have observed similar high value of Ca/P ratio in HA from bovine bones [Citation43].
Eggshells are essentially composed of calcium carbonate (94%), calcium phosphate (1%), organic matter (4%), and magnesium carbonate (1%) [Citation24]. The natural biological origin of eggshells, containing several trace elements that remain in the crystalline structure of as-prepared HA making its composition alike human bone, will benefit the overall physiological functioning after implantation [Citation23]. Mg is known to be an important trace element in bone and teeth. Moreover, Mg plays an important role in bone metabolism and hence its depletion causes bone fragility and bone loss [Citation44]. Consequently, the incorporation of Mg ions into the HA structure is of great interest for the development of artificial bones. Besides, in conventional biomimetic coatings on Ti metals, the presence of Mg greatly influences both the physical and biological properties of the deposited coatings. Barrere et al. studied the role of Mg2+ ions in the deposition of apatite coating on Ti using concentrated SBF solution [Citation45]. It appeared that the relatively high interfacial concentration in Mg2+ favors heterogeneous nucleation of tiny Ca–P globules onto the Ti-6Al-4V substrate, thereby enhancing physical adhesion at the early stages of the coating formation. In addition, in vitro results showed higher cell adhesion of Mg2+ apatite coating than apatite coating, promoting proliferation and expression of collagen type I with respect to bare Ti [Citation46]. Similarly, Sr is reported to improve bone strength and provide benefits in the treatment of osteoporosis [Citation47]. Sr-containing HA has also exhibited better mechanical properties than those of stoichiometric HA, and has shown to enhance the proliferation and differentiation of osteoblast cells in vitro [Citation48]. Moreover, Xue et al. [Citation49] demonstrated that plasma-sprayed Sr-HA coatings on Ti-6Al-4V substrate exhibited good biocompatibility with human osteoblasts, favoring the survival and proliferation of cells on the surface of the coatings.
4 Conclusions
This work demonstrates the synthesis of HA from eggshell powders mixed with DCPD via ball milling and subsequent heat treatment. The following key results can be summarized based on the results obtained in this study:
(1) | The XRD pattern of the 1 h milled sample indicated a small amount of β-TCP and HA phases. With increase in milling time up to 10 h, the β-TCP phase decreased, resulting in predominantly HA phase with small amounts of DCPA and CaCO3 from precursors. |
(2) | The formation of HA phase can be initiated by sintering the 1 h milled sample at 1000 °C for 1 h, while pure HA phase can be obtained upon sintering the 10 h milled sample. Even after sintering, the presence of β-TCP phase was observed in the XRD patterns of the 1 and 5 h milled samples. No peaks corresponding to the starting materials were found in the powders milled for 5 and 10 h followed by heat treatment at 1000 °C for 1 h. |
(3) | The final products composed entirely of pure HA crystals or biphasic calcium phosphate (HA + β-TCP crystals) can easily be prepared by using DCPD and eggshell powders after 5 and 10 h of ball-milling, respectively, and subsequent sintering at 1000 °C for 1 h. |
(4) | The FTIR analysis of 10 h milled and sintered sample showed bands corresponding to OH−, PO43− and H2O. The carbonate peaks of the specimen closely matched those of A- and B-type carbonates. |
(5) | Based on the XRD results, it can be concluded that the as-synthesized HA powder obtained by milling DCPD and eggshell powders for 10 h followed by heat treatment at 1000 °C for 1 h exhibited good crystallinity (96.4%) and high phase-purity. The elemental composition of the synthesized HA, as evaluated by the ICP-AES method, showed the presence of Ca (39.2 wt%), P (16.8 wt%), Mg (0.648 wt%), and Sr (0.031 wt%). |
Notes
Peer review under responsibility of The Ceramic Society of Japan and the Korean Ceramic Society.
References
- K.MoriK.YamaguchiT.HaraT.MizugakiK.EbitaniK.KanedaJ. Am. Chem. Soc.12420021157211573
- J.H.JunT.J.LeeT.H.LimS.W.NamS.A.HongK.J.YoonJ. Catal.2212004178190
- G.LusvardiG.MalavasiL.MenabueM.SaladiniWaste Manag.222002853857
- A.KrestouA.XenidisD.PaniasMiner. Eng.172004373381
- B.ViswanathN.RavishankarBiomaterials29200848554863
- J.L.XuK.A.KhorJ.J.SuiJ.H.ZhangW.N.ChenBiomaterials30200953855391
- F.C.DriessensJ.W.VanDijkJ.M.BorggrevenCalcif. Tissue Res.261978127137
- E.LandiG.CelottiG.LogroscinoA.TampieriJ. Eur. Ceram. Soc.23200323912937
- M.Vallet-RegiJ.MarG.CalbetProg. Solid State Chem.322004131
- A.SiddharthanS.K.SeshadriT.S.Sampat KumarJ. Mater. Sci. Mater. Med.15200412791284
- S.DasguptaS.TarafderA.BandyopadhyayS.BoseMater. Sci. Eng. C33201328462854
- S.J.KalitaA.BhardwajH.A.BhattMater. Sci. Eng. C272007441449
- S.C.CoxP.JamshidiL.M.GroverK.K.MallickJ. Mater. Sci. Mater. Med.2520143746
- R.KumarK.H.PrakashK.YennieP.CheangK.A.KhorKey Eng. Mater.28420055962
- F.LiuF.WangT.ShimizuK.IgarashiL.ZhaoCeram. Int.322006527531
- S.C.WuH.K.TsouH.C.HsuS.K.HsuS.P.LiouW.F.HoCeram. Int.39201381838188
- S.H.RheeBiomaterials23200211471152
- S.C.WuH.C.HsuY.N.WuW.F.HoMater. Charact.62201111801187
- W.F.HoH.C.HsuS.K.HsuC.W.HungS.C.WuCeram. Int.39201364676473
- A.F.LemosJ.H.G.RochaS.S.F.QuaresmaS.KannanF.N.OktarS.AgathopoulosJ.M.F.FerreiraJ. Eur. Ceram. Soc.26200636393646
- C.Y.OoiM.HamdiS.RameshCeram. Int.33200711711177
- M.SivakumarT.S.S.KumarK.L.ShanthaK.P.RaoBiomaterials17199617091714
- M.BoutinguizaJ.PouR.ComesañaF.LusquiñosA.de CarlosB.LeónMater. Sci. Eng. C322012478486
- V.SinghN.MehtaInt. J. Sci. Eng. Investig.120129294
- E.LandiA.TampieriG.CelottiS.SprioJ. Eur. Ceram. Soc.20200023772387
- M.H.FathiaA.HanifiaV.MortazaviJ. Mater. Process. Technol.2022008536542
- A.C.TasJ. Am. Ceram. Soc.94201137223726
- S.LalaB.SatpatiT.KarS.K.PradhanMater. Sci. Eng. C33201328912898
- J.TetsuyaT.D.DwightV.M.GoldbergJ. Arthroplasty172002902909
- C.KleinK.de GrootW.ChenY.LiX.ZhangBiomaterials1519943134
- M.I.AlamI.AsahinaK.OhmamiudaS.EnomotoJ. Biomed. Mater. Res.542001129138
- M.J.OlsztaX.ChengS.S.JeeR.KumarY.Y.KimM.J.KaufmanE.P.DouglasL.B.CogerMater. Sci. Eng. R58200777116
- G.BalasundaramM.SatoT.J.WebsterBiomaterials27200627982805
- A.StochW.JastrzębskiA.BrożekJ.StochJ.SzaraniecB.TrybalskaG.KmitaJ. Mol. Struct.5552000375382
- R.MuruganS.RamakrishnaActa Biomater.22006201206
- S.KoutsopoulosJ. Biomed. Mater. Res.622002600612
- M.HasegawaY.DoiA.UchidaJ. Bone Jt. Surg.85B2003142147
- A.J.MelvilleJ.HarrisonK.A.GrossJ.S.ForsytheA.O.TrounsonR.MollardBiomaterials272006615622
- J.BarraletM.AkaoH.AokiJ. Biomed. Mater. Res.492000176182
- C.V.M.RodriguesP.SerricellaA.B.R.LinharesR.M.GuerdesR.BorojevicM.A.RossiM.E.L.DuarteM.FarinaBiomaterials24200349874997
- M.E.FleetX.LiuBiomaterials26200575487554
- A.AntonakosE.LiarokapisT.LeventouriBiomaterials28200730433054
- S.JoschekB.NiesR.KrotzA.GöpferichBiomaterials21200016451658
- A.HoppeN.S.GüldalA.R.BoccacciniBiomaterials32201127572774
- F.BarrereC.A.van BlitterswijkK.de GrootP.LayrolleBiomaterials23200222112220
- B.BracciP.TorricelliS.PanzavoltaE.BoaniniR.GiardinoA.BigiJ. Inorg. Biochem.103200916661674
- S.G.DahlP.AllainP.J.MarieY.MaurasG.BoivinP.AmmannY.TsouderosP.D.DelmasC.ChristiansenBone282001446453
- E.LandiA.TampieriG.CelottiS.SprioM.SandriG.LogroscinoActa Biomater.32007961969
- W.XueH.L.HosickA.BandyopadhyayS.BoseC.DingK.D.K.LukK.M.C.CheungW.W.LueSurf. Coat. Technol.201200746854693