Abstract
The relationship between protein adsorption on hydroxyapatite (HAp) particles and their surface structure was investigated. Because the various crystal planes of HAp have been reported to exhibit selective adsorption, numerous studies have focused on developing methods to control HAp morphology for selective adsorption. However, few studies have examined the systematic adsorption of proteins on the HAp particles. We firstly synthesized HAp particles under various aging times and mild reaction conditions intending to obtain HAp particles having various surface structures despite similar morphology, chemical composition, and crystallinity. The aging time affected the pore size distribution of the HAp particles. A peak indicating pores with a diameter of approximately 2.5 nm was observed in the pore size distribution plots of the HAp particles prepared using aging times of 48 h or less. The adsorption of proteins on HAp particles with different surface structures was studied. The bovine serum albumin (BSA) adsorption behavior was influenced by the presence of pores on the HAp surface. The amount of BSA adsorbed on the HAp particles aged 72 h having no pores was nearly 1.5 times that of the other HAp particles having pores. These results indicated that the pore size distribution of HAp particles is one of the most important factors in controlling their protein adsorption behavior.
1 Introduction
The relationship between protein molecules and inorganic materials has attracted extensive interest in many fields, including biomaterials [Citation1,Citation2], biomineralization [Citation3], biosensors [Citation4], and biochemistry [Citation5]. Hydroxyapatite (Ca10(PO4)6(OH)2, HAp) is the most well-known crystalline phase of calcium phosphate salts. Because HAp is both biocompatible and osteoconductive, it has been widely used in various applications such as orthopedic and dental materials and packing column materials for affinity chromatography for the separation of various proteins [Citation6 Citation2013;Citation8].
HAp has been reported to exhibit selective adsorption on different crystal planes. HAp crystallizes in the P63/m space group has unit-cell parameters of a = b = 0.943 nm and c = 0.688 nm. The HAp particles possess two different charge planes, C-sites and P-sites, on their surface. They also possess binding sites capable of adsorbing different proteins [Citation9,Citation10]. The calcium atoms in HAp include two screw-axis calcium ions (Ca2+) at the particle surface. The C-sites are arranged on the a-plane of particles. Therefore, the a-plane is rich in Ca2+ ions, whose positive charge facilitates adsorption of the acidic groups of proteins. The P-sites are composed of oxygen ions of PO43− group. The P-sites, which are negatively charged, are arranged hexagonally on the c-plane. Therefore, positively charged proteins tend to adsorb on the c-plane [Citation11,Citation12].
Improvements in the control of the crystal and morphological properties of synthesized HAp crystals are important for their utility as general adsorbents, affinity chromatographic solid supports, drug delivery systems, and protein carriers [Citation13 Citation2013;Citation15]. Therefore, numerous researchers have developed methods to control the HAp morphology to influence its protein adsorption behavior.
Yoshimura et al. have reported the preparation of HAp whiskers with a sharp-faced hexagonal morphology by hydrothermal treatment of HAp with nitric acid, urea, and cetyltrimethylammonium bromide (CTAB). They have also reported the syntheses of HAp particles with plate-, hexagonal-, prism-, and needle-like morphologies by hydrothermal synthesis [Citation16,Citation17]. Aizawa et al. have reported the syntheses of calcium-deficient apatite fibers by a homogeneous precipitation method. The fibers had long axes of 60–100 μm, and elongated along the c-axis of the crystal structure with a wide a-plane [Citation18,Citation19]. Nagata et al. have reported the synthesis of HAp plate-like particles with a wide a-plane by hydrothermal treatment with alcohol or ethylamine [Citation20,Citation21]. Sadat-Shojai et al. systematically correlated various parameters (e.g., pH, temperature, and time) related to the hydrothermal treatments with the shape of HAp nanoparticles using a Taguchi experimental design approach [Citation22]. Han et al. have reported a method for controlling the HAp nanoparticle morphology under hydrothermal treatment in the presence of urea and gelatin. They synthesized various HAp morphologies by altering the phase transition route by varying the initial pH and hydrothermal treatment time [Citation23]. Kawachi et al. have reported the selective BSA adsorption on needle-shaped hydroxyapatite under appropriate concentration of phosphate in the solution [Citation24].
Many researchers have demonstrated the ability to control HAp morphology, although only a few studies have examined the ability of HAp to selectively adsorb proteins. Numerous researchers have, however, reported studies detailing the relationship between protein adsorption behavior and HAp properties such as carbonate content, crystallinity, etc.
Kandori et al. have reported the effects of HAp chemical composition on the adsorption of bovine serum albumin (BSA) by synthesized carbonate calcium hydroxyapatites [Citation25]. Nagata et al. have reported that HAp with high crystallinity is strongly affected by its specific binding sites [Citation26]. Fuji et al. and Dasgupta et al. have reported on the highly selective protein adsorption properties of zinc-containing hydroxyapatite crystals [Citation27,Citation28].
As previously described, the protein adsorption behavior of HAp has been investigated using various types of HAp particles. However, it is also known that HAp particles synthesized with different methods show different protein adsorption behaviors, even if the HAp particles have similar morphologies, chemical compositions, and crystallinities. Therefore, we have focused on surface structure of HAp particles which is the interface structure that contacts proteins. Few studies have investigated the surface structure of HAp particle from the view point of protein adsorption on HAp particles. In this study, two different sized proteins, BSA (molecular weight 66,000) and lysozyme (LSZ, molecular weight 15,000), were used to examine their effects on the HAp surface structure. We firstly synthesized HAp samples with different surface structures despite their similar morphologies, chemical compositions, and crystallinities. Our aim is to evaluate the relationship between the HAp particle surface structure and the proteins adsorbed on the HAp. We prepared HAp under mild conditions to prevent changes in its morphology, chemical composition, and crystallinity. In this paper, we report the synthesis of HAp with a controlled surface structure and discuss in detail the relationship between the HAp particle surface structure and proteins that adsorb on the HAp.
2 Material and methods
2.1 Materials
Calcium acetate solution [(CH3COOH)2Ca·H2O] (99.0%), diammonium hydrogen phosphate [(NH4)2HPO4] (99.0%), and phosphate buffer (11 mM, pH 7.4) were procured from Wako Pure Chemical Industries, Japan. BSA [isoelectric point (pI) = 4.7, molecular weight 66,000] and LSZ (pI = 11.1, molecular weight 15,000) were procured from Sigma-Aldrich. All materials were of analytical grade and were used without further purification.
2.2 Preparation of HAp
HAp was prepared from a mixture of a 50 mM solution of (CH3COOH)2Ca·H2O and a 30 mM solution of (NH4)2HPO4. Two-hundred milliliters of the 30 mM (NH4)2HPO4 solution was added to 200 mL of the 50 mM (CH3COOH)2Ca·H2O solution. The resulting mixture was subsequently stirred for 10 min at 20 °C. The temperature of the mixture was increased at 1 °C/min using a cool stirrer from NISSIN (SWC-9000, series D). The mixture was further stirred at 60 °C for 3, 5, 24, 48, and 72 h. The time for which the mixture was maintained at 60 °C is hereafter referred to as the aging time. The products of various aging times were recovered by filtration, washed repeatedly with distilled water, and freeze–dried.
In order to compare the crystallinity of the products with highly crystalline HAp in the XRD analysis, highly crystalline HAp was prepared using a hydrothermal method at 180 °C for 3 h.
2.3 Characterization of synthesized HAp
The morphology of the samples was studied by field-emission scanning electron microscopy (FE-SEM) using a Hitachi S-4300 electron microscope (Japan) operated at 20 kV.
Fourier-transform infrared spectroscopy (FT-IR) was performed on a JASCO MTF-2000 (Japan). The synthesized products (approximately 2 mg) were thoroughly ground with potassium bromide powder (approximately 150 mg, IR-grade KBr, Wako) using an agate mortar and pestle to give a fine mixture that was subsequently subjected to IR analysis. Finely ground KBr powder was used for the background spectrum.
The products were characterized by powder X-ray diffraction (XRD) using a Rigaku RINT2000/PC equipped with a Cu–Kα radiation source operated at 30 mA and 40 kV. Data were collected in the 2θ range from 3.0 to 60.0° at a scan speed of 2.000°/min.
The values of the ζ-potential of the samples were measured using an ELS-Z (Otsuka Electronics Co., Japan).
All the samples were characterized by nitrogen gas adsorption/desorption isotherms using a Shimadzu TriStar3000 system (Japan). The pore volume of the samples was calculated using the BJH method.
2.4 Protein adsorption on HAp
The protein adsorption behavior was studied using BSA and LSZ. Five milligrams of HAp particles were mixed in 1 mL of a 0.5 mg/mL protein solution dissolved in a 11 mM phosphate buffer solution. The mixture was stirred overnight at 4 °C. The mixture was then centrifuged at 12,000 rpm for 12 min. The amount of unadsorbed protein in the supernatant was determined using the Bradforf protein assay. The amount of protein adsorbed on the HAp was calculated on the basis of the amount of proteins in the supernatant.
The equation used to calculate the protein adsorption per unit surface area iswhere q (g m−2) is the amount of protein adsorbed per unit surface area, Cp (mg mL−1) is the concentration of the initial protein solution, Cs (mg mL−1) is the concentration of protein in the supernatant, V (mL) is the volume of the protein solution, aHA (m2 g−1) is the specific surface area of the sample, and M (mg) is the mass of the sample used in the adsorption experiment.
3 Results and discussion
3.1 Properties of HAp particles
shows the XRD patterns of the products synthesized using different aging times. As a reference pattern of highly crystalline HAp, the XRD pattern of the product synthesized hydrothermally is shown in [(a)]. The peaks associated with HAp (JCPDS: 90432) were observed in the pattern for the product synthesized by the hydrothermal method. In the patterns of the products synthesized at 60 °C at aging times of 3, 5, 24, 48, and 72 h, all the peaks were assigned to the HAp. The patterns confirmed that the samples were single-phase HAp [(a)–(f)]. The HAp products synthesized hydrothermally were highly crystalline, as indicated by the sharpness of their XRD peaks [(a)]. In contrast, the peaks in the patterns of the products aged 3–72 h at 60 °C were less sharp than those in the hydrothermally prepared HAp pattern. The crystallinity of the HAp aged 3–72 h was lower than that of the hydrothermally prepared HAp. The sharpness of XRD peaks among the patterns of HAp aged 3–72 h was similar; thus, the HAp samples aged 3–72 h exhibited similar crystallinities.
Fig. 1 XRD patterns of hydroxyapatite samples synthesized (a) at 180 °C and at 60 °C for (b) 3 h, (c) 5 h, (d) 24 h, (e) 48 h, and (f) 72 h.
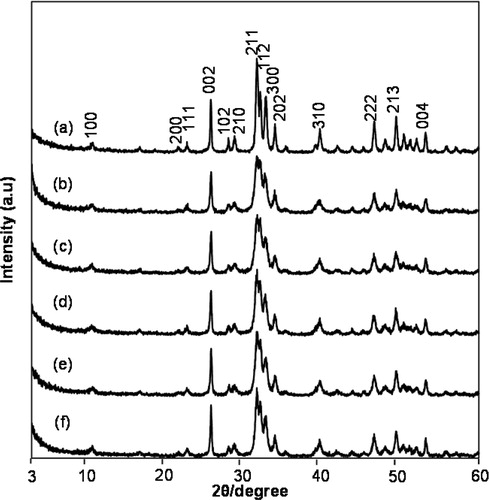
The morphologies of the products aged for various times were observed by FE-SEM [(a)–(e)]. These products primarily consisted of particles with a rod-like morphology. The products aged 3–72 h contained rod-like crystals with widths and lengths of 40 ± 10 nm and 180 ± 20 nm, respectively. The sizes of products aged 3–72 h did not vary remarkably.
Fig. 2 SEM images of hydroxyapatite synthesized using different aging times: (a) 3 h, (b) 5 h, (c) 24 h, (d) 48 h, and (e) 72 h.
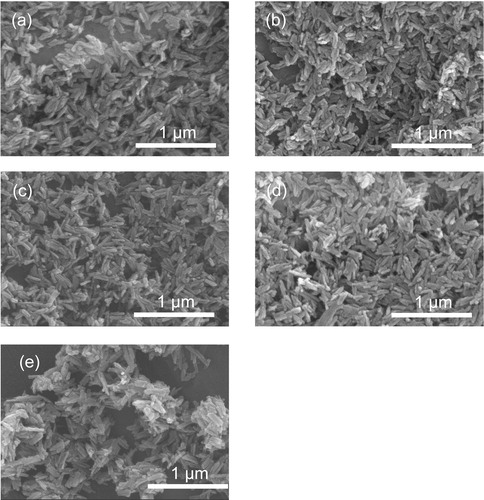
The functional groups contained in the HAp samples were analyzed by FT-IR. shows the FT-IR spectra of samples aged 3–72 h. The peaks at 3572 and 638 cm−1 are derived from the stretching and vibrational modes of the hydroxyl group. Peaks derived from the triply degenerated bending mode of the O–P–O bonds appear at 577 and 563 cm−1. Bands derived from the PO43− group appear at 1103, 1068, and 1028 cm−1. These peaks are a characteristic of HAp.
Fig. 3 FT-IR spectra of hydroxyapatite synthesized using different aging times: (a) 3 h, (b) 5 h, (c) 24 h, (d) 48 h, and (e) 72 h.
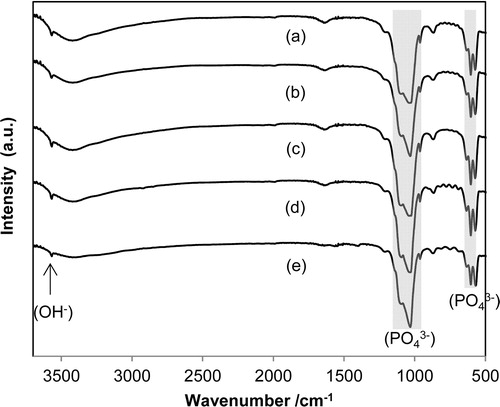
According to the literature, CO32− ions tend to occupy the OH and PO4 sites in the HAp structure [Citation25]. The FT-IR spectra contain bands that confirm the presence of CO32− ions. In the case where CO32− ions are substituted at the OH sites, the resulting carbonated HAp (CO3HAp) is designated as A-type substituted HAp. Bands derived from A-type HAp have been reported to appear at 1544 and 879 cm−1. In the case where CO32− ions occupy the PO4 positions, the resulting CO3HA is designated as B-type HAp [Citation29]. The bands derived from B-type appear at 1454, 1421, and 874 cm−1. These peaks derived from CO32− were not observed in our products; thus, the FT-IR spectra confirmed that the products aged 3–72 h were noncarbonated HAp.
The values of the ζ-potential for all HAp particles obtained in this study were −22 ± 4 mV. All HAp particles were negatively charged.
Surface analysis by N2 adsorption isotherms revealed that the aging time affected the pore size distribution and specific surface area of the HA particles. The specific surface area of products aged 3–48 h was calculated to be 90 ± 15 m2 g−1. In contrast, that of the product aged 72 h was 59 m2 g−1. The specific surface area of the product aged 72 h was substantially lower than that of the other products even though the SEM images indicated that the products aged 3–72 h exhibited similar morphologies. Therefore, the results of the specific surface area measurements suggest that the HAp particle surface structure could be altered by aging time.
The pore size distribution changed substantially with increasing aging time. A peak indicating a pore diameter of approximately 2.5 nm was observed in the pore size distribution plot for the HAp particles prepared at aging times of 48 h or less. In contrast, a corresponding peak was not observed in the pore size distribution plot for HAp particles aged 72 h []. According to these specific surface area results, products aged 3–48 h exhibited porous particle surfaces, which resulted in a large specific surface area. In contrast, the product aged 72 h did not have the ∼2.5-nm-diameter pores. Thus, the aging time altered the particles’ surface structure.
Fig. 5 Pore distribution on hydroxyapatite synthesized using different aging times: 3 h, 5 h, 24 h, 48 h, and 72 h.
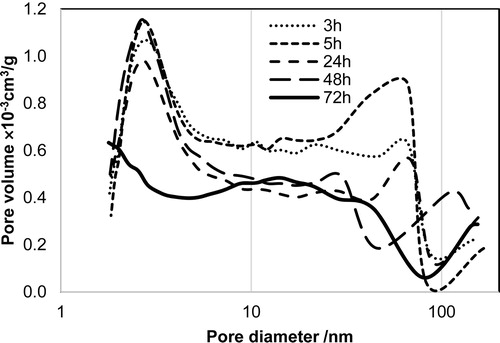
The pore size distribution results indicate that the HAp particles contain pores with a diameter of approximately 2.5 nm. The diameter of these pores is substantially smaller than the HAp particles whose morphologies were observed by FE-SEM. These results indicate that the pore size does not correspond to pore overlapping in HAp particles; therefore, pores should exist at the surface of the HAp particles. Wang et al. have reported that, during the earliest nucleation stage of the in vivo generation of tooth–enamel crystals, HAp crystals consist of 3–4 nm nanoparticles that are a composite of calcium phosphate nuclei and proteins [Citation30]. If the HAp particles synthesized in vitro also consist of 3–4 nm nanoparticles at the earliest nucleation stage, the pores with diameters of approximately 2.5 nm on the rod-like particles in this study might be caused by the space between the nanoparticles. The pore size distribution plot of the product aged 72 h did not exhibit a peak indicating the presence of ∼2.5-nm-diameter pores. The products’ surface structure might flatten during aging for 48–72 h by dissolution and reprecipitation of HAp particles.
3.2 Adsorption of proteins onto HAp
In this study, the synthesized HAp particles aged 3–72 h exhibited similar morphologies, chemical compositions, and crystallinities. The protein adsorption properties of the HAp particles were studied using two types of proteins: BSA (molecular weight 66,000) and LSZ (molecular weight 15,000). The results of protein adsorption on HAp are as shown in . [(b)] indicates that the amounts of LSZ adsorbed per unit surface area exhibited no obvious difference among the HAp particles prepared using various aging times. Unlike the HAp particles aged 48 h or less, the HAp particles aged 72 h did not contain ∼2.5-nm-diameter pores. However, the adsorption of LSZ on synthesized HAp did not affect the ∼2.5-nm-diameter pores. In contrast, the BSA adsorption behavior was influenced by the aging time of the HAp particles [(a)]. The amount of BSA adsorbed on the 72-h-aged HAp particles, which do not have the ∼2.5-nm-diameter pores, was nearly 1.5 times that adsorbed on the other HAp particles possessing pores. These results indicate that the ∼2.5-nm-diameter pores effectively increase the amount of BSA adsorbed on the HAp nanoparticles.
Fig. 4 Protein adsorption behavior of hydroxyapatite synthesized using various aging times: (a) bovine serum albumin (BSA) and (b) lysozyme (LSZ).
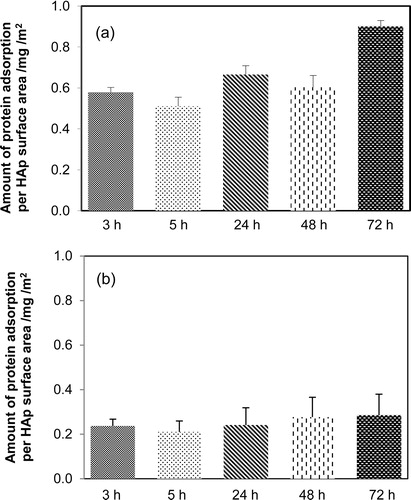
All the products exhibited similar morphologies, chemical compositions, and crystallinities, as indicated by SEM, FT-IR, and XRD, respectively. However, according to the results of protein adsorption studies, the amount of BSA adsorbed on HAp aged 72 h was significantly greater than the amounts adsorbed on the other samples. The primary difference among the HAp samples aged 3–72 h was the presence or absence of the ∼2.5-nm-diameter pores in their surface structures. Hence, the disappearance of the pores resulted in an increase in the amount of BSA adsorbed on the product aged 72 h. The ∼2.5-nm pores affected the amount of BSA adsorbed on the HAp. Particular attention was paid to the effect of the pore size on the amount of proteins adsorbed for different protein sizes, because the primary difference between BSA and LSZ lied in their respective sizes (BSA: 4 × 15 nm2; LSZ: 3 × 3.5 nm2). Thus, we plotted the amounts of BSA and LSZ adsorbed against the volume of the pores whose diameters were presumed to be smaller than BSA. shows the relationship between the amounts of proteins adsorbed on HAp particles and the volume of pores with diameters <10 nm. No variation was observed in the amount of LSZ adsorbed as a function of the pore volume. In contrast, the amount of BSA adsorbed exhibited a negative correlation to the pore volume. These results allowed us to assume that BSA would be difficult to adsorb inside the pores of the HAp particles, because BSA (4 × 15 nm2) is obviously larger than the pores. However, LSZ (3 × 3.5 nm2) is sufficiently small to adsorb inside the pores of the HAp particles. In this study, we revealed that surface structures of HAp particles were significantly changed by synthesize condition, even if the HAp particles have similar morphologies, chemical compositions, and crystallinities. Furthermore, the surface structures, ∼2.5-nm-diameter pores, affected protein adsorption behavior on HAp particles. These results indicate that the pore size distribution of HAp particles is one of the most important factors in controlling their protein adsorption behavior.
4 Conclusion
The effects of HAp particle surface structure on its protein adsorption behavior were examined. We synthesized HAp particles using various aging times intending to obtain HAp particles with different surface structure. HAp samples aged for 3 h or longer exhibited similar morphologies, chemical compositions, and crystallinities. The particle surface structure of HAp was altered by simply varying the synthesis aging time. The BET results indicate that HAp particles aged for 48 h or less have the pores with a diameter of approximately 2.5 nm, however the pores disappeared in the HAp aged 72 h. Protein adsorption studies on HAp revealed that the amounts of BSA adsorbed per unit surface area decreased with increasing pore volume of HAp, the pore diameters of which were <10 nm. In contrast, no change was observed in the amount of LSZ adsorbed as a function of the pore volume. This unique adsorption behavior is explained by the relationship between the size of the proteins and the pore diameter. Specifically, BSA (4 × 15 nm2) is substantially larger than the pores, whose diameter is approximately 2.5 nm; thus, adsorption of BSA inside the pores of the HAp particles is difficult. Therefore, the amount of BSA adsorbed is negatively correlated to the pore volume. However, the size of LSZ (3 × 3.5 nm2) is sufficiently small to adsorb inside the pores of the HAp particles. These results suggest that the pore size distribution of HAp particles affects their protein adsorption behavior. In this study, we revealed that it is of great importance to focus on surface structure of HAp particles systematic understanding of protein adsorption behavior.
Acknowledgment
This research was partially supported by Adaptable and Seamless Technology Transfer Program through Target-driven R&D, Japan Science and Technology Agency (AS262Z01181M).
References
- P.MalmbergH.NygrenProteomics8200837553762
- M.Sadat-ShojaiM.AtaiA.NodehiL.NasiriKhanlarDent. Mater.262010471482
- S.H.RheeJ.TanakaJ. Am. Ceram. Soc.81199830293031
- B.WangaJ.J.ZhangaZ.Y.PanaX.Q.TaobH.S.WangBiosens. Bioelectron.24200911411145
- T.OkudaK.IokuI.YonezawaH.MinagiY.GondaG.KawachiM.KamitakaharaY.ShibataH.MurayamaH.KurosawaT.IkedaBiomaterials29200827192728
- S.V.DorozhkinJ. Mater. Sci.44200923432387
- S.V.DorozhkinMaterials22009399498
- C.OhtsukiT.IchikawaH.ShibataG.KawachiT.TorimotoS.OgataJ. Mater. Sci. Mater. Med.21201012251232
- T.KawasakiK.IkedaS.TakahashiY.KubokiEur. J. Chem.1551986249257
- T.KawasakiS.TakahashiK.IkedaEur. J. Chem.1521985361371
- D.T.H.WassellR.C.HallG.EmberyBiomaterials161995697702
- M.R.MucaloK.KatoY.YokogawaColloids Surf. B: Biointerfaces7120095258
- T.MatsumotoM.OkazakiM.InoueS.TamaguchiT.KusunoseT.ToyonagaY.HamadaJ.TakahashiBiomaterials25200438073812
- K.KandoriS.MizumotoS.ToshimaM.FukusumiY.MorisadaJ. Phys. Chem. B11320091101611022
- F.NagataT.MiyajimaY.YokogawaJ. Eur. Ceram. Soc.262006533535
- I.S.NeiraF.GuitianT.TaniguchiT.WatanabeM.YoshimuraJ. Mater. Sci.43200821712178
- I.S.NeiraY.V.Kolen’koO.I.LebedevG.V.TendelooH.S.GuptaF.GuitiánM.YoshimuraCryst. Growth Des.912009466474
- M.AizawaH.UenoK.ItataniI.OkadaJ. Eur. Ceram. Soc.262006501507
- M.AizawaM.MatumotoJ. Soc. Inorg. Mater. Jpn.132006345352
- F.NagataY.YokogawaM.ToriyamaY.KawamotoT.SuzukiK.NishizawaJ. Ceram. Soc. Jpn.103119957073
- F.NagataM.ToriyamaK.TeraokaY.YokogawaChem. Lett.82001780781
- M.Sadat-ShojaiM.-T.KhorasaniA.JamshidiCryst. Growth Des.36120127384
- G.S.HanS.LeeD.W.KimD.H.KimJ.H.NohJ.H.ParkS.RoyT.K.AhnH.S.JungCryst. Growth Des.13201334143418
- G.KawachiT.WatanabeS.OgawaM.KamitakaharaC.OhtsukiJ. Ceram. Soc. Jpn.11772009847850
- K.KandoriM.SaitoT.TakebeA.YasukawaT.IshikawaColloids Surf. B: Biointerfaces519958187
- F.NagataY.YamauchiM.TomitaK.KatoJ. Ceram. Soc. Jpn.12192013797801
- E.FujiiM.OhkuboK.TsuruS.HayakawaA.OsakaK.KawabataC.BonhommeF.BabonneauActa Biomater.220066974
- S.DasguptaS.S.BanerjeeA.BandyopadhyayS.BoseLangmuir26201049584964
- S.KoutsopoulosJ. Biomed. Mater. Res.622002600612
- L.WangX.GuanH.YinJ.Moradian-OldakG.H.NancollasJ. Phys. Chem. C112200858925899