Abstract
LaO0.5F0.5BiS2 nanosheets were produced by ultrasonification. A slightly reddish colloidal suspension was obtained by ultrasonification of LaO0.5F0.5BiS2 powder in ethanol. Transmission electron microscope (TEM) images of the dried suspension indicated the nanosheet morphology of LaO0.5F0.5BiS2, and the electron diffraction spots indicated 4-fold symmetry. The thickness of the sheets was 2–6 nm, which corresponded to 2–4 unit cells of the c-axis of LaO0.5F0.5BiS2.
1 Introduction
Chalcogenite nanosheets, including MoS2, WS2, and ternary/quaternary compounds, have been extensively studied for use in catalysts, capacitors, sensing platforms, and so on [Citation1 Citation2013;Citation4]. Ternary or higher nanosheets are particularly attractive since their properties can be tuned by controlling their chemical composition. Further study to explore new nanosheets can accelerate the development of new two-dimensional functional materials.
LnOBiS2 (Ln: La–Sm) species are layered mixed-anion compounds, the crystal structure of which is shown in [Citation5]. LaOBiS2 is a semiconductor with a direct band gap of ca. 1 eV; the bands near the Fermi level consists of Bi 6p and S 3p orbitals of Bi–S along the ab plane [Citation6]. Tuning the properties of LnOBiS2 by changing the anion sites has been reported. The doping of F into the O site increases the energy of the Fermi level, and F-doped LaOBiS2 thus exhibits metallic behavior and superconductivity below 3 K [Citation5,Citation7]. Enhanced thermoelectronic properties have reportedly been achieved by substitution of S for Se [Citation8]. Therefore, nanosheets of LnOBiS2 and related oxychalcogenides may prospectively exhibit various properties including light absorption, electronic conduction, or other unprecedented properties. Moreover, first-principles calculations predict that nanosheets of isostructural SrFBiS2 and BiOBiS2 should exhibit a large Rashba effect [Citation9].
Fig. 1 Crystal structure of LaO0.54F0.46BiS2: (a) view parallel to Bi–S layer and (b) perpendicular to Bi-S layer.
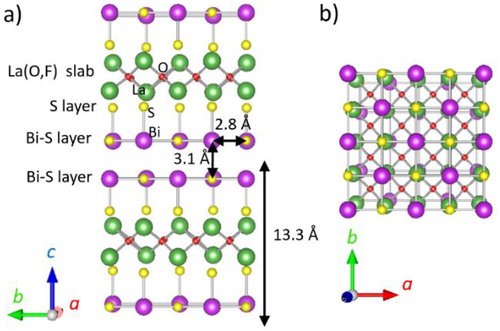
Cleavage between two Bi–S planes of F-doped LnOBiS2 in single crystals occurs with the application of scotch tape; the clean surface of the exfoliated Bi–S plane has been analyzed by scanning tunnelling microscope (STM) and X-ray photoelectron spectroscopy (XPS) analyses [Citation10 Citation2013;Citation12]. Weak interaction is expected between two Bi–S layers owing to the lone pair of the Bi cation [Citation13]. The interplane distance between two Bi–S layers is approximately 3.1 Å for LaO0.54F0.46BiS2, which is much longer than the in-plane Bi–S distance (2.8 Å) [Citation13]. Ultrasonification is a common technique for fabrication of chalcogenide nanosheets, like MoS2 [Citation1], but no reports on F-doped LnOBiS2 nanosheets have been documented. In this study, La O0.5F0.5BiS2 nanosheets are synthesized by ultrasonification in ethanol and characterized by optical absorption, transmission electron and atomic force microscopy.
2 Experimental
LaO0.5F0.5BiS2 powders were synthesized by the solid-state reaction at 700 °C of mixed powders of La2O3, Bi, BiF3, and Bi2S3 in the nominal composition of LaO0.5F0.5BiS2, according to the literature [Citation5]. A synthesized LaO0.5F0.5BiS2 pellet was ground with a mortar and pestle, and 0.1 g of the ground powder was placed in a centrifugal precipitation tube with 40 ml of ethanol. This tube was capped and ultrasonicated at 28 kHz for 60 min. The suspension was centrifuged for 20 min at 550 rpm and allowed to stand overnight. The top and middle portions of the liquid in the suspension were used for further characterization.
The crystal structure of the starting material was examined by X-ray diffraction (XRD; MiniFlex-600). The optical absorption of the suspension was measured using a V-670 UV–vis-NIR spectrophotometer. The morphology and electron diffraction images of the nanosheets were collected using transmission electron microscopy (TEM; JEM-2010). The thickness of the nanosheets was measured by atomic force microscopy (AFM; Nanocute). The nanosheets were affixed to the surface of a Si substrate by the dip-coating technique in conjunction with baking at ca. 80 °C.
3 Results
The XRD pattern () of LaO0.5F0.5BiS2 powder shows the diffraction peaks assigned as La O0.5F0.5BiS2 [Citation5]. The lattice parameters derived from 004 and 110 diffraction peaks were a = 0.4068 nm and c = 1.337 nm, which were close to the reported values (a = 0.40527 nm, c = 1.33247 nm [Citation5]). Minor peaks of Bi metals were detected ().
Ultrasonification of LaO0.5F0.5BiS2 powder in ethanol produced a transparent and slightly reddish suspension. The Tyndall effect was confirmed by passing a green laser beam through the solution, suggesting its colloidal nature (). shows the optical absorption spectrum of the solution. The solution absorbs over a wide range of the visible spectral region, and a broad peak with a maximum at ca. 480 nm (blue absorption) was observed. This would include the optical absorption and scattering of the LaO0.5F0.5BiS2 nanosheets.
Fig. 3 Suspension of LaO0.5F0.5BiS2 nanosheets in ethanol. A green laser beam was passed through the suspension.

shows the TEM image of the synthesized sheets and corresponding electron diffraction pattern. The size of the nonosheet was approximately 300 nm. The electron diffraction spots indicated four-fold symmetry, which can be assigned to the diffraction of the ab-plane of the tetragonal structure of LaO0.5F0.5BiS2. Notably, not all the particles observed by TEM analysis had sheet morphology; some particles were not thin enough to transmit the electron beam, and some particles had a tube-like shape; these were possibly produced by rolling the nanosheets. Energy dispersive X-ray spectroscopy shows peaks of La, O, F, Bi, S, which qualitatively agrees with LaO0.5F0.5BiS2.
Fig. 5 TEM image of LaO0.5F0.5BiS2 nanosheets and corresponding electron diffraction and energy dispersive X-ray spectroscopy spectrum.
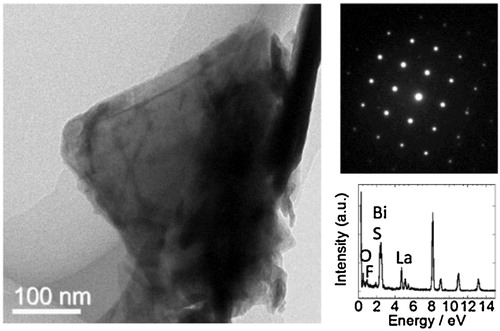
shows AFM images of the LaO0.5F0.5BiS2 sheets on a Si substrate. The thickness of the sheet was 2–6 nm, which corresponded to 2–4 unit cells of the c-axis of LaO0.5F0.5BiS2. Nanosheet assemblies with dimensions of a few tens of microns were observed. Although some aggregated particles were also found, these nanosheet assemblies suggested the potential for the formation of nanosheet films for device application.
4 Summary
The TEM and AFM results suggested that LaO0.5F0.5BiS2 nanosheets were obtained by the ultrasonification route. As reported for many chalcogenide nanosheets [Citation1], fabrication of the sulfide nanosheets, such as MoS2 nanosheets, occurred by exfoliation due to the weak interaction between sulfur anions. In the case of LaO0.5F0.5BiS2, exfoliation can be characterized by breakage of the interaction between the S anion and Bi cation having a lone pair. Further investigation of the synthesis route to achieve increased yield, control of the thickness and size of the nanosheets, as well as measurements of the nanosheet properties are underway.
Acknowledgement
This work is supported by JSPS KAKENHI Grant Number 15K14113.
References
- J.N.ColemanM.LotyaA.O’NeillS.D.BerginP.J.KingU.KhanK.YoungA.GaucherS.DeR.J.SmithI.V.ShvetsS.K.AroraG.StantonH.-Y.KimK.LeeG.T.KimG.S.DuesbergT.HallamJ.J.BolandJ.J.WangJ.F.DoneganJ.C.GrunlanG.MoriartyA.ShmeliovR.J.NichollsJ.M.PerkinsE.M.GrievesonK.TheuwissenD.W.McCombP.D.NellistV.NicolosiScience3312011568571
- X.HuW.ShaoX.HangX.ZhangW.ZhuY.XieAngew. Chem. Int. Ed.55201657335738
- C.TanP.YuY.HuJ.ChenY.HuangY.CaiZ.LuoB.LiQ.LuL.WangZ.LiuH.ZhangJ. Am. Chem. Soc.13720151043010436
- P.RamasamyM.KimH.S.RaJ.KimJ.S.LeeNanoscale8201679067913
- Y.MizuguchiS.DemuraK.DeguchiY.TakanoH.FujihisaY.GotohH.IzawaO.MiuraJ. Phys. Soc. Jpn.812012114725
- A.MiuraY.MizuguchiT.TakeiN.KumadaE.MagomeC.MoriyoshiY.KuroiwaK.TadanagaSolid State Commun.22720161922
- V.P.S.AwanaA.KumarR.JhaS.Kumar SinghA.PalShrutiJ.SahaS.PatnaikSolid State Commun.15720132123
- Y.MizuguchiA.OmachiY.GotoY.KamiharaM.MatobaT.HiroiJ.KajitaniO.MiuraJ. Appl. Phys.1162014163915
- Y.MaY.DaiN.YinT.JingB.HuangJ. Mater. Chem. C2201485398545
- T.MachidaY.FujisawaM.NagaoS.DemuraK.DeguchiY.MizuguchiY.TakanoH.SakataJ. Phys. Soc. Jpn.832014113701
- K.TerashimaJ.SonoyamaT.WakitaM.SunagawaK.OnoH.KumigashiraT.MuroM.NagaoS.WatauchiI.TanakaH.OkazakiY.TakanoO.MiuraY.MizuguchiH.UsuiK.SuzukiK.KurokiY.MuraokaT.YokoyaPhys. Rev. B902014
- S.IshiiY.HonguA.MiuraM.NagaoAppl. Phys. Express92016063101
- A.MiuraM.NagaoT.TakeiS.WatauchiI.TanakaN.KumadaJ. Solid State Chem.2122014213217