Abstract
C10H18N2Na2O10 (ethylenediaminetetra-acetic acid disodium salt) inhibition and adsorption mechanism on the corrosion of steel-reinforcement corrosion in concrete immersed in corrosive environments were investigated in this paper. For this, seven different concentrations ranging from 0% to 0.667% C10H18N2Na2O10 per weight of cement were admixed in steel-reinforced concretes immersed in saline and in acidic sulphate test-media and these were monitored using electrochemical techniques. Statistical analyses of the scatter of measured data from these, as per ASTM G16-95 R04, showed that C10H18N2Na2O10 > 0% admixtures portrayed excellent efficiency at inhibiting steel-reinforcement corrosion in the saline environment. However, attaining comparably high inhibition of steel-reinforcement corrosion in concrete immersed in the acidic sulphate environment exhibited greater dependency on high C10H18N2Na2O10 admixture concentration in the steel-reinforced concretes. Different models of adsorption isotherms bear indications of chemical adsorption, chemisorptions, as the prevalent adsorption mechanism of C10H18N2Na2O10 on steel-reinforcement in both of the corrosive environments.
1 Introduction
Steel reinforced concrete is a material of choice in the construction industry worldwide because of its desirable structural strength properties, relative cost and durability. Usually, steel reinforced concrete durability is ensured by high alkalinity (pH > 12.5) of the concrete pore environment which protects the steel rebar by a thin stable passive oxide film, from hydrated product of cement pastes, strongly adherent to the steel surface (CitationTang et al., 2012). However, environmental agents of corrosion reduce concrete alkalinity and break down the protective passive oxide film on the steel-rebar thus rendering it susceptible to corrosion attacks (Tang et al., Citation2012; Garcés et al., Citation2011"). These environmental agents take the form of carbonation from the atmosphere, chloride ingress from saline (e.g. from de-icing salts) or marine and sulphate attack from acid rain in industrial or microbial activities in sewage environments (Okeniyi et al., Citation2014a; Gerengi et al., Citation2013; De Muynck et al., Citation2009;Tommaselli et al., Citation2009"). By-products from the attacks of these on steel-rebar are expansive within the concrete, induce low structural strength and lead to cracks, spalling, delamination and, if unchecked, eventual catastrophic collapse of the concrete structure. The premature and progressive deterioration of concrete structures and infrastructures due to corrosion of steel reinforcement (steel-rebar) in the concrete militates against safe and sustainable infrastructure worldwide (Dong et al., Citation2012; Feng et al., Citation2011; Fedrizzi et al., Citation2005").
Many studies had deliberated on methods for mitigating corrosion degradation of reinforcing steel in concrete (Okeniyi et al., Citation2014a; Dong et al., Citation2012; Parthiban et al., Citation2008; Valcarce and Vázquez, Citation2008"). Some of the methods proposed include cathodic protection, coatings of the steel-rebar and/or the reinforced concrete, concrete realkalization and the use of admixtures for inhibiting steel rebar corrosion in concrete. Among these methods, the use of corrosion inhibiting admixtures is considered a simple and less costly technique for protecting reinforcing steel, embedded in concrete, from corrosion degradation (CitationFedrizzi et al., 2005).
Generally, corrosion inhibitors could be broadly classified as inorganic and organic (CitationQian and Cusson, 2004). While many inorganic inhibitors like nitrites and chromates had been known to be effective for rebar corrosion protection in concrete they suffered many drawbacks that are limiting their usage in many countries of the world. These drawbacks include environmental restrictions due to toxicity, inhibition mechanism limitation to the anodic sites, corrosion aggravation potency from insufficient quantity applications in the corrosive system and relatively high costs of the inorganic chemicals (Feng et al., Citation2011; Fu et al., Citation2010; Mennucci et al., Citation2009; Vaysburd and Emmons, Citation2004"). In contrast, organic corrosion inhibitors are environmentally friendly, mixed, i.e. both anodic and cathodic, inhibitors and relatively cheaper than their inorganic counterparts (Feng et al., Citation2011; Sastri, Citation2011; Fu et al., Citation2010").
C10H18N2Na2O10 (ethylenediaminetetra-acetic acid disodium salt) is an organic chemical that is non-toxic and non hazardous to the environmental ecosystem and which had been found as a suitable agent in studies for stripping corrosion products from metals (Keny et al., Citation2006; Huda, Citation2002"). Although, CitationQu et al. (2007) had employed C10H18N2Na2O10 for inhibiting metallic corrosion in acidic chloride (HCl), there is still dearth of studies on the inhibition and the adsorption mechanism by C10H18N2Na2O10 on concrete steel-reinforcement in corrosive environments. This paper therefore studies inhibition and adsorption mechanisms of C10H18N2Na2O10 on steel-reinforcement corrosion in concrete slabs immersed in NaCl medium, simulating saline/marine environment and in H2SO4 medium simulating industrial/microbial environment.
2 Materials and methods
2.1 Materials
2.1.1 Chemicals employed
Distilled water was employed for solution preparations (CitationMuralidharan et al., 2004), except for concrete mixing whereby drinkable tap water was used (CitationZafeiropoulou et al., 2011). Also, all chemicals used were of commercially pure quality. These include C10H18N2Na2O10 (ethylenediaminetetra-acetic acid disodium salt) as inhibitor, sodium chloride (NaCl) and sulphuric acid, (H2SO4) as aggressive agents, acetone (C3H6O) for degreasing and iso-propyl alcohol (C3H8O) for wetting fluid.
2.1.2 Steel reinforced concrete materials
Deformed steel-rebar employed in the study was of 12 mm diameter. This has the composition: 0.273% C, 0.403% Si, 0.780% Mn, 0.039% P, 0.037% S, 0.142% Cr, 0.109% Ni, 0.016% Mo, 0.240% Cu, 0.0086% Co, 0.0083% Nb, 0.0063% Sn, 0.0032% V, 0.0037% Ce and the balance Fe. This reinforcing steel was cut, for the corrosion test-experiment, into lengths of 190 mm, and for each of these lengths of rods, surface preparation was maintained uniformly as prescribed from reported study (CitationMuralidharan et al., 2004) and from the specifications of CitationASTM G109-99a (2005). 150 mm length of each steel rod was embedded in each 100 mm × 100 mm × 200 mm concrete block sample, such that the remaining 40 mm steel protrusion could be used for electrochemical connections. This protrusion was painted with glossy paint, for each block after concrete casting.
Four block samples were made in each mould, in a replicated experimental design (CitationHaynie, 2005) that totalled twenty-eight reinforced concrete blocks, for the study. These facilitate seven variations of C10H18N2Na2O10 admixture concentrations, as presented in , whereby duplicate samples of blocks (tagged with “_Dup” or simply “Dup”) with similar C10H18N2Na2O10 concentrations were tested in each aggressive test medium. In the first six moulds, C10H18N2Na2O10 admixtures were varied from 0%, for the blank specimens, in increments of 0.0833% (i.e. a part by weight of C10H18N2Na2O10 in 1200 parts by weight of cement) up to 0.4167% of C10H18N2Na2O10 for each block sample. These translate to 0 M C10H18N2Na2O10 admixture in increment of 0.00448 M C10H18N2Na2O10 to 0.024 M C10H18N2Na2O10 relative to cement mixing water, where 1 M ≡ 1 mol/dm3. The last mould of four blocks had 0.6667% (0.03584 M) of C10H18N2Na2O10 admixture per block sample for studying the effect of such a high concentration on steel-rebar corrosion.
Table 1 Concentrations in wt% of C10H18N2Na2O10 admixtures in steel-reinforced concrete samples.
Formulation of steel-reinforced concrete in the study employed mixed proportion of Ordinary Portland Cement = 300.0 kg/m3, river sand, of 2.80 fineness modulus, conforming to CitationASTM C33-03 (2005), = 890.6 kg/m3, granite stone coarse aggregate (of 7.54 fineness modulus) = 1106.3 kg/m3 and 149.7 kg/m3 of water. The sieve analyses for the fine and coarse aggregates were studied as prescribed in CitationASTM C136-01 (2005). Water-cement ratio employed for the concrete mix = 0.499 (Ormellese et al., Citation2006; ASTM G109-99a, Citation2005"). Preparations and casting of the steel-reinforced concrete specimens were carried out as specified by CitationASTM C192/192M-02 (2005) and as described in CitationOkeniyi et al. (2014b).
2.2 Experimental methods
2.2.1 Setup of concrete test-specimens
The steel reinforced concrete test-specimens were divided into two sets of duplicated specimens. Specimens in each set were immersed, partially along the lengths of the concretes, in plastic bowls containing their respective corrosive test-environments. Samples of the first duplicated set of fourteen specimens were partially immersed in 3.5% NaCl solution (Zhou et al., Citation2012; Zafeiropoulou et al., Citation2011") for simulating saline/marine medium while samples of the second duplicated set were partially immersed in 0.5 M H2SO4 solution (Gerengi et al., Citation2013; Obot and Obi-Egbedi, Citation2010; Moretti et al., Citation2004") for simulating industrial/microbial medium. The corrosive test-environments in the bowls were made up to just below the reinforcing steel but without touching the rebar. These corrosive test-solutions were then replenished every three weeks in the bowls, both for preventing dryness and for ensuring continuity of the corrosive environments in the bowls (CitationMuralidharan et al., 2004).
Non-destructive electrochemical measurements as detailed in the literature (Zafeiropoulou et al., Citation2011; Song and Saraswathy, Citation2007; Broomfield, Citation2003") were taken from each specimen of steel-reinforced concrete, in five day interval for forty days and thereafter in seven day interval for the following three weeks. This totalled sixty-one days. The non-destructive electrochemical test-techniques employed include:
I. | Half-cell potential (HCP), also known as corrosion potential, measurements versus copper/copper sulphate electrode (CSE), as per CitationASTM C876-91 R99 (2005) (Okeniyi et al., Citation2014a,Citationb; Gulikers, Citation2010"). |
II. | Electrochemical cell current (ECC) measurements versus CSE, using zero resistance ammeter (CitationOkeniyi et al., 2013a, Citationb; CitationSastri, 2011; CitationMcCarter and Vennesland, 2004; CitationJäggi et al., 2001). |
III. | Corrosion rate (CR) measurement: was obtained using the 3-electrode LPR Data Logger, Model MS1500L, from Metal Samples® (Okeniyi et al., Citation2014b; Abosrra et al., Citation2011; Sastri, Citation2011"). |
A water retentive conducting sponge was employed for the point of contact of the measuring probes and the reinforced concrete to ensure good electrical contact for the electrochemical measurements (Okeniyi et al., Citation2014a; Gulikers, Citation2010; Song and Saraswathy, Citation2007"). This sponge was wetted before measurements using contact solution consisting of drinkable tap water, small addition of iso-propyl alcohol and some local detergent, as prescribed in CitationASTM C876-91 R99 (2005), for improving the wetting characteristics of the conducting solution.
2.3 Data analyses
2.3.1 Statistical probability distribution analyses
As per CitationASTM G16-95 R04 (2005), the scatter of electrochemical test-data, from each corrosion test variable, was subjected to the descriptive statistics of the Weibull probability density function for detailing prevalent condition in the corrosive test system (Okeniyi et al., Citation2012, Citation2013b; Omotosho et al., Citation2011, Citation2012; Izquierdo et al., Citation2004; Roberge, Citation2003"). This probability density function (pdf) is given by (CitationKotz and Nadarajah, 2000):(1) where x is measured corrosion test data, k is the shape parameter and c is the scale parameter. Estimations of these parameters were obtained, for sample size n = 12 data points of measurements of each electrochemical test-variable, from the solution of the simultaneous maximum likelihood equations (Okeniyi et al., Citation2014b; Reiss and Thomas, Citation2007; Kotz and Nadarajah, Citation2000"):
(2)
(3)
For these, the combined fixed point iterative–Aitken Δ2 procedures were employed (Lange, Citation2010; Hoffman, Citation2001"). The unbiased estimated values of k and c, from these, were used to evaluate the Weibull mean, μ, model of corrosion test variables over the test-period, as proposed by CitationBungey et al. (2006), through (Okeniyi et al., Citation2013b; Omotosho et al., Citation2011"):(4) where
is the gamma function of
.
2.3.2 Goodness-of-fit test statistics
As prescribed in the literature (ASTM G16-95 R04, Citation2005; Roberge, Citation2003"), the Kolmogorov–Smirnov (K–S) goodness-of-fit (GoF) test statistics was employed to verify scattering of experimental data from each electrochemical test-variable like the Weibull distribution at the significant level α = 0.05 (Okeniyi et al., Citation2012, Citation2013b,Citationc; Okeniyi and Okeniyi, Citation2012; Roberge, Citation2003"). The K-S GoF method measures the absolute difference between empirical distribution function F∗(x) and theoretical distribution function F(x), for n data points, using:(5)
2.3.3 Testing of statistical significance between steel-reinforced concrete samples
Significance of differences between corrosion test-data of the duplicated samples of each admixture concentration was investigated by using the student’s t-test statistics (CitationASTM G16-95 R04, 2005). This includes the homoscedastic (hom) t-test of equal variance assumption, and the heteroscedastic (het) t-test of unequal variance assumption. This t-test statistics finds usefulness for indicating whether differences in test-responses encountered in duplicated samples were due to chance and thus not significant or otherwise.
2.3.4 Surface coverage and inhibition efficiency
The mean model, μ, of the Weibull fittings of corrosion rate test data was employed for evaluating the surface coverage, θ, and the inhibition efficiency, η, for each concentration of C10H18N2Na2O10 admixture in concrete relative to the blank using the relationships (Anejjar et al., Citation2014; Karthikaiselvi and Subhashini, Citation2014; Singh et al., Citation2012"):(6)
(7)
2.3.5 Modelling of adsorption mechanism
For studying the mechanisms of C10H18N2Na2O10 adsorption on the metallic steel-reinforcement three models of adsorption isotherms were fitted to the experimental data and the concentration of C10H18N2Na2O10 in mol/dm3. These include the Langmuir, the El-Awady and the Freundlich models of adsorption isotherms. For these adsorption isotherm models, characteristic equations and parameter estimations/interpretations that have been detailed in studies (Anejjar et al., Citation2014; Karthikaiselvi and Subhashini, Citation2014; Obi-Egbedi et al., Citation2012; Singh et al., Citation2012; Foo and Hameed, Citation2010; Vijayaraghavan et al., Citation2006") were employed.
In addition, the Langmuir model of adsorption isotherm facilitates estimation of the separation factor, RL, using the relationship (CitationFoo and Hameed, 2010):(8) where Kads is the Langmuir equilibrium constant of the adsorption-desorption process; RL a dimensionless constant for indicating C10H18N2Na2O10 adsorption nature on steel-rebar as either irreversible if RL = 0 or favourable if 0 < RL < 1 or linear if RL = 1 or unfavourable if RL > 1.
Also from the Langmuir and the El-Awady models of adsorption isotherms, the Gibbs free energy of adsorption was calculated from its relationship with Kads according to (Anejjar et al., Citation2014; Karthikaiselvi and Subhashini, Citation2014"):
(9) where R = 8.314J/mol·K is the molar gas constant, T is absolute temperature (K) and 55.5 is the concentration of water in solution expressed in molar. The Gibbs free energy of adsorption
finds usefulness for indicating adsorption mechanism on the metallic surface. For instance, it is well known that value of
around −20 kJ/mol or lower is indicative of physical adsorption mechanism or physisorption while value around −40 kJ/mol or higher is indicative of chemical adsorption mechanism or chemisorptions (Anejjar et al., Citation2014; Karthikaiselvi and Subhashini, Citation2014; Obi-Egbedi et al., Citation2012; Singh et al., Citation2012").
Also, the value of 1/λ, the slope obtained from the fitting of Freundlich isotherm model, is useful for indicating adsorption mechanism on the metallic surface. Value of 1/λ < 1 implies chemical adsorption mechanism, chemisorptions, while 1/λ > 1 indicates cooperative adsorption effects of physical adsorption mechanism, physisorption (CitationFoo and Hameed, 2010). This was therefore employed in this study for comparison with the adsorption mechanism interpreted from the Gibbs free energy of adsorption from the Langmuir and the El-Awady models of adsorption isotherm.
3 Results and discussion
3.1 Results of statistical analyses of corrosion test data
Results of the analysis techniques of the Weibull probability distribution function for the corrosion test data of electrochemical variables are presented as graphical plots in for steel-reinforced concrete samples immersed in the NaCl and in H2SO4 media. From these plots, it is worth noting that the Weibull mean of test responses from the blank samples in NaCl medium exhibited higher severity of corrosive activities than the Weibull mean of test-responses from the blank samples in H2SO4 medium. This could be due to the availability of chloride ions for continuous dissolutions and corrosion accelerations of concrete steel-reinforcement in the NaCl corrosive environment because they, the chloride ions, were not consumed in the corrosion process (Wei et al., Citation2013; Fontana, Citation1987"). In contrast, the acidic sulphate ions were usually consumed in concrete attacks even before the ions could attain the steel-rebar for corrosion attacks (CitationHewayde et al., 2007).
Figure 1 Graphical plots of Weibull mean of electrochemical test-data from steel-reinforced concretes (a) half-cell potential (HCP) (b) electrochemical cell current (ECC) (c) corrosion rate (d) corrosion rate for samples in NaCl medium without the blank samples (e) corrosion rate of samples in H2SO4 medium including the blank samples in the medium.
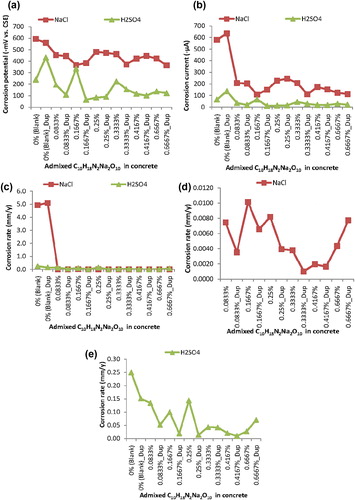
It could also be noted that the plots of Weibull mean of half-cell potential in (a) find pattern agreements with the plots of Weibull mean of electrochemical cell current in (b). However, the high corrosion rates from the blank samples in NaCl medium make visualising the pattern of the Weibull mean of corrosion rate from the blank samples in H2SO4 and the C10H18N2Na2O10 admixed samples in both corrosive media impossible as shown in (c). This necessitates the plotting of the corrosion rate from the C10H18N2Na2O10 admixed samples, without the high corrosion rate-valued blank samples, in NaCl medium in (d) and the plotting of the samples (including the blanks) in H2SO4 medium in (e). These latter figures bear indications of more reductions in the corrosion rate of concrete steel-reinforcement in C10H18N2Na2O10 admixed samples in NaCl medium than the corrosion rate of concrete steel-reinforcement in C10H18N2Na2O10 admixed samples in H2SO4 medium.
For instance, the highest corrosion rate, of 0.0102 mm/y from the 0.1667% C10H18N2Na2O10 admixed sample, among the other admixed samples in NaCl medium, just surpassed the “0.001–0.01 mm/y” “low/moderate” range of corrosion rate classifications in the literature (Söylev et al., Citation2007; Bungey et al., Citation2006; Millard et al., Citation2001"). This was in spite of the corrosion rate models of the blank samples in the NaCl medium which surpassed the “0.1–1 mm/y” that represented the “very high” corrosion rate classifications from the cited studies. In contrast, while the corrosion rate of the blank samples in H2SO4 medium classified to the “very high” corrosion rate, only the 0.4167% C10H18N2Na2O10 admixed sample in the H2SO4 exhibited corrosion rate of 0.0095 mm/y that classified to the “low/moderate” corrosion rate. The other C10H18N2Na2O10 admixed samples in the acidic medium were modelled with corrosion rate ranging from the “very high” to the “high” rate of corrosion classifications. It is worth noting that both the beyond “very high” corrosion rate by the blank samples in the NaCl and the “very high” corrosion rate from the blank samples in the H2SO4 medium lay credence that the two test-media represented severe conditions of corrosive test-systems. These therefore followed the prescription of the preferred practice by CitationRoberge (2003) for reducing the time for effects to be observed so that the dominant factor could be employed as the rank ordering factor of performance in the conduct of laboratory corrosion tests. However, these prevailing corrosion conditions in each of the corrosive test-systems, by the Weibull mean models of corrosion test-data, necessitate ascertaining that the fitted test-data by the Weibull descriptive statistics scatter like the Weibull probability distribution function.
The results of K–S GoF test applications to the Weibull fitting of corrosion test-data are presented in for steel-reinforced concrete samples immersed in the corrosive environments of NaCl and of H2SO4 medium. In the figure, the linear plot of significant level α = 0.05 was included for easy ascertaining of test-data not scattering like the Weibull probability distribution function. From the plots, it could be noted that the K–S p-value > 0.05 for the test-data of corrosion test-variables subjected to the fittings of the Weibull probability distribution function. These bear indications that the scatter of non-destructive electrochemical test-data in this study distributed like the Weibull probability distribution thus bearing support for the suitability of the Weibull distribution as the statistics for describing the test-data.
Figure 2 Kolomogorov-Smirnov goodness-of-fit (K-S GoF) test results of the Weibull fittings of the test-data from steel-reinforced concrete samples in corrosive environments.
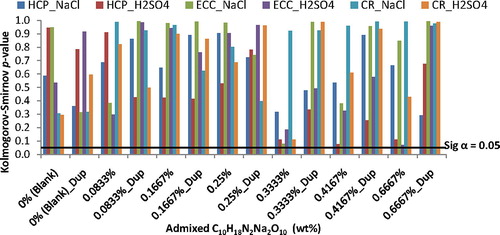
Although the K–S GoF statistics showed that the test-data of corrosion variables from samples in the corrosive media scattered like the Weibull distribution, the Weibull mean of corrosion responses from duplicates of concrete samples still exhibited differences. For this, it is further needful to ascertain whether the between-duplicate differences encountered in the Weibull mean of corrosion response from the steel-reinforced concrete duplicates were due to chance or were significant.
The results of student’s t-test statistics using the homoscedastic (hom) and the heteroscedastic (het) applications to the corrosion test-data of the duplicates of steel-reinforced concrete samples were presented in graphical plots in . In this figure, the linear plots of significant level α = 0.05 were also included for direct identification of significance of differences from the charts. From this, it could be observed that except for the ECC of the 0.3333% C10H18N2Na2O10, the differences in test-data of the other corrosion test-variables encountered between the duplicates of the concrete samples in NaCl medium were due to chance but were not significant. While the ECC of the 0.3333% C10H18N2Na2O10 exhibited p-value ≈ 0.02 < 0.05, data of other test-variables from the NaCl-immersed samples exhibited p-values > 0.05 by student’s t-test statistics of the homoscedastic (hom) and the heteroscedastic (het) applications, (a). By this, the differences encountered in the ECC measurements of the duplicate samples admixed with 0.3333% C10H18N2Na2O10 were not due to chance but were significant at α = 5% level of significance.
Figure 3 Plots of student’s t-test statistics for significance of differences between replicates of steel-reinforced concrete samples (a) in NaCl medium (b) in H2SO4 medium.
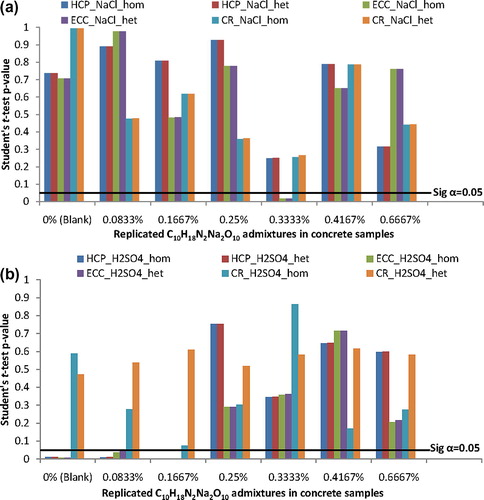
Also, (b) showed that the between-duplicate differences encountered in the HCP test-data of the 0% (the blank samples), the 0.0833% C10H18N2Na2O10 and of the 0.1667% C10H18N2Na2O10 admixed concretes in H2SO4 medium were not due to chance but were significant. It could also be deduced from (b) that the ECC test-data from the concrete samples admixed with these 0% (blank), 0.0833% and 0.1667% C10H18N2Na2O10 admixtures in H2SO4 medium exhibited differences that were not due to chance but that were significant. The p-values from student’s t-test statistics were less than the significant level of 0.05 by the “hom” and by the “het” t-test applications.
However, student’s t-test results in (a), from the NaCl-immersed samples, and in (b), from the H2SO4-immersed samples, showed that the differences encountered in the corrosion rate of the duplicate samples were due to chance but were not significant. This bears support for the electrochemical test-variable of corrosion rate as the dominant factor that could be used as rank ordering factor, as per CitationRoberge (2003), for detailing C10H18N2Na2O10 performance on steel-reinforcement corrosion in the corrosive environments.
3.2 Inhibition effectiveness ranking
The lack of significant differences in the corrosion rate test-response from the duplicates of steel-reinforced concretes being studied facilitates estimations of averaged corrosion rate from each duplicate of steel-reinforced concretes in each of the corrosive test-media. To these averages, Eq. Equation(7)(7) was relevantly applied for evaluating inhibition efficiency, η, by each of the admixed C10H18N2Na2O10 concentrations on the concrete steel-reinforcement corrosion in the corrosive test-media. These inhibition efficiency results of C10H18N2Na2O10 admixture on concrete steel-reinforcement corrosion are presented in ranking order of inhibition effectiveness in .
Figure 4 Inhibition efficiency ranking of C10H18N2Na2O10 admixtures on concrete steel-reinforcement corrosion (a) in NaCl medium (b) in H2SO4 medium.
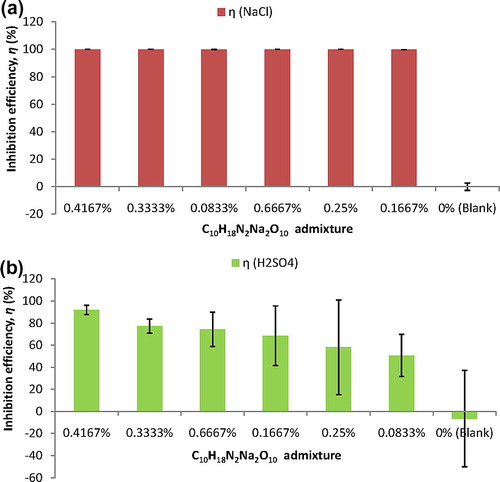
From (a), it could be noted that C10H18N2Na2O10 admixtures exhibited very strong effectiveness at inhibiting concrete steel-reinforcement corrosion in the also highly aggressive NaCl medium. Also, the inhibition efficiency by C10H18N2Na2O10 admixture on concrete steel-reinforcement corrosion could be described as invariant to the concentrations of C10H18N2Na2O10 admixed in concrete. In spite of this concentration invariance, however, the 0.4167% C10H18N2Na2O10 admixture exhibited optimal inhibition effectiveness, η = 99.96 ± 0.004%, at inhibiting concrete steel-reinforcement in the saline/marine simulating environment. The 0.1667% C10H18N2Na2O10 admixture exhibited inhibition efficiency of η = 99.83 ± 0.04% as the least effective among the C10H18N2Na2O10 admixtures in the NaCl medium.
The kind of concentration invariance encountered in the NaCl medium was not exhibited by C10H18N2Na2O10 admixture on concrete steel-reinforcement corrosion in the H2SO4 medium, (b). Although, the 0.4167% C10H18N2Na2O10 admixture still exhibited optimal inhibition efficiency of η = 92.04 ± 4.13%, the 0.0833% C10H18N2Na2O10 admixture exhibited least effectiveness of η = 50.67 ± 19.04%, at inhibiting reinforcing steel corrosion in the H2SO4 medium. It is worth noting from (b) that inhibition efficiency of η > 74% was attained by the higher C10H18N2Na2O10 concentrations, i.e. the 0.4167%, the 0.3333% and the 0.6667% C10H18N2Na2O10 admixtures. These bear suggestions that high C10H18N2Na2O10 concentrations were required for high effectiveness of the C10H18N2Na2O10 admixture at inhibiting concrete steel-reinforcement corrosion in H2SO4 medium.
3.3 Mechanism of C10H18N2Na2O10 adsorption on steel-reinforcement
The resulting parameters from the fittings of the different adsorption isotherm models to the experimental performance of C10H18N2Na2O10 admixtures on steel-reinforcement corrosion are presented in . From this, the Langmuir isotherm model could be observed as the best-fitting model of C10H18N2Na2O10 adsorption on concrete steel-reinforcement in the NaCl environment with R2 = 0.9999 and in the H2SO4 environment with R2 = 0.9520.
Table 2 Estimated parameters for models of adsorption isotherm.
Apart from the non-fittings of experimental performance of C10H18N2Na2O10 by the El-Awady and the Freundlich isotherm models, they still bear supports for important inferences that could be drawn from them and from the Langmuir isotherm, which include:
• | Multilayer of C10H18N2Na2O10 was formed on the metal surface in both corrosive media because “1/y > 1” from the El-Awady isotherm; |
• | The adsorption was favourable in both media because “0 < RL < 1” from the Langmuir isotherm; |
• | The adsorption process was spontaneous and the adsorbed C10H18N2Na2O10 layer was stable on the metallic surface in both corrosive media due to the negative values of |
• | The adsorption mechanisms of C10H18N2Na2O10 on concrete steel-reinforcement were more indicative of chemical adsorption or chemisorptions as being prevalent in both corrosive test-media, based on the Freundlich slope “1/λ < 1” and the |
4 Conclusions
Inhibition and adsorption mechanisms of concentrations of C10H18N2Na2O10 admixtures on steel-reinforcement corrosion in concrete immersed in NaCl and H2SO4 corrosive environments had been studied in this work. The conclusions that could be drawn from these include:
• | Data of non-destructive electrochemical test-measurements from the study distributed like the Weibull probability distribution according to the Kolmogorov-Smirnov test-statistics, thus supporting the use of the Weibull distribution fittings as the descriptive statistics for detailing prevailing corrosion conditions in the corrosive test-systems; |
• | Test-data of corrosion rate exhibited differences that were not significant between duplicated steel-reinforced concrete samples admixed with similar C10H18N2Na2O10 concentrations, in the same test-media, according to student’s t-test statistics of the homoscedastic and heteroscedastic assumptions at α = 0.05 significant level; |
• | Although, all the C10H18N2Na2O10 admixture concentrations in this study exhibited very high, η > 99%, inhibition efficiency in concrete immersed in NaCl medium, the 0.4167% C10H18N2Na2O10 admixture exhibited optimal effectiveness, η = 99.96 ± 0.004%, at inhibiting steel-reinforcement corrosion in concrete immersed in this saline/marine simulating environment; |
• | The 0.4167% C10H18N2Na2O10 admixture also exhibited optimal effectiveness, η = 92.04 ± 4.13% at inhibiting steel-reinforcement corrosion in concrete immersed in H2SO4 medium, where high concentrations, the 0.4167%, the 0.3333% and the 0.6667% of C10H18N2Na2O10 were required to attain inhibition efficiency of η > 74%; |
• | Adsorption modelling showed that C10H18N2Na2O10 admixtures were best fitted by the Langmuir isotherm even as the other two isotherm models employed, the El-Awady and the Freundlich, bear supports with the Langmuir model at indicating chemical adsorption, chemisorptions, as the prevalent adsorption mechanism of C10H18N2Na2O10 on concrete steel-reinforcement in both the NaCl and the H2SO4 corrosive environments. |
Notes
Peer review under responsibility of University of Bahrain.
References
- L.AbosrraA.F.AshourM.YouseffiCorrosion of steel reinforcement in concrete of different compressive strengthsConstr. Build. Mater.25201139153925
- A.AnejjarR.SalghiA.ZarroukO.BenaliH.ZarrokB.HammoutiE.E.EbensoInhibition of carbon steel corrosion in 1 M HCl medium by potassium thiocyanateJ. Assoc. Arab Univ. Basic Appl. Sci.1520142127
- ASTM C136-01, 2005. Standard Test Method for Sieve Analysis of Fine and Coarse Aggregates, ASTM International, West Conshohocken, PA.
- ASTM C192/192M-02, 2005. Standard practice for making and curing concrete test specimens in the laboratory, ASTM International, West Conshohocken, PA.
- ASTM C33-03, 2005. Standard Specification for Concrete Aggregates, ASTM International, West Conshohocken, PA.
- ASTM C876-91 R99, 2005. Standard test method for half-cell potentials of uncoated reinforcing steel in concrete, ASTM International, West Conshohocken, PA.
- ASTM G109-99a, 2005. Standard test method for determining the effects of chemical admixtures on the corrosion of embedded steel reinforcement in concrete exposed to chloride environments, ASTM International, West Conshohocken, PA.
- ASTM G16-95 R04, 2005. Standard guide for applying statistics to analysis of corrosion data, ASTM International, West Conshohocken, PA.
- J.P.BroomfieldCorrosion of Steel in Concrete: Understanding, Investigation and Repair2003Taylor & FrancisNew York
- J.H.BungeyS.G.MillardM.G.GranthamTesting of Concrete in Structuresfourth ed.2006Taylor & FrancisNew York
- W.De MuynckN.De BelieW.VerstraeteEffectiveness of admixtures, surface treatments and antimicrobial compounds against biogenic sulfuric acid corrosion of concreteCement Concr. Compos.312009163170
- S.DongB.ZhaoC.LinR.DuR.HuG.X.ZhangCorrosion behavior of epoxy/zinc duplex coated rebar embedded in concrete in ocean environmentConstr. Build. Mater.2820127278
- L.FedrizziF.AzzoliniP.L.BonoraThe use of migrating corrosion inhibitors to repair motorways’ concrete structures contaminated by chloridesCem. Concr. Res.352005551561
- L.FengH.YangF.WangExperimental and theoretical studies for corrosion inhibition of carbon steel by imidazoline derivative in 5% NaCl saturated Ca(OH)2 solutionElectrochim. Acta582011427436
- M.G.FontanaCorrosion Engineeringthird ed.1987McGraw-HilI Book CoSingapore
- K.Y.FooB.H.HameedInsights into the modeling of adsorption isotherm systemsChem. Eng. J.1562010210
- J.J.FuS.N.LiL.H.CaoY.WangL.H.YanL.D.LuL-tryptophan as green corrosion inhibitor for low carbon steel in hydrochloric acid solutionJ. Mater. Sci.452010979986
- P.GarcésP.SauraE.ZornozaC.AndradeInfluence of pH on the nitrite corrosion inhibition of reinforcing steel in simulated concrete pore solutionCorros. Sci.53201139914000
- H.GerengiY.KocakA.JazdzewskaM.KurtayH.DurgunElectrochemical investigations on the corrosion behaviour of reinforcing steel in diatomite- and zeolite-containing concrete exposed to sulphuric acidConstr. Build. Mater.492013471477
- J.GulikersStatistical interpretation of results of potential mapping on reinforced concrete structuresEur. J. Environ. Civil Eng.142010441466
- F.H.HaynieStatistical treatment of data, data interpretation, and reliabilityR.BaboianCorrosion Tests and Standards: Application and Interpretationsecond ed.2005ASTM InternationalWest Conshohocken, PA8388
- E.HewaydeM.L.NehdiE.AlloucheG.NakhlaUsing concrete admixtures for sulphuric acid resistanceProc. Inst Civil Eng. Constr. Mater.16020072535
- J.D.HoffmanNumerical Methods for Engineers and Scientistssecond ed.2001Marcel Dekker IncNew York
- K.HudaA note on the efficacy of ethylenediaminetetra-acetic acid disodium salt as a stripping agent for corrosion products of copperStud. Conserv.472002211216
- D.IzquierdoC.AlonsoC.AndradeM.CastellotePotentiostatic determination of chloride threshold values for rebar depassivation: experimental and statistical studyElectrochim. Acta49200427312739
- Jäggi, S., Bohni, H., Elsener, B., 2001. Macrocell corrosion of steel in concrete–experimental and numerical modelling. In: Proceedings of Eurocorr 2001, Riva di Garda, Italy, Associazione Italiana Metallurgia (AIM), Milan, Italy.
- R.KarthikaiselviS.SubhashiniStudy of adsorption properties and inhibition of mild steel corrosion in hydrochloric acid media by water soluble composite poly (vinyl alcohol-o-methoxy aniline)J. Assoc. Arab Univ. Basic Appl. Sci1620147482
- S.J.KenyJ.ManjannaG.VenkateswaranR.KameswaranDissolution behavior of synthetic Mg/Zn-ferrite corrosion products in EDTA and NTA based formulationsCorros. Sci.48200627802798
- S.KotzS.NadarajahExtreme Value Distributions: Theory and Applications2000Imperial College PressLondon
- K.LangeNumerical Analysis for Statisticianssecond ed.2010Springer Science+Business Media, LLCNew York
- W.J.McCarterØ.VenneslandSensor systems for use in reinforced concrete structuresConstr. Build. Mater.182004351358
- M.M.MennucciE.P.BanczekP.R.P.RodriguesI.CostaEvaluation of benzotriazole as corrosion inhibitor for carbon steel in simulated pore solutionCement Concr. Compos.312009418424
- S.G.MillardD.LawJ.H.BungeyJ.CairnsEnvironmental influences on linear polarisation corrosion rate measurement in reinforced concreteNDT&E Int.342001409417
- G.MorettiF.GuidiG.GrionTryptamine as a green iron corrosion inhibitor in 0.5 M deaerated sulphuric acidCorros. Sci.462004387403
- S.MuralidharanV.SaraswathyS.P.Merlin NimaN.PalaniswamyEvaluation of a composite corrosion inhibiting admixtures and its performance in Portland pozzolana cementMater. Chem. Phys.862004298306
- N.O.Obi-EgbediI.B.ObotS.A.UmorenSpondias mombin L. as a green corrosion inhibitor for aluminium in sulphuric acid: correlation between inhibitive effect and electronic properties of extracts major constituents using density functional theoryArabian J. Chem.52012361373
- I.B.ObotN.O.Obi-Egbedi2,3-Diphenylbenzoquinoxaline: a new corrosion inhibitor for mild steel in sulphuric acidCorros. Sci.522010282285
- J.O.OkeniyiO.A.OmotoshoO.O.AjayiC.A.LotoEffect of potassium-chromate and sodium-nitrite on concrete steel-rebar degradation in sulphate and saline mediaConstr. Build. Mater.502014448456
- J.O.OkeniyiI.J.AmbroseS.O.OkpalaO.M.OmoniyiI.O.OladeleC.A.LotoP.A.I.PopoolaProbability density fittings of corrosion test-data: implications on C6H15NO3 effectiveness on concrete steel-rebar corrosionSādhanā – Acad. Proc. Eng. Sci.392014731764
- J.O.OkeniyiI.O.OladeleI.J.AmbroseS.O.OkpalaO.M.OmoniyiC.A.LotoA.P.I.PopoolaAnalysis of inhibition of concrete steel-rebar corrosion by Na2Cr2O7 concentrations: implications for conflicting reports on inhibitor effectivenessJ. Cent. S. Univ.20201336973714
- J.O.OkeniyiI.J.AmbroseI.O.OladeleC.A.LotoP.A.I.PopoolaElectrochemical performance of sodium dichromate partial replacement models by triethanolamine admixtures on steel-rebar corrosion in concretesInt. J. Electrochem. Sci.820131075810771
- J.O.OkeniyiU.E.ObiajuluA.O.OgunsanwoN.W.OdiaseE.T.OkeniyiCH4 emission model from the waste of sus domesticus and gallus domesticus in Nigerian local farms: environmental implications and prospectsMitig. Adapt. Strat. Glob. Change182013325335
- J.O.OkeniyiE.T.OkeniyiImplementation of Kolmogorov-Smirnov P-value computation in Visual Basic®: implication for Microsoft Excel® library functionJ. Stat. Comput. Simul.82201217271741
- J.O.OkeniyiO.A.OmotoshoO.O.AjayiO.O.JamesC.A.LotoModelling the performance of sodium nitrite and aniline as inhibitors in the corrosion of steel-reinforced concreteAsian J. Appl. Sci.52012132143
- O.A.OmotoshoC.A.LotoO.O.AjayiJ.O.OkeniyiAniline effect on concrete steel rebar degradation in saline and sulfate mediaAgric. Eng. Int.: CIGR J.132011117
- O.A.OmotoshoJ.O.OkeniyiO.O.AjayiC.A.LotoEffect of synergies of K2Cr2O7, K2CrO4, NaNO2 and aniline inhibitors on the corrosion potential response of steel reinforced concrete in saline mediumInt. J. Environ. Sci.22012220226
- M.OrmelleseM.BerraF.BolzoniT.PastoreCem. Concr. Res.362006536547
- G.T.ParthibanT.ParthibanR.RaviV.SaraswathyN.PalaniswamyV.SivanCathodic protection of steel in concrete using magnesium alloy anodeCorros. Sci.50200833293335
- S.QianD.CussonElectrochemical evaluation of the performance of corrosion-inhibiting systems in concrete bridgesCement Concr. Compos.262004217233
- Q.QuS.JiangW.BaiL.LiEffect of ethylenediamine tetraacetic acid disodium on the corrosion of cold rolled steel in the presence of benzotriazole in hydrochloric acidElectrochim. Acta52200768116820
- R.D.ReissM.ThomasStatistical Analysis of Extreme Valuesthird ed.2007Birkhäuser Verlag AGBasel, Switzerland
- P.R.RobergeStatistical interpretation of corrosion test resultsS.D.CramerB.S.CovinoJr.ASM Handbook, A – Corrosion: Fundamentals, Testing, and Protectionvol. 132003ASM InternationalMaterials Park, OH425429
- V.S.SastriGreen Corrosion Inhibitors: Theory and Practice2011John Wiley & Sons IncHoboken, New Jersey
- A.K.SinghS.K.ShuklaM.A.QuraishiE.E.EbensoInvestigation of adsorption characteristics of N,N′-[(methylimino)dimethylidyne]di-2,4-xylidine as corrosion inhibitor at mild steel/sulphuric acid interfaceJ. Taiwan Inst. Chem. Eng.432012463472
- H.-W.SongV.SaraswathyCorrosion monitoring of reinforced concrete structures: a reviewInt. J. Electrochem. Sci.22007128
- T.A.SöylevC.McNallyM.RichardsonEffectiveness of amino alcohol-based surface-applied corrosion inhibitors in chloride-contaminated concreteCem. Concr. Res.372007972977
- Y.M.TangY.F.MiaoY.ZuoG.D.ZhangC.L.WangCorrosion behavior of steel in simulated concrete pore solutions treated with calcium silicate hydratesConstr. Build. Mater.302012252256
- M.A.G.TommaselliN.A.MarianoS.E.KuriEffectiveness of corrosion inhibitors in saturated calcium hydroxide solutions acidified by acid rain componentsConstr. Build. Mater.232009328333
- M.B.ValcarceM.VázquezCarbon steel passivity examined in alkaline solutions: the effect of chloride and nitrite ionsElectrochim. Acta53200850075015
- A.M.VaysburdP.H.EmmonsCorrosion inhibitors and other protective systems in concrete repair: concepts or misconceptsCement Concr. Compos.262004255263
- K.VijayaraghavanT.V.N.PadmeshK.PalaniveluM.VelanBiosorption of nickel(II) ions onto Sargassum wightii: application of two-parameter and three-parameter isotherm modelsJ. Hazard. Mater.B1332006304308
- J.WeiJ.H.DongW.KeCorrosion evolution of scaled rebar in concrete under dry/wet cyclic condition in 3.5% NaCl solutionInt. J. Electrochem. Sci.8201325362550
- T.ZafeiropoulouE.RakantaG.BatisPerformance evaluation of organic coatings against corrosion in reinforced cement mortarsProg. Org. Coat.722011175180
- X.ZhouH.YangF.WangInvestigation on the inhibition behavior of a pentaerythritol glycoside for carbon steel in 3.5% NaCl saturated Ca(OH)2 solutionCorros. Sci.542012193200