Abstract
Systematic Evolution of Ligands by EXponential enrichment (SELEX) is the method to select the specific aptamer against a wide range of targets. For this process, the initial library usually has a length of random sequences from ∼25 and it reaches over 100 bases. The lengthy sequences have disadvantages such as difficult to prepare, less stable and expensive. It is wise to prefer shorter version of aptamer for a wide range of applications including drug delivery process. It is a common practice to shorten the full-length aptamer by mapping analyses and it is tedious. Here, we used a crawling method to shorten the aptamer by different sequential deletion of bases from both 5′ and 3′ ends, assisted by Mfold web server application. Two different kinds of aptamer with varied lengths (randomized region of 30 and 74 bases) were desired for this study, generated against Influenza A/Panama/2007/1999 (H3N2) and gD protein of Herpes Simplex Virus-1. It was found that shortening the aptamer length by crawling pattern is possible with the assistance of Mfold web server application. The obtained results resemble the shortened aptamer derived by mapping analyses. The proposed strategy is recommended to predict the shorter aptamer without involving any wet experimental section.
1 Introduction
Aptamer is an artificial ligand generated from the random library of molecules by iterative rounds of complex formation followed by separation and amplification of bound molecules (complexed with the target), by the method called ‘SELEX’ (Systematic Evolution of Ligands by EXponential enrichment) (Gopinath, Citation2007a; Lakshmipriya et al., Citation2013a, Citation2013b; Lozupone et al., Citation2003; Szeto et al., Citation2013). Aptamers have advantages over antibodies with attractive characteristics, such as easy to prepare, low cost, non-immunogenic and easy to modify. There are various kinds of aptamer that have been generated against a wide range of targets from a small molecule to a whole cell. The selected aptamers have applications in different fields such as environmental, medical and sensor applications (Gopinath et al., Citation2008, Citation2012a; Gopinath, Citation2007b; Ni et al., Citation2011; Yang et al., Citation2015). Moreover, in the field of therapeutics and drug delivery aptamers have been highly involved. With all these purposes, if we use aptamer as full-length, they will have hurdles such as short lifespan, expensive in preparation and larger in size.
Generally, SELEX method starts from the highly enriched pool with the desired length of molecules. It is believed that with longer sequences, there are more chances to find the aptamer, due to the possibilities of different structural variations. However, it is also dependent on the quality of prepared pools, in some cases, lengths with even ∼25 bases have been used for the efficient aptamer selection. In any case, after selecting the aptamer the length is needed to be shortened by the laborious strategies such as mapping or footprinting analysis. Mapping analysis involves either enzymatic digestions or other chemical treatments to reveal the region of aptamer binding on the target (Citationb0005). After confirming the binding region(s), it is easier to eliminate the unreacted bases from the lengthier aptamer. But mapping methods need experimental skill and are time consuming and expensive. To replace this routine route, herein we introduced a crawling method using Mfold web server to predict the shorter aptamer, which keeps the original activity same as the full length. In which, we eliminated the bases gradually from both ends 5′ and 3′ of the aptamer. For these analyses, we have chosen two different RNA aptamers selected against Influenza A/Panama/2007/1999 (H3N2) and gD protein from Herpes Simplex Virus-1 (Gopinath et al., Citation2006, Citation2012b), having different lengths of random sequences with N30 and N74, respectively. RNA aptamers have been chosen for this study due to their versatility to generate multiple secondary structures rather than DNA aptamers, leading to the selection of high-affinity aptamers. The method shown here is very useful to shorten the generated aptamer sequences without involving laboratory experiments.
2 Experimental analyses
Mfold web server online application was used to predict the secondary structures of aptamers selected for the analyses. Two aptamer sequences, namely anti-Influenza A/Panama/2007/1999 (H3N2) (GGGAGAAUUCCGACCAGAAGGGUUAGCAGUCGGCAUGCGGUACAGACAGACCUUUCCUCUCUCCUUCCUCUUCU) and anti-gD protein from Herpes Simplex Virus (GGGAGCUCAGCCUUCACUGCACGAGAGAGGUCGUCCCCAGGGGAGAACUCGUGCUCCUGGAGGCAAGUUGACUGCUCGCUCUCCGCUGGUCAAG) were chosen for this study. Crawling method by deleting bases from both ends of aptamer were applied. For this analysis, after entering the web server, RNA folding form has been selected and the sequences to be analyzed had been pasted in the space provided. Pasted sequences were folded under the suggested default parameters. The output secondary structures were collected and analyzed for the presence of stems and loops. Similar parameters have been applied for all other structures that were analyzed.
Energy distributions were obtained from the energy dot blot generated from the Mfold web server. It displays two triangles, lower triangle shows the optimal energy and upper triangle shows the plotted base pairs. Square symbols in the triangles appear in different colors; red indicates optimal energy; other colors indicate reduction in the energy level in descending order from red to orange, yellow, green, cyan, blue, violet and black.
2.1 Determination of dissociation constant (Kd)
Aptamer-target (Hemagglutinin of Influenza A/Panama/2007/1999 (H3N2) or gD protein of Herpes Simplex Virus-1) interactions were analyzed using a BIAcore T100 or BIAcore 2000 optical system at room temperature on Streptavidin modified sensor chip (SA chip, GE Healthcare) (Gopinath et al., Citation2006, Citation2012b). To determine the binding rate constants of the aptamers, we prepared the aptamers with 24-mer poly(A) nucleotides at their 3′ ends, which could anneal to the complementary biotinylated oligo(dT) [5′-Biotin-(T)24-3′]. To prepare this RNA with the 3′ end extension, we used a forward primer and a reverse primer [5′-(T)24-reverse primer sequences-3′]. The double-stranded DNA template was generated by PCR and transcribed in vitro. Initially, 5 μM biotinylated oligo (dT)24 was attached to the streptavidin by dissolving the oligo in binding buffer. The excess or unbound biotinylated oligo was washed away with binding buffer. To analyze the binding kinetics, 20 μl aptamer was injected (100 nM final concentration) at a flow rate of 2 μl/min for 10 min. Single cycle kinetics or multi cycle kinetics were performed with various concentrations of target (10, 20, 40, 80 and 160 nM) by injecting the protein through both the experimental flow-cell (FC2) and the control flow-cell (FC1) with a flow rate of 30 μl/min for 2 min. Sensorgrams were corrected for specific binding by subtracting the aptamer attached curve (FC2) by the control curve (FC1), and the binding kinetics was evaluated as described before (Gopinath et al., Citation2006, Citation2012b).
3 Results and discussion
Aptamers are potential artificially selected molecules, so-called ‘artificial antibodies’, widely accepted to be substituted for conventional antibodies. Aptamers have been displayed and demonstrated for a wide range of biomedical applications (Gopinath et al., Citation2014, Citation2012a; You et al., Citation2011). Further, aptamers generated with special focus on viruses can be used as anti-viral compound, as it can be a drug-lead. To apply aptamer as a drug, the primary criterion is to design the shorter version, to facilitate the easier usage. Thus, in the present study a strategy (Crawling of base deletion) is proposed to shorten the full-length aptamer, without involving wet laboratory experiments. For the potential application, two anti-viral aptamers were chosen for the demonstration, these are anti-Influenza A/Panama/2007/1999 (H3N2) and anti-gD protein from Herpes Simplex Virus-1 aptamers (Gopinath et al., Citation2006, Citation2012b).
3.1 Crawling the sequences for anti-Influenza A/Panama/2007/1999
3.1.1 Aptamer for Influenza A/Panama
In addition to the advantages of aptamer over antibody, aptamer can also distinguish the closely related molecules compared. It is due to the reason that aptamer binds on the target with a few bases contact. But in the case of antibody, it is larger in size and the major portion of the antibody binds with the target. So that, aptamers are more attractive to be used for the purpose of distinguishing closely related molecules. There are a lot of aptamers that have been generated against a wide range of targets. Gopinath et al. have generated the RNA aptamer against Influenza A/Panama/2007/1999 (H3N2) virus. It is well known that in Influenza-A there are several sub-types that have been emerged with seasonal variations. These subtypes are classified based on Hemagglutinin (HA) and Neuraminidase (NA) proteins, the major proteins on the surface of Influenza viruses (CitationIsin et al., 2002). Antibodies against these proteins cannot differentiate among different Influenza subtypes. CitationGopinath et al. (2006) have generated an aptamer against Influenza A/Panama/2007/1999 (H3N2), which is proved to differentiate the closely related Influenza A viruses. This aptamer (GGGAGAAUUCCGACCAGAAGGGUUAGCAGUCGGCAUGCGGUACAGACAGACCUUUCCUCUCUCCUUCCUCUUCU) was generated using the N30 randomized library. With the addition of both end primers, the length became 74. a shows the secondary structure of the selected aptamer against Influenza A/Panama/2007/1999 (H3N2) and folded by Mfold web server. The selected aptamer binds with the HA, which is confirmed by radioisotope and biacore analyses. It was proved that the selected aptamer fails to bind with HA proteins of other influenza viruses, such as Influenza A/Aichi.
3.1.2 Shortened aptamer by mapping analyses
After generation of anti-influenza A/Panama/2007/1999 (H3N2) aptamer with the length of 30 bases, to obtain the shortened aptamer CitationGopinath et al. (2006) used ethylnitrosourea (ENU)-modification based phosphate interference analysis. For that, they labeled with the radioisotope at the 5′ end of the aptamer and confirmed that real binding sites of the selected aptamer on the intact Influenza virus. It was found that the binding positions of the bases in the aptamer (32, 34, 35, 37 and 39–43) were confirmed to interfere with the HA binding. From that, they found the shortened aptamer sequence as GGGUUAGCAGUCGGCAUGCGGUACAGACAGACCC. b shows the secondary structure of the shortened aptamer by Mfold web server.
3.1.3 Shortening the aptamer using crawling method
To reconfirm the above mapping results, we introduced a crawling method to shorten the aptamer assisted by Mfold web server application. For that, we eliminated the bases gradually from both ends (5′ and 3′) of aptamer. Supplementary Fig. 1a shows the structures of the aptamer after eliminating 2 bases from both sides (5′ and 3′). Generally, the loop region in the secondary structure of aptamer binds with the target rather than the stem region. In the aptamer structure shown in Supplementary Fig. 1a, it has 6 loop regions. Among that, 3 loop regions are close to the forward and reverse primers. Generally, aptamer has less possibility to bind by the bases close to the primer region so that, we focused on other 3 loop regions, which are away from the primer regions. We started to eliminate step-by-step 2, 4, 8, 10, 12, 14, 16, 18, 20 and 22 bases from both 5′ and 3′ ends of the aptamer. As shown in the Supplementary Fig. 1a–k, until we eliminated 20 bases from both 5′ and 3′ ends, the 3 loops maintain their structures. Generally to keep stable loop structure, need support from stem region, so that only 4 bases of the stem are remaining to make these loops. After this point, we eliminated only one base from both ends. As shown in the Supplementary Fig. 1k after we eliminated one base, the stem loses its structure. So, we decided that this is the point to stop crawling and we finalized the shortened aptamer as shown in Supplementary Fig. 1j. The shortened aptamer has 3 proper loop structures and the stable stem.
3.1.4 Confirmation of the shortened aptamer with mapping analyzes
The sequence of our shortened aptamer by Mfold web server application was GGUUAGCAGUCGGCAUGCGGUACAGACAGACCUU. Previously, the shortened aptamer determined against hemagglutinin by mapping analyses was GGGUUAGCAGUCGGAAUGCGGUACAGACAGACCC. When we compared these two sequences as shown in , they completely resemble each other. Structures from both sequences have 3 loop structures with the stable stem. a shows the secondary structure and the energy distribution analyses of the selected aptamer against Influenza A/Panama by Mfold web server. b and c shows the predicted secondary structure of both shortened aptamers (predicted by mapping analyses and crawling method) and energy distribution. These secondary structures are almost same and the energy distribution also shows the same pattern. The dissociation constant (Kd) of both full-length and shorter version of this aptamer against hemagglutinin is similar with the value of ∼188 pM (CitationGopinath et al., 2006).
Figure 2 Secondary structure and the energy distribution analyses with aptamer selected against Influenza A/Panama/2007/1999 (H3N2) (a) Shortened aptamer by mapping analyses. (b) Shortened aptamer by crawling method. Energy distribution patterns obtained from Mfold web server are shown in lower panel.
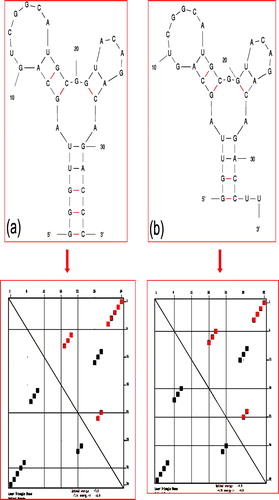
3.2 Crawling the aptamer sequence against gD protein of Herpes Simplex Virus-1
3.2.1 Aptamer against gD protein of Herpes Simplex Virus-1 (gD-HSV-1)
For the second example in the present study, we choose the aptamer generated against gD-HSV-1. gD-HSV-1 is a highly homologous protein, with a sequence identity of about 86% within the ectodomain region. gD protein is required for viral entry to bind the specific cellular receptors. Because of this importance, against this gD protein, CitationGopinath et al. (2012b) have generated the aptamer using SELEX method. The SELEX method started from the nucleic acid library contains the randomized region of 74 bases (N74). The selected aptamer (GGGAGCUCAGCCUUCACUGCACGAGAGAGGUCGUCCCCAGGGGAGAACUCGUGCUCCUGGAGGCAAGUUGACUGCUCGCUCUCCGCUGGUCAAGGGCACCACGGUCGGAUCCUG) has 74 randomized regions. With the addition of both primers, the length becomes 114 bases. a shows the predicted secondary structure of the aptamer generated against gD-HSV-1 by Mfold web server. Since the full-length of the aptamer has 114 bases, it is too long, and it is hard to shorten the aptamer length by routine wet experiments.
3.2.2 Shortening gD-HSV-1aptamer by mapping analyses
To find out the shortened aptamer, CitationGopinath et al. (2012b) used the mapping interference analyses similar to the previous case. It was found that the phosphates between the base positions 29 and 41 were found to interfere strongly with gD binding, suggesting their importance of these bases. In addition, the phosphate regions of base positions 44–53 were also involved in these interactions. From these mapping analyses, they predicted that the shortened aptamer is with 57 bases (GGGCCUUCACUGCACGAGAGAGGUCGUCCCCAGGGGAGAACUCGUGCUCCUGGAGGC). b shows the secondary structure of the shortened aptamer determined by Mfold web server. And also the shortened aptamer binding with gD-HSV-1 was confirmed by radioisotope and biacore analyses (CitationGopinath et al., 2012b).
3.2.3 Shortening the aptamer using crawling method
As stated in previous discussion, CitationGopinath et al. (2012b) used the method of mapping analyses to predict the shortened aptamer. Here, we used the crawling method assisted by Mfold web server to predict the shortened aptamer. As shown in a, the predicted secondary structure has 7 loops. Four loops are very close to the primer regions, and we focused on the remaining 3 loops, which are far from the primers. It is more possible that the binding region is close to these loop regions. As in the previous case, here also we started to eliminate 2 bases from both 5′ and 3′ ends and checked the structural changes. As shown in the Supplementary Fig. 2a–h, after eliminating 16 bases from both sides we couldn’t observe any specific structural changes in the loop region. Then we eliminated 4 bases further from both sides, as shown in the Supplementary Fig. 3a and b and there is no structural change in the loop region. Since it is close to the loop region, from this point, we eliminated one base from both sides. Until we eliminated 25 bases (Supplementary Fig. 3c–f) the structure remained intact with loop regions. After elimination of 26 bases from both sides, the obtained structure is displayed in the Supplementary Fig. 3g, it shows the complete loss of loop region so that, we stopped the elimination at this point and finalized the shortened aptamer.
3.2.4 Confirmation of the shortened aptamer with mapping analyzes
The sequence of our shortened aptamer by Mfold web server has 66 mer (GAGAGGUCGUCCCCAGGGGAGAACUCGUGCUCCUGGAGGCAAGUUGACUGCUCGCUCUCAGCUGGU). The shortened aptamer by mapping analyses was GGGCCUUCACUGCACGAGAGAGGUCGUCCCCAGGGGAGAACUCGUGCUCCUGGAGGC. a shows the secondary structure of the selected aptamer against gD-HSV1 and its energy distribution determined by Mfold web server. b and represents the secondary structure of the shortened aptamer and energy distribution against gD-HSV1 by mapping analyses and Mfold web server. When we compared b and c, these two structures are almost resembles each other. Both structures have 3 loop regions with the stable stem. The secondary structures of these aptamers are almost having the same energy distribution. The dissociation constant (Kd) of both full-length and shorter version of this aptamer are with the values of ∼109 and 32 nM, respectively (CitationGopinath et al., 2012b).
4 Conclusion
Shortening the aptamer sequences is important to design experiments and down-stream applications. Herein, we introduced a crawling method to shorten the aptamer by Mfold web server application. For that, we have chosen two different lengths of the aptamer generated against Influenza A/Panama/2007/1999 (H3N2) and gD protein of Herpes Simplex Virus-1, which have a randomized nucleotide sequences of 30 and 74 bases, respectively. Successfully, we could predict the shorter version of aptamer using crawling method and the predicted aptamers were resembles with the shortened aptamer determined by mapping analyses. The proposed crawling method is useful to shorten the lengthier aptamer sequences. It addition, it is wise to shorten the aptamer by excluding the primer regions for easier analyses; however, it depends on the involvement of primer regions in the formation of active stem and loop portions of the structures.
Conflict of interest
Authors declared that we have no conflict of interest.
Appendix A Supplementary material
Supplementary data associated with this article can be found, in the online version, at http://dx.doi.org/10.1016/j.jaubas.2016.07.001.
Appendix A Supplementary material
Supplementary Material
Download MS Power Point (455.3 KB)Notes
Peer review under responsibility of University of Bahrain.
References
- S.C.B.GopinathAnti-coagulant aptamersThromb. Res.122200883884710.1016/j.thromres.2007.10.022
- S.C.B.GopinathMethods developed for SELEXAnal. Bioanal. Chem.387200717118210.1007/s00216-006-0826-2
- S.C.B.GopinathAntiviral aptamersArch. Virol.15220072137215710.1007/s00705-007-1014-1
- S.C.B.GopinathK.AwazuM.FujimakiWaveguide-mode sensors as aptasensorsSensors1220122136215110.3390/s120202136
- S.C.B.GopinathK.HayashiP.K.R.KumarAptamer that binds to the gD protein of herpes simplex virus 1 and efficiently inhibits viral entryJ. Virol.8620126732674410.1128/JVI.00377-12
- S.C.B.GopinathT.LakshmipriyaK.AwazuColorimetric detection of controlled assembly and disassembly of aptamers on unmodified gold nanoparticlesBiosens. Bioelectron.51201411512310.1016/j.bios.2013.07.037
- S.C.B.GopinathT.S.MisonoK.KawasakiT.MizunoM.ImaiT.OdagiriP.K.R.KumarAn RNA aptamer that distinguishes between closely related human influenza viruses and inhibits haemagglutinin-mediated membrane fusionJ. Gen. Virol.87200647948710.1099/vir.0.81508-0
- S.C.B.GopinathT.S.MisonoP.K.R.KumarProspects of ligand-induced aptamersCrit. Rev. Anal. Chem.382008344710.1080/10408340701804558
- B.IsinP.DorukerI.BaharFunctional motions of influenza virus hemagglutinin: a structure-based analytical approachBiophys. J.82200256958110.1016/S0006-3495(02)75422-2
- T.LakshmipriyaM.FujimakiS.C.B.GopinathK.AwazuGeneration of anti-influenza aptamers using the systematic evolution of ligands by exponential enrichment for sensing applicationsLangmuir292013151071511510.1021/la4027283
- T.LakshmipriyaM.FujimakiS.C.B.GopinathK.AwazuY.HoriguchiY.NagasakiA high-performance waveguide-mode biosensor for detection of factor IX using PEG-based blocking agents to suppress non-specific binding and improve sensitivityAnalyst13820132863287010.1039/c3an00298e
- C.LozuponeS.ChangayilI.MajerfeldM.YarusSelection of the simplest RNA that binds isoleucineRNA920031315132210.1261/rna.5114503
- X.NiM.CastanaresA.MukherjeeS.E.LupoldNucleic acid aptamers: clinical applications and promising new horizonsCurr. Med. Chem.1820114206421410.2174/092986711797189600
- K.SzetoD.R.LatulippeA.OzerJ.M.PaganoB.S.WhiteD.ShallowayJ.T.LisH.G.CraigheadRAPID-SELEX for RNA aptamersPLoS ONE82013e8266710.1371/journal.pone.0082667
- C.YangN.SpinelliS.PerrierE.DefrancqE.PeyrinMacrocyclic host-dye reporter for sensitive sandwich-type fluorescent aptamer sensorAnal. Chem.8720153139314310.1021/acs.analchem.5b00341
- M.YouY.ChenL.PengD.HanB.YinB.YeW.TanEngineering DNA aptamers for novel analytical and biomedical applicationsChem. Sci.22011100310.1039/c0sc00647e