Abstract
This paper describes a simple, rapid, inexpensive method for the preparation of a new biosorbent based on the modification of miswak fibers by NaOH (AT-Miswak-F). The synthesized AT-Miswak-F sorbent was utilized as an efficient sorbent for the extraction and preconcentration of iron group metal ions from various water samples. In this study, it was found that Fe(III), Co(II) and Ni(II) ions were completely extracted (100%) at pH range of 3–7 and flow rate of 0.3–2.0 mL min−1. Also, the sorption capacity of AT-Miswak-F for Fe(III), Co(II) and Ni(II) are 0.54, 0.24 and 0.15 mmol g−1, respectively. Equilibrium was best described by Freundlich isotherm model (R2 = 0.793) and the initial rate constants were 0.077, 0.054 and 0.035 mmol g−1 min−1, respectively. Under the optimized conditions, the method exhibited a detection limit of 1.4, 2.8 and 2.1 ng mL−1 for Fe(III), Co(II) and Ni(II) ions in water samples with relative standard deviations of 2.6% (n = 4). The method was successfully applied for the determination of Fe(III), Co(II) and Ni(II) ions in sea, ground and contaminated water samples.
1 Introduction
Iron has an essential role in life and a range of biochemical reactions in the living organisms. Iron–proteins are found in all living organisms, ranging from the evolutionarily primitive archaea to humans (Kassem and Amin, Citation2013; Pourjavid et al., Citation2014). Cobalt is also beneficial for humans since it is a metallo-cofactor in vitamin B12 (cobalamin); which contributes to the prevention of pernicious anemia and production of red blood cells and has a key role in the normal functioning of the brain and nervous system (Wen et al., Citation2013; Naushad et al., Citation2015a). Nickel is used in many industries such as stainless steel, nickel cast irons, alnico magnets, coinage, rechargeable batteries, electric guitar strings, and plating and as a green tint in glass (CitationGómez-Nieto et al., 2013).
Pollution from chemicals products is a real threat to the aquatic environment, air, and soil (CitationEl Haddad et al., 2012). Heavy metals are unpleasantly affecting our ecosystem due to their toxicological and physiological effects in environment (CitationNaushad and AL-Othman, 2015). These metals, if present beyond certain concentration can be a serious health hazard which can lead to many disorders in normal functioning of human beings and animals (CitationAL-Othman et al., 2012). Determination of Fe, Co and Ni ions in water samples is to some extent problematic because of the high concentration of interfering matrix components. Preconcentration and separation procedures have been proposed to overcome this problem (Naushad et al., Citation2014; Awual et al., Citation2015; Naushad et al., Citation2015b). Methods employing biosorbents are recommended for the preconcentration and separation studies due to their simplicity and environmentally friendliness (CitationHe and Chen, 2014). Moreover, they offer improvements in the sensitivity and selectivity of spectrophotometric methods for the determination of many metal ions (CitationOguz and Ersoy, 2014). The root of salvadora persica tree (miswak) is used as a natural toothbrush (Aboul-Enein, Citation2014; Naeini et al., Citation2014) since it contains fluoride salts, detergents, antiseptics, and enzyme inhibitors (CitationAlili et al., 2014). In previous studies, we used the miswak for the extraction of some organic dyes (Moawed and Abulkibash, Citation2012; Moawed, Citation2013). Though miswak is an excellent sorbent, the leaching of some of its components hinders its application. This problem necessitates the preparation of a chemically stable miswak for extraction purposes. To improve their properties, biosorbents were modified with different reagents including KOH, ZnCl2 and/or C6H12 (AL-Othman et al., Citation2012; Alshehri et al., Citation2014; Nadeem et al., Citation2014).
This paper describes a simple, rapid, inexpensive procedure with available versatile techniques for the preparation of a new biosorbent by the treatment of miswak fibers with an alkali (NaOH) to eliminate the problem of leached compounds. The AT-Miswak-F was characterized by IR and UV/Vis spectroscopy, SEM and elemental analysis. Parameters controlling the sorption of Fe(III), Co(II), and Ni(II) ions onto AT-Miswak-F, including pH, initial concentrations, shaking time and solution temperature were optimized.
2 Experimental
2.1 Instruments
Iron, cobalt and nickel ions were determined using a Shimadzu UV-1800 spectrophotometer (Shimadzu Corporation, Japan). A glass mini column 10 cm long and 1.0 cm in diameter was used for dynamic procedure. pH measurements were carried out by a Jenway pH meter model 3510, (U.K). Conductivity was performed using a Conductivity Meter (Jenway, model 4071, U.K).
2.2 Reagents and materials
Stock solutions of iron(III), cobalt(II) and nickel(II) (1 mg mL−1) were prepared by dissolving appropriate amounts of iron(III) sulfate, cobalt(II) nitrate and nickel(II) nitrate salts (Fluka) in distilled water containing 1 mL of concentrated HNO3 (Adwic, Egypt). Salvadora persica sticks (Miswak) were obtained from a plantation in El-Khtrichia, El-Qtif, Saudi Arabia.
2.3 General procedures
Alkali-treated miswak fibers (AT-Miswak-F) were prepared by blending miswak sticks (Miswak-F) in a food-processing blender, and then Miswak-F powder was soaked in 1 mol L−1 solution of NaOH for 24 h. AT-Miswak-F was filtered off then washed with distilled water, dried at 100 °C and sieved (particle size >250 μm).
A portion of 0.05 g of the AT-Miswak-F was agitated with 25 mL solution of the tested metal ions for 60 min at 25 °C. The solutions were filtrated and the remaining Fe(III), Co(II) and Ni(II) concentrations was measured spectrophotometrically (CitationMarczenko, 1986).
A 25 mL of the tested metal solutions (1 μg mL−1) were passed through the AT-Miswak-F–containing columns at a flow rate of 1 mL min−1. Metal ions were eluted from the AT-Miswak-F columns by 1 mol L−1 HCl. The eluates of metal ions were determined spectrophotometrically.
The tests of addition/recovery of different amounts of iron were performed for the underground water of Dammam city. A 0.1 g portion of the AT-Miswak-F was mixed with 25 mL of water sample which spiked with 20–125 μg of iron(III) then the batch procedure was applied.
To estimate the accuracy of the presented procedure for determination of iron in water; underground water of Nestle SA (Springer Water Factory Co Ltd), sea water of Arabian Gulf in Dammam city have been investigated using batch technique. Collection and determination of iron (III), cobalt (II) and nickel (II) ions in industrial wastewater of from the cooling pond of the casting iron company in Dammam city (spiked with 20 μg of metal ions) using dynamic technique has been investigated.
Density of AT-Miswak-F measurement was carried out in a 25 mL density bottle (pycnometer). The AT-Miswak-F was added to the density bottle with gentle tapping to ensure the settlement of the particles to the bottom; water was then added to the pycnometer in a way to fill all air spaces with water. Chemical composition of AT-Miswak-F, pHZPC, iodine number, density, electrical conductivity, acidic and basic sites were estimated according to procedure described elsewhere (Ververis et al., Citation2007; Moawed, Citation2013; Moawed et al., Citation2014).
3 Results and discussion
3.1 Characteristics of AT-Miswak-F
The densities of Miswak-F and AT-Miswak-F are 0.52 and 0.37 g cm−3, respectively (). AT-Miswak-F (miswak after treatment by NaOH solution) is less dense than the normal one. This is due to hydrolysis of plant pigments, lipids and mineral salts and these fractions were leached out from the Miswak-F. Also, the hydrolysis process is leading to the increase in spaces, channels, and pores.
Table 1 Chemical and physical properties of miswak fibers and alkali treated miswak fibers.
The values of electrical conductivity are 1.74 and 0.19 mS/cm for 1% solution of Miswak-F and AT-Miswak-F, respectively (). This result indicates the chemical stability of the AT-Miswak-F in aqueous solution when compared with that of the Miswak-F.
IR spectra of Miswak-F in KBr pellets were collected. shows the IR main spectral bands of Miswak-F and AT-Miswak-F recorded in the spectral range of 400 and 4000 cm−1. From the obtained results, it is clear that the spectral bands of AT-Miswak-F are shifted to higher frequency compared to those of the Miswak-F. This result may be attributed to the leaching out of some mineral salts in addition to the hydrolysis of some of the miswak matrix into free functional groups.
Table 2 Spectra data of miswak fibers and alkali treated miswak fibers.
C, H, N, S and O percentages of Miswak-F and AT-Miswak-F were estimated. The results show that carbon and hydrogen percentages of the AT-Miswak-F (45.1 and 5.8%) are greater than those of the Miswak-F (34.5 and 4.5%). However, the percentages of nitrogen, sulfur and oxygen decreased due to the leaching out of some mineral salts. The obtained results show that the AT-Miswak-F consists mainly of hydrocarbon compounds e.g. lignin and cellulose.
The Surface morphology of Miswak-F and AT-Miswak-F was investigated using scanning electron microscopy (SEM) at magnifications from 100 to 3000×. The images of Miswak-F and AT-Miswak-F (400–800×) are shown in . The microstructure of the miswak fibers reveals that spaces, channels and pores of miswak fibers increased after alkali treatment. Also, the surface of AT-Miswak-F contains many pores with pore size between 50 μm and 500 μm (), indicating its suitability for metal ions sorption.
Figure 2 Surface morphology of AT-Miswak-F using scanning electron microscopy (SEM) at magnifications 800 and 1600×.
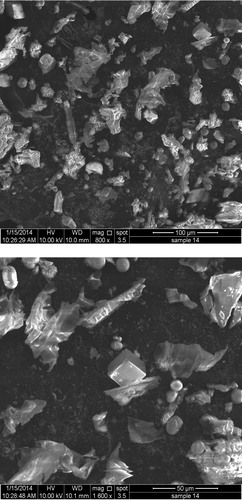
The electronic spectra of Miswak-F and AT-Miswak-F were recorded using Nujol mulls method. The UV spectrum of Miswak-F shows many peaks between 200 and 400 nm (381, 219, 212 and 205 nm, ) attributed to its numerous functional groups. These peaks are slightly shifted (360, 217, 208 and 202 nm) in the AT-Miswak-F spectra (blue shift, ). This may be due to the hydrolysis of the functional groups of the Miswak-F.
The values of pHZPC of both the Miswak-F and AT-Miswak-F are 5.2 and 4.6, respectively, indicating their acidic character (a). The surface of the AT-Miswak-F is positively charged at pH < 4.6; however, the surface sites are negatively charged at pH > 4.6. The positive charge of the surface of the AT-Miswak-F in acidic medium is due to the protonation of oxygen atoms of cellulose and lignin. The negative charge of the surface of the AT-Miswak-F would be attributed to the deprotonation of the phenolic and carboxylic groups. The pHZPC value of the AT-Miswak-F is lower than that of the Miswak-F. This may be attributed to the decrease in the basic functional groups after the treatment with NaOH solution. Due to the buffering effect of water on Miswak-F and AT-Miswak-F surfaces; the pH measurements are only considered in the pH ranges of 4–10.8 and 4.8–7.5, respectively (b).
Figure 3 (a) pHZPC of miswak fiber (Miswak-F) and miswak fibers after alkali treatment (AT-Miswak-F) & (b) effect of initial pH on the Miswak-F and AT-Miswak-F.
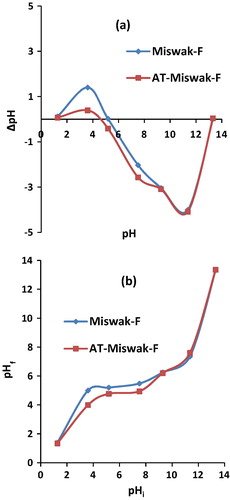
The iodine number gives information about the internal surface of an adsorbent; since it is assumed to measure the surface area in micropores within pore sizes ⩾10 Å (CitationDaifullah et al., 2003). The iodine molecule has an area of 0.15–0.42 nm2, therefore it can fit into the micropores of sorbent particles, providing information about the internal surface (CitationAttia et al., 2006). The iodine number of both Miswak-F and AT-Miswak-F are 3.1 (396.9 mg g−1) and 5.2 mmol g−1 (653.8 mg g−1), respectively (). Based on the value of the iodine number, the AT-Miswak-F exhibits better surface area when compared to activated carbon synthesized from jatropha husk (70 mg g−1) (CitationNamasivayam et al., 2007), rice husk (122 mg g−1) (CitationDaifullah et al., 2003), eucalyptus sawdust (179 mg g−1) (CitationMartins et al., 2007), coir pith (203 mg g−1) (CitationNamasivayam and Sangeetha, 2005), and rubber wood (300 mg g−1) (CitationKumar et al., 2006).
shows the chemical analyses of Miswak-F and AT-Miswak-F. Miswak-F contains cellulose and hemicellulose 59.5%, lignin 31.6% and ash 8.9%. After alkali treatment, the cellulose/hemicellulose and ash percentages decreased to 41.9% and 1.4%, respectively, while the lignin content increased to 56.7% in the AT-Miswak-F. This shows that lignin is the main component of the AT-Miswak-F. It is worth noting that the AT-Miswak-F has a low ash content (2% or less), which makes it suitable to be a good stable biosorbent.
Table 3 Composition of miswak fibers and alkali treated miswak fibers.
The total acidic sites estimated for Miswak-F and AT-Miswak-F are 1.4 and 3.5 mmol g−1, respectively (). The increase in the acidic sites of the AT-Miswak-F is due to the hydrolysis of the Miswak-F matrix to free functional groups. AT-Miswak-F contains 0.75 mmol g−1 of lactonic groups, 0.87 mmol g−1 of carboxylic groups, 1.88 mmol g−1 of phenolic groups. Also, the total basicity was determined to be 0.5 mmol g−1 and as noticed, the acidic sites are greater than the basic sites (3.5 mmol g−1:0.5 mmol g−1 ≈ 7:1).
3.2 Optimum conditions for the extraction of metal ions using miswak fibers
The percentage of the extraction of Fe(III), Co(II) and Ni(II) ions was plotted against the pH value (). The results show that the extraction of Fe(III) and Ni(II) rapidly increased by increasing the pH from 1 to 7 then decreased at pH 9. The maximum extraction efficiency reached 100% for Fe(III) and 78.4% for Ni(II) ions at pH 7, while, the maximum extraction of cobalt (100% Co) was at pH 3. Fe3+ and Ni2+ are sorbed due to the electrostatic interaction between metal ions (cation) and the negatively charged surface of the AT-Miswak-F. Based on the behavior of Co(II) ions extraction onto the AT-Miswak-F, it can be speculated that chelation may be the principal mechanism, since chelation process requires a proper acidity. The selectivity sequence at the pH range of 1–3 is in the order Co(II) > Fe(III) > Ni(II), while the order is Fe(III) > Co(II) > Ni(II) at pH 7. The separation factors (α) of Co(II)/Fe(III) and Co(II)/Ni(II) are 3.8 and 4.0, respectively at pH 1. The results show that controlling the pH of the solution enhances the selectivity of AT-Miswak-F toward the investigated metal ions.
Figure 4 Effect of initial pH on the sorption of iron (III), cobalt (II) and nickel (II) onto AT-Miswak-F.
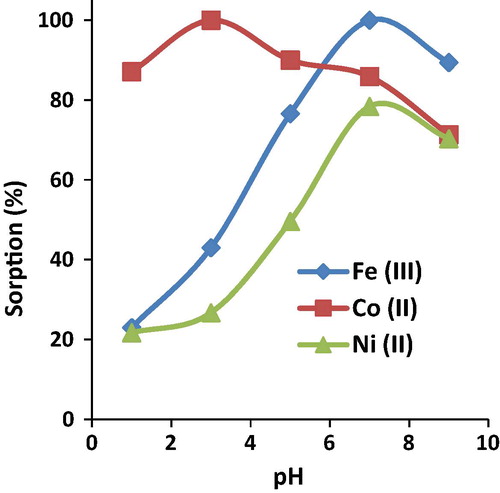
shows the plot of the amount of sorbed metal per unit mass of AT-Miswak-F versus the initial concentrations of Fe(III), Co(II) and Ni(II) ions. The results show that the plots are linear (average value of intercept 0.003) with good correlation (average value of R2 = 0.995). The capacity values of miswak fibers for iron(III), cobalt(II) and nickel(II) ions were estimated to be 0.54, 0.24 and 0.15 mmol g−1, respectively. The sequence of capacity of miswak fibers for these metal ions is in the order, Fe3+ > Co2+ > Ni2+, which may be ascribed to their different ionic sizes and the reaction between AT-Miswak-F functional groups and metal ions. Also, the AT-Miswak-F exhibits better capacity toward Fe(III) (30.2 mg g−1), Co(II) (14.1 mg g−1) and Ni(II) (8.8 mg g−1) in comparison to other sorbents e.g. SiO2/Nb2O5/ZnO mixed oxide by sol–gel: 0.5 mg Co g−1 (CitationDiniz et al., 2014), activated carbon: 11.1 mg Co g−1 and 7.8 mg Ni g−1 (CitationDobrowolski and Otto, 2012), Nile blue–grafted polyurethane foam: 15.0 mg Fe g−1 (CitationEl-Shahat et al., 2003), polyhydroxyl polyurethane foam: 0.5 mg Fe g−1 (CitationMoawed et al., 2013), chromosorb 108: 4.7 Co mg g−1 and 4.9 mg Ni g−1 (CitationTuzen et al., 2005), chitosan polymers: 3.4 mg Co g−1 and 3.1 mg Ni g−1 (CitationEmara et al., 2011), zeolite: 2.0 mg Ni g−1 (CitationAlvarez-Ayuso et al., 2003) and vermiculite: 0.2 mg Ni g−1 (CitationBlais et al., 2003).
Figure 5 Effect of initial platinum concentration on the extraction of iron (III), cobalt (II) and nickel (II) onto AT-Miswak-F.
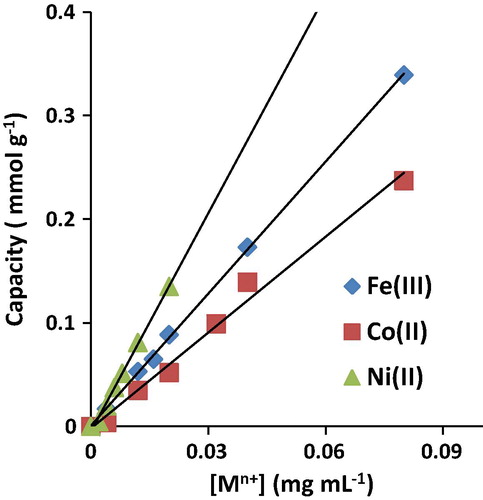
Limits of detection (LOD = 3 σ) and quantification (LOQ = 10 σ) for iron(III), cobalt(II) and nickel(II) ions were calculated. The values of LOD of Fe(III), Co(II) and Ni(III) are 1.4, 2.4 and 2.1 ng mL−1, respectively, while the LOQ values of Fe(III), Co(II) and Ni(II) are 4.7, 8.0 and 7.1 ng mL−1, respectively. The miswak fiber method is more efficient when compared to other methods such as Fe: 2–18 ng mL−1 (Merli et al., Citation2012; Du et al., Citation2013; Kassem and Amin, Citation2013), Co: 2.4–6 ng mL−1 (Rezaei et al., Citation2001; Amin, Citation2014; Zeng et al., Citation2012; Xu et al., Citation2013) and Ni: 1.7–13 ng mL−1 (de Sousa and Korn, Citation2001; Rezaei et al., Citation2001; Amin and AL-Attas, Citation2012; Xu et al., Citation2013). The average recovery percentage values are 98.4% Fe(III), 100% Co(II) and 76.0% Ni(II) with low relative standard deviation values (RSD = 2.0, 2.3 and 3.7%, respectively, n = 4), which indicates both good precision and accuracy of the proposed method for the determination of iron, cobalt and nickel.
The effect of temperature (25–90 °C) on the extraction of iron, cobalt and nickel onto miswak fibers was studied. The maximum extraction of Fe(III) and Co(II) ions (99–100%) was observed at low temperature, while the sorption of Ni(II) ions increased by increasing the temperature. The sorption capacities of AT-Miswak-F for Fe(III) and Co(II) decreased by increasing the temperature, while the capacity for Ni(II) increased. This shows that this method is more efficient for the sorption of nickel disposed from hot wastewater–industries. Also, this reveals that the chemisorption of both iron(III) and cobalt(II) is exothermic while it is endothermic for nickel(II).
The effect of flow rate of Fe(III), Co(II) and Ni(II) solutions (0.3–4.0 mL min−1) through the miswak fibers column was studied. The maximum recovery was observed in the range 0.3–2.0 mL min–1 and the recovery percentage decreased from 100% to 97.8% with increasing flow rate from 2 to 3.5 mL min–1.
The effect of various eluting agents on the stripping of iron from miswak column was tested. It was observed that Fe(III), Co(II) and Ni(II) ions were completely eluted by HCl solution. The effect of different concentrations of HCl (0.05–1.0 mol L−1) on the desorption of the retained iron(III), cobalt(II) and nickel(II) ions on the miswak fibers–columns was studied. A concentration of 0.5 mol L−1 (25 mL) was found to be the minimum concentration of HCl necessary for the complete elution of Fe(III), Co(II) and Ni(II).
The effect of foreign ions (200 μg) on the determination of iron, cobalt and nickel ions (5 μg) was studied. Al3+, Cr3+, Fe2+, Cd2+, Mo6+ and Ag+ ions were found to reduce the sorption of iron ions from 8.6% to 23.4%, while Cu2+ and Bi3+ caused the greatest interference 59.2 and 51.3%, respectively (). Al3+, Cr3+, Ag+, Bi3+ and Cd2+ ions slightly interfered (3.8–18.2%) in the determination of Co2+, while Cu2+ and Fe2+ ions strongly interfered, 47.9 and 75.6%, respectively. Al3+, Mo6+, Cd2+, Fe2+, Cr3+, Bi3+ and Ag+ ions have a slight interference (2.9–18.3%) in the determination of Ni2+, while Cu2+ has the strongest interference 92.7%. The addition of phosphate and citrate anions alongside with adjusting the pH of the solution minimizes the interfering effect.
3.3 Sorption mechanism
The electronic spectral data of the metal: miswak complexes are listed in . In the region between 200 and 800 nm, the absorption bands of the free AT-Miswak-F fibers appeared at 202, 208, 217 and 360 nm. The higher energy bands at 202, 208, 217 nm are assigned to the intraligand transitions (π–π∗) that are localized on the conjugated system and are largely unaffected by complexation (CitationShebl et al., 2014). The lower energy band at 360 nm are assigned to n–π∗ transition of the carbonyl (CO) chromophores that overlapped with the charge-transfer (CT) transitions within the molecule. The UV–Vis spectra of the Fe(III), Co(II) and Ni(II) complexes also exhibited similar transition bands but were shifted to lower wavelengths for all of the metal complexes. The results showed that the spectral bands of miswak functional groups are blue shifted, which may be due to the chelation with the metal ions. The electronic spectra of metal: miswak complexes exhibited new bands at 373 and 384 nm for iron(III) miswak complex, 364 and 394 nm for cobalt(II) miswak complex and 375 and 390 nm for nickel(II) miswak complex. These new bands may be assigned to the intermolecular ligand–metal charge transfer transition LMCT (CitationEl-ghamry et al., 2013).
Table 4 Conductivity and electronic spectral data and their assignments for Miswak-F, AT-Miswak-F and metal: AT-Miswak-F complexes.
The electronic spectra of metal miswak complexes showed two bands in the visible region at 444 and 643 nm for iron(III) miswak complex, 422 and 646 nm for cobalt(II) miswak complex and 448 and 647 nm for nickel(II) miswak complex. For iron(III) miswak complex, the first band at 444 nm is assigned to a charge transfer band (MLCT) and the second band at 643 nm is a d–d transition which can be assigned to 6A1 → 4T2 electronic transition and provides an evidence for octahedral arrangement around the iron(III) ion (CitationIsmail et al., 2012). For cobalt (II) miswak complex, the two bands at 422 and 646 nm can be assigned to 4T1g(F) → 4T1g(P) and 4T1g(F) → 4A2g(F) transitions, respectively, and indicates that the geometry around cobalt(II) ion is octahedral (CitationEmara et al., 2011). For nickel (II) miswak complex, the two bands at 448 and 647 nm can be assigned to 3A2g(F) → 3T1g(P) and 3A2g(F) → 3T1g(F) transitions, respectively, suggesting an octahedral geometry around nickel(II) ion.
The conductivities of the metal miswak complexes in aqueous solutions (1%) were measured at room temperature and the results are listed in . The observed conductivity values for iron (III), cobalt (II) and nickel (II) miswak complexes are 0.34, 0.24 and 0.15 mS/cm respectively, suggesting the non-electrolytic nature of all complexes. The neutrality of these complexes may be attributed to either partial de-protonation of the phenolic (OH) or carboxylic (OCOH) groups of the free AT-Miswak-F during the complexation with the metal ions, and/or coordination with the anions (SO42− or NO3− ions) of the metal salts.
The percentages of carbon, hydrogen, nitrogen, sulfur and oxygen elements of the free AT-Miswak-F (45.1%, 5.8%, 0.9%, 1.2% and 47.0%, respectively) changed after complexation with iron(III) (40.5%, 5.5%, 1.2%, 3.0% and 49.8%, respectively), cobalt(II), (41.7%, 5.7%, 4.5%, 0.6%, 47.5%, respectively) and for nickel(II) (44.5%, 6.0%, 5.9%, 0.4% and 43.2%, respectively). The decrease in the carbon and hydrogen contents of the corresponding AT-Miswak-F–metal complexes indicates the bonding between AT-Miswak-F fibers and the metal ions (Fe3+, Co2+ and Ni2+) and provides an evidence for complex formation. The decrease in the carbon and hydrogen contents of AT-Miswak-F fibers after complexation with the metal ions is arranged as follows: iron(III) miswak complex > cobalt(II) miswak complex > nickel(II) miswak complex which agrees with the sorption capacity results of miswak fibers: 0.34, 0.24 and 0.15 mmol g−1 for the sorption of Fe(III), Co(II) and Ni(II) ions, respectively. The increase in the sulfur and oxygen contents of the iron(III) AT-Miswak-F complex (3.0 and 49.8%, respectively) compared with those of the free AT-Miswak-F (1.2 and 47.0%, respectively) may be due to the coordination of sulfate groups with iron(III) ions. Also, the increase in the nitrogen content of cobalt(II) (5.9%) and nickel(II) (4.5%) AT-Miswak-F complexes when compared with that of the free AT-Miswak-F (0.9 %) may be attributed to the coordination of the nitrate groups with cobalt(II) and nickel(II) ions. This supports the postulation of the complex formation between AT-Miswak-F and the metal ions (Fe3+, Co2+ and Ni2+), as indicated by low conductivity values of the AT-Miswak-F-metal complexes (0.15–0.34 mS/cm).
The stretching vibrations of carbonyl υ(CO) and phenolic υ(OH), υ(CO) groups of the AT-Miswak-F-metal complexes were noticed to be shifted when compared to those of the free AT-Miswak-F (). The υ(OH) of the AT-Miswak-F at 3387.35 cm−1 was shifted to lower frequencies in the metal miswak complexes (3383.5, 3349.89 and 3358.43 cm−1). This indicates chelation of the phenolic OH group to the metal ions via oxygen atoms. The υ(CO) of the metal miswak complexes was shifted to lower wavenumber (1710.55, 1708.62 and 1712.48 cm−1) compared to that of the AT-Miswak-F at 1717.30 cm−1, indicating the coordination of the CO groups of the AT-Miswak-F with the investigated metal ions. The υ(CO) of the phenolic groups of the AT-Miswak-F at 1333.54 and 1105.01 cm−1 were shifted to lower frequencies after metal sorption (1328.71 and 1101.16, 1324.86 and 1096.33, 1326.79 and 1103.08 cm−1) suggesting its participation in the chelation. The appearance of extra bands at 435.83, 434.87 and 438.73 for Fe(III), Co(II) and Ni(II) complexes, respectively, could be assigned to the stretching frequencies of the υ(MO) bands.
From the results of the electronic and IR spectra of the AT-Miswak-F before and after metal sorption, we suggest that the sorption of iron (III), cobalt (II) and nickel (II) ions on AT-Miswak-F occurs principally according to chelation mechanism via the coordination of phenolic (OH) and carboxylic (OCOH) groups with the metal ions.
The data of isotherm curves were analyzed using Langmuir [Ce/Qc = (1/KLb) + (Ce/KL)] and Freundlich () models, where Ce is the amount of metal sorbed at equilibrium, and KF, n, KL and b are constants. The results show that the correlation coefficient values for the sorption of Fe (III), Co (II) and Ni (II) are 0.683, 0.872 and 0.825, respectively. The plot of Qc/Ce versus Ce for the data according to Langmuir model gives bad linear relationship (R2 = 0.097, 0.206 and 0.142, respectively) for the sorption of iron, cobalt and nickel onto AT-Miswak-F. This leads to conclude that the surface of the AT-Miswak-F has a heterogeneous structure and the value of n > 1 shows the favorable nature of adsorption (CitationNaushad and AL-Othman, 2015).
The diffusion mechanism of the sorption of Fe(III), Co(II) and Ni(II) onto AT-Miswak-F was investigated using Morris-Weber [] and Reichenberg [Bt = −0.4977 – ln(1 − F)] & [F = (6/R)(Dit/π)1/2] equations, where Qe and Qt are the amount of metal ion sorbed at equilibrium and time t, respectively. ki is the intraparticle diffusion rate constant (mmol g−1 min−1/2). The Bt value is a mathematical function of
. Di is the effective diffusion coefficient. The obtained data show that the plots of Q versus
are linear and the values of correlation coefficients are 0.996, 0.931 and 0.77, respectively. The diffusion rate constant values of sorption of Fe(III), Co(II), Ni(II) are 8.7, 6.1 and 0.7 μmol g−1 min−1/2, respectively. According to the forgoing results, the diffusion rate is rapid. The sequence of diffusion rate was in the order, Fe(III) > Co(II) > Ni(II) which is attributed to their different ionic sizes and nature of the reaction of AT-Miswak-F functional groups with these metal ions. The relation between Bt and t for the sorption of Fe(III), Co(II) and Ni(II) was investigated (average value of R2 = 0.845). This reflects the formation of partial film along with the intraparticle diffusion. The linear plots of F versus t1/2 give the numerical values of effective diffusion coefficient (Di) from the slope. The values of Di of the Fe(III), Co(II) and Ni(II) sorption are 9.6 × 10−8, 9.3 × 10−8 and 0.09 × 10−8 cm2 min−1, respectively. The film diffusion rate depends on the size of metal ions.
3.4 Analytical applications
Results given in show that the removal of iron ions (>95%) from water samples by At-Miswak-F were quantitative. There is a good agreement between added and recovered tested metal ions using batch procedure. The average value of standard deviation for the spiked samples is 2.25%.
Table 5 Recovery of iron (III) from underground water using AT-Miswak fibers.
The obtained results are given in . There is a good agreement between the certified analyses and the direct spectrophotometric determination of iron content with batch procedure. This result shows that iron can be determined effectively in sea water and underground water using AT-Miswak-F method. The relative standard deviation values for the water samples are in the average of 1.15% (n = 3). The obtained data conferred susceptible accuracy of the developed method based on the satisfactory values of RSD% in addition to the reasonably high sensitivity and validity of the proposed method for the determination of iron in different water samples.
Table 6 Determination of iron in certified water samples using batch technique.
The average removal and recovery percentages of metal ions from the wastewater samples are 94–103% (). The average RSD% values are found to be in the range 0.0–1.7%, which is considered relevant for real samples. The results indicate the suitability of the AT-Miswak-F column for water analysis and the removal of the metal ions from industrial wastewater.
Table 7 Determination of metal ions in wastewater samples using dynamic technique.
4 Conclusion
It is of great interest to employ agricultural by-products such as Miswak as an economical method for the treatment of wastewater containing metal ions. Our results demonstrate that miswak is an effective adsorbent for the removal of Fe(III), Co(II) and Ni(II) from aqueous solutions with high sensitivity and selectivity. The treatment of miswak fibers by NaOH solution led to the widening of the pore spaces resulting in the increase in the internal surface area of the miswak fibers. The modified sorbent has better capacity toward Fe (30.2 mg g−1), Co (14.1 mg g−1) and Ni (8.8 mg g−1) than other sorbents. The maximum extraction of Fe(III) and Ni(II) ions was at pH 7, while the maximum extraction of cobalt was at pH 3. Kinetics and isotherm studies have confirmed the fast and intensive sorption of the tested metal ions onto miswak fibers. The faster adsorption kinetic data may be useful for environmental technologies in designing heavy metal removal from wastewater plants. The developed procedure is simple, rapid, inexpensive, with high tolerance to interfering ions. The sorption of iron(III), cobalt(II) and nickel(II) ions on miswak fibers occurs principally according to chelation mechanism, ion association and/or anion exchange. The applicability of the AT-miswak-F was evaluated by the analysis of several real samples. The results indicate that iron group can be determined effectively in seawater and underground water using miswak fiber method. Also, the sorbent is suitable for the removal of metal ions from industrial wastewater. Analytical features such as detection limit, enrichment factor and precision are satisfactory for trace analysis of iron, cobalt and nickel.
Notes
Peer review under responsibility of University of Bahrain.
References
- B.H.Aboul-EneinThe miswak (Salvadora persica L.) chewing stick: cultural implications in oral health promotionSaudi J. Dent. Res.52014913
- N.AliliJ.C.TürpE.M.KulikT.WaltimoVolatile compounds of Salvadora persica inhibit the growth of oral Candida speciesArch. Oral Biol.592014441447
- Z.A.AL-OthmanR.AliMuNaushadHexavalent chromium removal from aqueous medium by activated carbon prepared from peanut shell: adsorption kinetics, equilibrium and thermodynamic studiesChem. Eng. J.1842012238247
- S.M.AlshehriMu.NaushadT.AhamadZ.A.AL-OthmanA.AldalbahiSynthesis, characterization of curcumin based ecofriendly antimicrobial bio-adsorbent for the removal of phenol from aqueous mediumChem. Eng. J.2542014181189
- E.Alvarez-AyusoQuerol X.Garcia-SanchezPurification of metal electroplating waste waters using zeolitesWater Res.37200348554862
- A.S.AminStudy on the solid phase extraction and spectrophotometric determination of cobalt with 5-(2-benzothiazolylazo)-8-hydroxyquinoleneArabian J. Chem.72014715721
- A.S.AminA.S.AL-AttasStudy of the solid phase extraction and spectrophotometric determination of nickel using 5-(4′-chlorophenylazo)-6-hydroxypyrimidine-2,4-dione in environmental samplesJ. Saudi Chem. Soc.162012451459
- A.A.AttiaW.E.RashwanS.A.KhedrCapacity of activated carbon in the removal of acid dyes subsequent to its thermal treatmentDyes Pigm.692006128136
- Md.R.AwualG.E.EldesokyT.YaitaMu.NaushadH.ShiwakuZ.A.AL-OthmanS.SuzukiSchiff based ligand containing nano-composite adsorbent for optical copper(II) ions removal from aqueous solutionsChem. Eng. J.2792015639647
- J.F.BlaisS.ShenN.MeunierR.D.TyagiComparison of natural adsorbents for metal removal from acidic effluentEnviron. Technol.242003205215
- A.A.M.DaifullahB.S.GirgisH.M.H.GadUtilization of agro residues (rice husk) in small waste water treatment plansMater. Lett.57200317231731
- C.S.de SousaM.KornEffects of ultrasonic irradiation on the spectrophotometric determination of nickel with dimethylglyoximeAnal. Chim. Acta4442001309315
- K.M.DinizF.A.GorlaE.S.RibeiroM.B.O.do NascimentoR.J.CorrêaC.R.T.TarleyM.G.SegatelliPreparation of SiO2/Nb2O5/ZnO mixed oxide by sol–gel method and its application for adsorption studies and on-line preconcentration of cobalt ions from aqueous mediumChem. Eng. J.2392014233241
- R.DobrowolskiM.OttoDetermination of nickel and cobalt in reference plant materials by carbon slurry sampling GFAAS technique after their simultaneous preconcentration onto modified activated carbonJ. Food Compos. Anal.2620125865
- Y.DuM.ChenY.ZhangF.LuoC.HeM.LiX.ChenDetermination of iron(III) based on the fluorescence quenching of rhodamine B derivativeTalanta1062013261265
- M.El HaddadR.MamouniN.SaffajSaidLazarRemoval of a cationic dye – Basic Red 12 – from aqueous solution by adsorption onto animal bone mealJ. Assoc. Arab Univ. Basic Appl. Sci.1220124854
- M.A.El-ghamryA.A.SalehS.M.E.KhalilA.A.MohammedMono, bi- and trinuclear metal complexes derived from new benzene-1,4-bis(3-pyridin-2-ylurea) ligand. Spectral, magnetic, thermal and 3D molecular modeling studiesSpectrochim. Acta Part A1102013205216
- M.F.El-ShahatE.A.MoawedM.A.A.ZaidPreconcentration and separation of iron, zinc, cadmium and mercury, from waste water using Nile blue a grafted polyurethane foamTalanta592003851866
- A.A.A.EmaraM.A.TawabM.A.El-ghamryM.Z.ElsabeeMetal uptake by chitosan derivatives and structure studies of the polymer metal complexesCarbohydr. Polym.832011192202
- B.Gómez-NietoM.J.GismeranM.T.SevillaJ.R.ProcopioSimultaneous and direct determination of iron and nickel in biological solid samples by high-resolution continuum source graphite furnace atomic absorption spectrometryTalanta1162013860865
- J.HeJ.P.ChenA comprehensive review on biosorption of heavy metals by algal biomass: materials, performances, chemistry, and modeling simulation toolsBioresour. Technol.16020146778
- T.M.A.IsmailA.A.SalehM.A.El GhamryTetra- and hexadentate Schiff base ligands and their Ni(II), Cu(II) and Zn(II) complexes. Synthesis, spectral, magnetic and thermal studiesSpectrochim. Acta Part A862012276288
- M.A.KassemA.S.AminSpectrophotometric determination of iron in environmental and food samples using solid phase extractionFood Chem.141201319411946
- B.G.P.KumarK.ShivakamyL.R.MirandaM.VelanPreparation of steam activated carbon from rubber wood sawdust (Hevea brasiliensis) and its adsorption kineticsJ. Hazard. Mater.1362006922929
- Z.MarczenkoSpectrophotometric Determination of Elementsthird ed.1986Ellis HorwoodChichester
- A.F.MartinsA.L.CardosoJ.A.StahlJ.DinizLow temperature conversion of rice husks, eucalyptus sawdust and peach stones for the production of carbon like adsorbentBioresour. Technol.98200710951100
- D.MerliA.ProfumoC.DossiAn analytical method for Fe(II) and Fe(III) determination in pharmaceutical grade iron sucrose complex and sodium ferric gluconate complexJ. Pharm. Anal.22012450453
- E.A.MoawedEffect of heating processes on Salvadora persica (Miswak) and its application for removal and determination of aniline blue from wastewaterJ. Taibah Univ. Sci.720132634
- E.A.MoawedA.B.AbulkibashSelective separation of Light green and Safranin O from aqueous solution using Salvadora persica (Miswak) powder as a new biosorbentJ. Saudi Chem. Soc.201218 http: //dx.doi.org/10.1016/ j.jscs. 2012.10.011
- E.A.MoawedN.BurhamM.F.El-ShahatSeparation and determination of iron and manganese in water using polyhydroxyl polyurethane foamJ. Assoc. Arab Univ. Basic Appl. Sci.1420136066
- E.A.MoawedI.IshaqA.Abdul-RahmanM.F.El-ShahatSynthesis, characterization of carbon polyurethane powder and its application for separation and spectrophotometric determination of platinum in pharmaceutical and ore samplesTalanta1212014113121
- R.NadeemM.N.ZafarA.AfzalM.A.HanifR.SaeedPotential of NaOH pretreated Mangifera indica waste biomass for the mitigation of Ni(II) and Co(II) from aqueous solutionsJ. Taiwan Inst. Chem. Eng.452014967972
- A.NaeiniN.J.NaderiH.ShokrAnalysis and in vitro anti- Candida antifungal activity of Cuminum cyminumand Salvadora persica herbs extracts against pathogenic Candida strainsJ. Med. Mycol.2420141318
- C.NamasivayamD.SangeethaKinetic studies of adsorption of thiocyanate onto ZnCl2 activated carbon from coir pith, an agricultural solid wasteChemosphere60200516161623
- C.NamasivayamD.SangeethaR.GunasekaranRemoval of anions, heavy metals, organics and dyes from water by adsorption onto a new activated carbon from jatropha husk an agro-industrial solid wasteTrans. IChemE Part B: Process Saf. Environ. Prot.852007181184
- Mu.NaushadM.A.KhanZ.AAL-OthmanM.R.KhanAdsorptive removal of nitrate from synthetic and commercially available bottled water samples using De-Acidite FF-IP resinJ. Ind. Eng. Chem.20201434003407
- Mu.NaushadZ.A.AL-OthmanSeparation of toxic Pb2+ metal from aqueous solution using strongly acidic cation-exchange resin: analytical applications for the removal of metal ions from pharmaceutical formulationDesalin. Water Treat.53201521582166
- Mu.NaushadA.MittalM.RathoreV.GuptaIon-exchange kinetic studies for Cd(II), Co(II), Cu(II), and Pb(II) metal ions over a composite cation exchangerDesalin. Water Treat.54201528832890
- Mu.NaushadZ.A.AL-OthmanMd.R.AwualM.M.AlamAdsorption kinetics, isotherms, and thermodynamic studies for the adsorption of Pb2+ and Hg2+ metal ions from aqueous medium using Ti(IV) iodovanadate cation exchangerIonics21201522372245
- E.OguzM.ErsoyBiosorption of cobalt(II) with sun flower biomass from aqueous solutions in a fixed bed column and neural networks modelingEcotoxicol. Environ. Saf.9920145460
- M.R.PourjavidA.A.SehatM.ArabiehS.R.YousefiM.H.HosseiniM.RezaeeColumn solid phase extraction and flame atomic absorption spectrometric determination of manganese(II) and iron(III) ions in water, food and biological samples using 3-(1-methyl-1H-pyrrol-2-yl)-1H-pyrazole-5-carboxylic acid on synthesized graphene oxideMater. Sci. Eng. C352014370378
- B.RezaeiA.A.EnsafiF.ShandizSimultaneous determination of cobalt and nickel by spectrophotometric method and artificial neural networkMicrochem. J.7020013540
- M.SheblM.A.El-ghamryS.M.E.KhalilM.A.A.KishkMono- and binuclear copper(II) complexes of new hydrazone ligands derived from 4,6-diacetylresorcinol: synthesis, spectral studies and antimicrobial activitySpectrochim. Acta Part A1262014232241
- M.TuzenM.SoylakL.ElciMulti-element pre-concentration of heavy metal ions by solid phase extraction on Chromosorb 108Anal. Chim. Acta5482005101108
- C.VerverisK.GeorghiouD.DanielidisD.G.HatzinikolaouP.SantasR.SantasV.CorletiCellulose, hemicelluloses, lignin and ash content of some organic materials and their suitability for use as paper pulp supplementsBioresour. Technol.982007296301
- X.WenL.HeC.ShiQ.DengJ.WangX.ZhaoApplication of rapid cloud point extraction method for trace cobalt analysis coupled with spectrophotometric determinationSpectrochim. Acta Part A1152013452456
- H.XuW.ZhangX.ZhangJ.WangJ.WangSimultaneous preconcentration of cobalt, nickel and copper in water samples by cloud point extraction method and their determination by flame atomic absorption spectrometryProcedia Environ. Sci.182013258263
- C.ZengY.JiaY.LeeX.HouL.WuUltrasensitive determination of cobalt and nickel by atomic fluorescence spectrometry using APDC enhanced chemical vapor generationMicrochem. J.10420123337