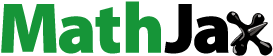
Abstract
In this report, we investigate the electrochemical capacitance and redox behaviour of a stand-alone polyaniline–poly(vinyl alcohol) composite film cast on an insulating substrate (normal printer paper) and compared it with an electrochemically synthesized composite film deposited on a gold electrode. The stand-alone composite film showed timescale-dependent, wide range charging–discharging redox cycling and high electrochemical capacitance in potentiodynamic electrochemical experiments carried out in 1 M HCl solution.
1 Introduction
Conducting polymers can replace metals with the added advantage of being cost-effective, light and easy to process. From the technological standpoint, electroactive polymer films have a variety of important applications, including in electrocatalysis [Citation1,Citation2], energy storage and conversion [Citation1,Citation3–Citation5], smart windows and optical displays [Citation1,Citation6–Citation8], (bio)chemical sensors [Citation9–Citation11], nanoactuators and artificial muscles [Citation12,Citation13]. Most conducting polymers are based on the widely available monomers of aniline, thiophene, pyrrole and their derivatives [Citation14–Citation20] because of their good response rates during redox switching in potential cycling experiments [Citation21].
Polyaniline (PAN) is a particularly interesting conducting polymer, with unique, attractive attributes. It can be easily prepared by both chemical and electrochemical routes, has multiple accessible redox states and is chemically, thermally and environmentally stable in air and aqueous media [Citation22,Citation23]. Nevertheless, it is not easy to process because of its brittleness and insolubility in most common solvents, obviating its use in many technologically important applications. Both the mechanical and the redox behaviour of PAN can be significantly improved by adopting strategies such as blending or compositing with conventional polymers [Citation22,Citation24–Citation28]; introducing substituent functional groups [Citation29,Citation30]; polymer intercalation with carbon nanotubes [Citation31], grapheme [Citation32], metal–metal oxide nanowires or nanoparticles [Citation10,Citation33]; and copolymerization [Citation34].
Poly(vinyl alcohol) (PVA) is widely available, water-soluble, has robust mechanical properties and is easy to process and could therefore be used to overcome the generic problem of the brittleness of PAN. PAN–PVA composites have been synthesized by various methods. In many studies, the properties of the composite films were shown to depend on the initial concentration of complexing agent (here, PVA) and the route of PAN preparation (chemical or electrochemical) [Citation22,Citation27,Citation28,Citation35]. Recently, we reported a correlation between the structural stiffening, redox amplification and ion–solvent compositional dynamics of electrochemically prepared PAN–PVA composite films [Citation36]. Addition of PVA to the PAN matrix significantly increased the polymer’s redox behaviour and noticeably improved the mechanical properties of the composite film, manifested through removal of the film solvent content (softening agent).
The aim of this study was to determine the redox behaviour and electrochemical capacitance of a chemically prepared stand-alone PAN–PVA composite film cast on insulating printer paper and to compare its properties with those of an electrochemically prepared, electrode-surface confined composite film. The redox behaviour of films was characterized as a function of potential, charge injection and experimental timescale using potentiodynamic electrochemical methods. In this study, we aim to examine the doping–de-doping processes of ion exchange at the electrode–electrolyte interface during redox switching transformation to resolve ions trapped at deep and shallow levels in both bulk composite films and at the electrode–electrolyte double layer.
2 Experimental
2.1 Materials and solution preparation
Aniline (99%, Panreac), PVA (≥99% hydrolysed, relative molecular mass 89 000–98 000, Aldrich) and HCl (37%, Panreac) were used as received without further purification. For chemical synthesis of PAN–PVA composite film, an aqueous solution of 0.1 M aniline containing 4% (w/w) of PVA was prepared from 1 M HCl electrolyte solution. PVA readily dissolved in water, and a homogeneous solution was obtained by continuous stirring at ∼70 °C for ∼1 h.
2.2 Preparation and characterization of composite films
A stand-alone PAN/PVA composite film was prepared by chemical oxidation by adding 10 ml of a 0.5 M aqueous solution of FeCl3·7H2O to 30 ml of 0.1 M aniline/1 M HCl aqueous solution containing 4% (w/w) PVA. After gentle mixing with a magnetic stirrer for ∼1 h, the composite polymer was formed and then cast on printer paper. A photo of the cast polymer film connected to a simple electrical circuit consisting of wires, a 9-V battery and a LED lamp is shown in Fig. S1 (supplementary material). An electrode of about 1 cm2 was cut and used for electrochemical characterization. The electrochemically prepared composite film was also grown on a gold electrode (area, ∼0.3 cm2) from 0.1 M aniline/1 M HCl solution in a potentiodynamic control experiment using an Autolab instrument PSTAT 128 N (Netherlands) connected to a PC running NOVA 1.7 software in the potential range of −0.2 < E/V < 0.9 and a scan rate of v = 10 mV s−1. The voltammogram of PAN–PVA composite deposition is shown in Fig. S2 (supplementary material). The film thicknesses estimated by coulombic appraisal, assuming a film density of ∼1.2 g cm3, were 5 μm for the stand-alone film and 0.3 μm for the surface-confined film. All measurements were carried out at room temperature. Practically, an as-prepared composite film was casted on normal printer paper (insulating surface) and used as standalone electrode acting as the working electrode of conventional electrochemical cell containing an electrolyte solution of 1 M HCl.
For redox characterization, the composite films were thoroughly rinsed with deionized water and transferred to a monomer-free background electrolyte of 1 M HCl solution. Using an Autolab instrument and the conventional three-electrode electrochemical cell, we subjected the films to potential cycling at different scan rates. A Pt mesh and saturated calomel electrodes were used as counter and reference electrodes, respectively.
3 Results and discussion
3.1 Overview
In this study, we found significant differences in the electrochemical responses of the chemically prepared films used as stand-alone electrodes compared to films synthesized electrochemically and deposited on a highly conducting gold electrode. Oxidative polymerization of the chemical and electrochemical composite films from the growth (deposition) electrolyte solution allowed the doping properties of the counter ions (chlorine in this case) to drastically modify the electrical conductivity of the film. Incorporation or removal of counter ions within the polymer matrix during doping and de-doping is needed to satisfy the electroneutrality of balancing the net charge build-up of films during electrochemical oxidation–reduction conversions, i.e. during redox switching. In the case of PAN-based films, the mechanism of initial charge compensation involves the ingress and egress of hydrogen proton (the only counter cation in this case), where film conductivity is sensitive to both local pH changes and counter-anion exchanges [Citation17], resulting in a complex charge transport mechanism of mixed proton-ionic charge carriers. In addition to the ion exchange required to maintain electroneutrality, other fundamental processes accompanying film redox switching include the exchange of neutral species (water in the case of permoselective films) to satisfy thermodynamic activity balance constraint and redox- and composition-driven film structural changes.
We showed previously that increasing the amount of PVA in the matrix of an electrochemically synthesized PAN film significantly increases film growth and affects the film composition dynamics, in such a way that the rate of ion exchange (charge transfer) is enhanced at the polymer–electrolyte (cf. polymer-electrode) interface [Citation36]. It was also showed that a relatively small PVA content dramatically changed the film solvent composition, reflecting a direct correlation between the intercalation of PVA chains in the contexture of PAN and stiffening of the composite film structure.
3.2 Electrochemical responses
The electrochemical characterization of the cast PAN–PVA composite polymer is compared with the potentiodynamic behaviour of the electrodeposited film in . The potential-dependent processes, i.e. the i–E responses, of the two systems are quite distinct. The redox behaviour of the stand-alone chemically prepared PAN–PVA composite film cast on insulating print-paper differed from that of the electrochemical film deposited on a gold electrode and reflect the differences in physico-chemical properties of the two systems. shows three phenomena. First, the electrochemical window of the stand-alone composite film is much wider, extending to 3 V, than that of the thin electrochemically deposited film, 0.7 V, i.e. more than four times wider, a very important property for energy storage and battery technology.
Fig. 1 The i–E responses of the standalone and the electrochemically PAN/PVA composite films redox-cycled in 1 M HCl electrolyte solution: (a) represents i–E response of the standalone film showing two distinctive current responses assigned to two separate modes of ion exchange as explained in the main text; (b) i–E response of PAN/PVA composite film electrochemically deposited on gold electrode subjected to redox switching in 1 M HCl electrolyte solution.
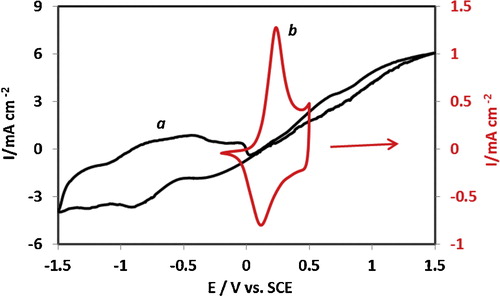
Secondly, differences in the shape of the i–E response and the current density of the two systems can be ascribed to many competing, mixed electrochemical processes, including mixed electron-ionic controlled conductivity and redox-driven composition–structure relations, which may play a crucial role in the distinctive redox response of the stand-alone composite film (curve “a” in ). The effects of the insulating cellulose-based paper on the electrochemical activity of the cast composite film may be a factor in the observed changes in the shape and position of the i–E curve of the stand-alone polymer film electrode. The i–E response (curve “b” in ) of the electrodeposited PAN–PVA composite film shows typical, characteristic redox transformations of surface-immobilized PAN films, as widely reported in the literature [Citation28,Citation35,Citation36].
Thirdly, the i–E response of the stand-alone PAN–PVA electrode exhibits two separate potential-dependent redox responses, at the lower and extreme ends of the oxidation potentials. We suggest that this separation is due to the effects of spatial distribution and the localization of mobile species in the film matrix, where there are two-phase distributions of shallowly trapped ions (in the bulk of the film) and deeply trapped ions (in the double layer near the electrode–film interface), as depicted in . The redox behaviour of the stand-alone film in relation to the deeply trapped ions could be accessed at extreme oxidation potential perturbations and at the extended experimental timescale (slower potential scan rate, here, v = 10 mV s−1). As shown in , at higher potential scan rates (v = 20, 50 and 100 mV s−1), the redox response due to deeply trapped ions disappears, while the current responses of shallowly trapped ions increase with the scan rate.
Scheme 1 Depicts the state of the standalone film in two different modes of mobile species (solvent/ion) entrapment resulting in two distinct electrochemical response behaviours: (a) represents a slightly oxidized state of the film where ions are shallowly trapped at electrolyte/polymer interface; (b) shows highly oxidized state of the film at extreme oxidation potential during redox switching where ions are deeply trapped at electrode/polymer double layer.
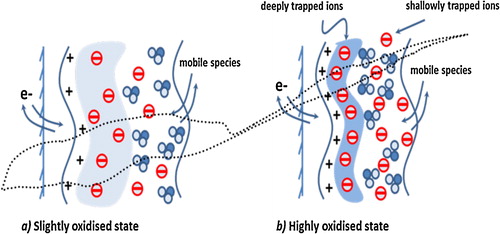
3.3 Energy storage and electrochemical capacitance of the PAN–PVA stand-alone electrode
illustrates the charge–discharge cycle of PAN–PVA composite films, where the charge profile of the stand-alone composite film was compared with that of the film electrodeposited on a gold electrode surface. Although the window of the charge–discharge cycle of the stand-alone composite film shows a wide response, the shape of the Q-E response is capacitive; the charge profile of the surface-confined film shows a typical response of redox-controlled charge conversions.
Fig. 3 Coulombic appraisal of composite films: (a) charging–discharging cycle of the standalone film exhibiting capacitive behaviour; (b) charging–discharging cycle of the electrochemically prepared composite film showing typical redox conversion response of PAN films. The potential scan rate for the cycles shown is v = 10 mV s−1.
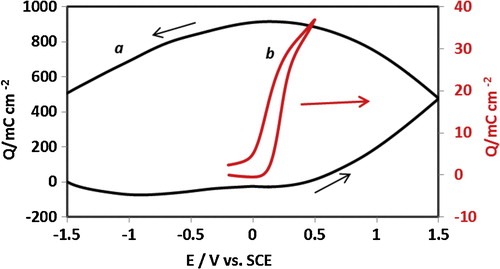
The electrochemical behaviour of the electrode was stable at successive cycles at all scan rates used in all the experiments performed. Nonetheless, the charge–discharge capacity of the stand-alone film dropped dramatically with increasing potential sweeping rate (). This behaviour may be due to the overall charge storage associated with the redox transformations of the stand-alone electrode of the PAN–PVA composite film, which may be controlled by the capacitive behaviour of deeply trapped ions accessed at extended experimental timescales and extreme oxidative potentials.
Fig. 4 Charging–discharging cycles of the standalone composite film as a function of scan rate calculated from the data in .
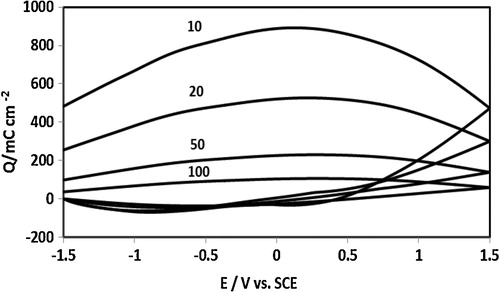
The electrochemical capacitance of the stand-alone electrode was calculated from the potential-dependent redox transformations represented in at different scan rates from the equation:(1)
(1) where Ce is the electrochemical capacitance, Iav is the average current of the redox cycle, and v is the scan rate at particular potential cycles.
The electrochemical capacitance of the stand-alone composite film as a function of the scan rate is shown in . As discussed above, the electrochemical capacitance of the stand-alone film is a function of the experimental timescale. At shorter experimental times (faster scan rate), the film capacitance fell significantly, whereas at longer times (slower scan rate), capacitive behaviour increased dramatically. The inset in shows the inverse relation between film electrochemical capacitance and experimental scan rate. We envisage that the slow kinetics of mass transport (ion–solvent transfer) in the polymer film results in a fall in capacitance at higher scan rates. At lower scan rates (extended experimental timescale), the electrochemical response of deeply trapped ions dominates film capacitance.
4 Conclusions
The stand-alone electrode of the PAN–PVA composite film showed a wide electrochemical window, which is crucial for energy storage and wide-range charge–discharge cycling experiments. The redox behaviour of the composite film exhibited capacitive responses at extreme oxidation potentials and an extended experimental timescale (slow scan rate). Depending on the potential sweeping scan rate (experimental timescale), electrochemical appraisal of the composite film showed two distinctive current responses that were, accordingly, assigned to two doping and de-doping modes, represented as deep and shallow trapped ions during film redox transformation. With regard to electrochemical capacitance, the stand-alone electrode of the composite film showed a high timescale-dependent response that decreased dramatically at faster potential scans.
Supplementary data
Download MS Word (495.2 KB)Acknowledgements
The authors are grateful to the Deanship of Scientific Research of Taibah University for financial support (grant no. 739/431; M.A. Mohamoud and grant no. 658/432; S. Ben Aoun).
Notes
Peer review under responsibility of Taibah University
References
- G.InzeltConducting Polymers: A New Era in Electrochemistry2nd ed.2012SpringerBerlin
- X.J.SongJ.H.YuanF.LiD.X.HanJ.F.SongL.NiuTunable activity in electrochemical reduction of oxygen by gold–polyaniline porous nanocompositesJ. Solid State Electrochem.1420101915
- H.K.SongG.T.R.PalmoreRedox-active polypyrrole: toward polymer-based batteriesAdv. Mater.1820061764
- Y.KiyaJ.C.HendersonH.D.Abruña4-Amino-4H-1,2,4-triazole-3,5-dithiol: a modifiable organosulfur compound as a high-energy cathode for lithium-ion rechargeable batteriesJ. Electrochem. Soc.1542007A844
- G.TsekourasC.O.TooG.G.WallacePhotovoltaic properties of poly(terthiophene) doped with light-harvesting dyes and photocurrent generation mechanismSynth. Met.1572007441
- G.ZerzaB.RöthlerN.S.SariciftciR.GómezJ.L.SeguraN.MartinPhotophysical properties and optoelectronic device applications of a novel naphthalene–vinylene type conjugated polymerJ. Phys. Chem. B10520014099
- C.MarcelJ.M.TarasconAn all-plastic WO3·H2O/polyaniline electrochromic deviceSolid State Ionics143200189
- L.ZhaoY.XuT.QiuL.ZhiG.ShiPolyaniline electrochromic devices with transparent graphene electrodesElectrochim. Acta552009491
- C.DhandM.DasM.DattaB.D.MalhotraRecent advances in polyaniline based biosensorsBiosens. Bioelectron.2620112811
- X.FengC.MaoG.YangW.HouJ.J.ZhuPolyaniline/Au composite hollow spheres: Synthesis, characterization, and application to the detection of dopamineLangmuir2220064384
- A.T.RamaprasadV.RaoChitin–polyaniline blend as humidity sensorSens. Actuators B: Chem.1482010117
- C.O.BakerB.SheddP.C.InnisP.G.WhittenG.M.SpinksG.G.WallaceR.B.KanerMonolithic actuators from flash-welded polyaniline nanofibersAdv. Mater.202008155
- W.LuA.G.FadeevB.QiE.SmelaB.R.MattesJ.DingG.M.SpinkJ.MazurkiewiczD.ZhouG.G.WallaceD.R.MacFarlaceS.A.ForsythM.ForsythUse of ionic liquids for π-conjugated polymer electrochemical devicesScience2972002983
- L.NiuC.KcarnströmA.IvaskaMixed ion transfer in redox processes of poly(3,4-ethylenedioxythiophene)J. Electroanal. Chem.5692004151
- M.A.SkopekM.A.MohamoudK.S.RyderA.R.HillmanNanogravimetric observation of unexpected ion exchange characteristics for polypyrrole film p-doping in a deep eutectic ionic liquidChem. Commun.82009935
- J.M.PernautJ.R.ReynoldsUse of conducting electroactive polymers for drug delivery and sensing of bioactive molecules. A redox chemistry approachJ. Phys. Chem. B10420004080
- A.R.HillmanM.A.MohamoudIon, solvent and polymer dynamics in polyaniline conducting polymer filmsElectrochim. Acta5120066018
- G.FabregatE.Córdiva-MateoE.ArmelinO.BertranC.AlemánUltrathin films of polypyrrole derivatives for dopamine detectionJ. Phys. Chem. C115201114933
- C.S.RyooE.Y.JeonJ.H.KimJ.H.RheeH.LeePhotoexcitation of polyaniline and the derivativesSynth. Met.551993200
- X.ZhaoX.HuC.Y.YueX.XiaL.H.GanSynthesis, characterization and dual photochroic properties of azo-substituted polythiophene derivativesThin Solid Films417200295
- A.R.HillmanM.A.MohamoudI.EfimovTime–temperature superposition and the controlling role of solvation in the viscoelastic properties of polyaniline thin filmsAnal. Chem.8320115696
- D.S.PatilJ.S.SkaikhD.S.DalaviS.S.KalagiP.S.PatilChemical synthesis of highly stable PVA/PANI films for supercapacitor applicationMater. Chem. Phys.1282011449
- T.C.GirijaM.V.SangaranarayananAnalysis of polyaniline-based nickel electrodes for electrochemical supercapacitorsJ. Power Sources1562006705
- J.LaskaK.ZakA.ProńConducting blends of polyaniline with conventional polymersSynth. Met.841997117
- S.PrakashD.R.K.RaoM.V.JiyanNew polyaniline(PAni)-polyelectrolyte (PDDMAC) composites: synthesis and applicationsElectrochim. Acta5320085704
- B.GuptaR.PrakashProcessible polyacid doped polyaniline composites: application for charge storage devicesMater. Sci. Eng. C2920091746
- Z.ZhangM.WanComposite films of nanostructured polyaniline with poly(vinyl alcohol)Synth. Met.128200283
- R.GangopadhyayA.DeG.GhoshPolyaniline–poly(vinyl alcohol) conducting composite: material with easy processability and novel application potentialSynth. Met.123200121
- X.ChenC.K.Y.WongC.A.YuanG.ZhangImpact of the functional group on the working range of polyaniline as carbon dioxide sensorsSens. Actuators B: Chem.175201215
- M.BlomquistJ.BobackaA.IvaskaK.LevonElectrochemical and spectroscopic study on thiolation of polyanilineElectrochim. Acta902013604
- A.R.HillmanQ.DongM.A.MohamoudI.EfimovCharacterization of viscoelastic properties of composite films involving polyaniline and carbon nanotubesElectrochim. Acta5520108142
- Q.ZhangY.liY.FengW.W.FengElectropolymerization of graphene oxide/polyaniline composite for high-performance supercapacitorElectrochim. Acta90201395
- T.C.MoH.W.WangS.Y.ChenY.C.YehSynthesis and dielectric properties of polyaniline/titanium dioxide nanocompositesCeram. Int.3420081767
- X.X.LiuY.Q.DouJ.WuX.Y.PengChemical anchoring of silica nanoparticles onto polyaniline chains via electro-co-polymerization of aniline and N-substituted aniline grafted on surfaces of SiO2Electrochim. Acta5320084693
- J.BhadraD.SarkarSize variation of polyaniline nanoparticles dispersed in polyvinyl alcohol matrixBull. Mater. Sci.332010519
- M.A.MohamoudPolyaniline/poly(vinyl alcohol) (PAN/PVA) composite films: the synergy of film structural stiffening, redox amplification, and mobile species compositional dynamicsJ. Solid State Electrochem.1720132771
Appendix A
Supplementary data
Supplementary data associated with this article can be found, in the online version, at doi:10.1016/j.jtusci.2014.03.001.
Appendix A
Supplementary data
The following are the supplementary data to this article: