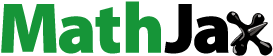
Abstract
Proteases have characteristics of biotechnological interest and have thus become important industrial enzymes. We report the production of thermostable protease and its characterization in Bacillus species, which is a thermotolerant bacterium; Bacillus subtilis is widely used for isolating protease enzyme. Gelatin was used as the substrate in nutrient agar medium for screening and showed the maximum zone of activity (22 mm) after overnight incubation and addition of the indicator. Under submerged fermentation conditions, a high level of protease production was found at 45 °C after 36 h at pH 10, with continuous agitation (180 rpm). The presence of galactose and peptone in the medium enhanced enzyme production by 0.5% when compared with other carbon and nitrogen sources. Thus, such additions can augment protease production and their application in various industries.
1 Introduction
Microorganisms are known to play a vital role in technology for the production of intracellular and extracellular enzymes on an industrial scale [Citation1,Citation2]. For maximum yield, selected organisms are grown in fermenters under optimum conditions and can be further used to make products such as cheese, bread, wine and beer [Citation4–Citation6]. Most reactions inside living cells require enzymes, which act as catalysts and are essential for life [Citation3,Citation7,Citation8].
Bacillus species are the main producers of extracellular proteases, and industrial sectors frequently use Bacillus subtilis for the production of various enzymes. Proteases are the main enzymes produced from microbial sources, of which only few are recommended as commercial producers. B. subtilis is found mainly in soil and is also known as hay bacillus and grass bacillus. It is a rod-shaped organism, which can form a tough, protective endospore and can withstand extreme environmental conditions. Bacillus species are obligate aerobes or facultative anaerobe and include both free-living and pathogenic species [Citation9,Citation10]. In view of their wide application in various industries, protease enzymes occupy an important position [Citation11].
Alkaline proteases constitute 60–65% of the global industrial market [Citation12,Citation13]. These proteases are the single class of enzymes widely used in detergents, pharmaceuticals, leather and the food and agriculture industries [Citation14,Citation15]. B. subtilis is widely used for the production of specific chemicals and industrial enzymes [Citation16,Citation17,Citation1]. Proteases are important components of biopharmaceutical products such as contact lens cleaners and enzymatic debriders [Citation18]. Proteolytic enzymes support the natural healing process in local management of skin ulceration by efficient removal of necrotic material [Citation19].
Proteases catalyze or hydrolyze protein [Citation20] and therefore play a vital role in various industrial applications [Citation21,Citation22]. Hyperactive strains are being sought for use in different industries [Citation23]. Conversion of wastes into useful biomass by microorganisms and their enzymes is a new trend, and new protease-producing microorganisms and perfected fermentation technology are needed to meet the ever-growing demand for this enzyme [Citation24]. The aim of the present study was to purify and characterize alkaline protease from B. subtilis and optimize the conditions for maximum production of extracellular protease.
2 Materials and methods
2.1 Microorganism and culture conditions
Soil samples were collected from side roads near Armats Biotek Institute in Chennai. Serial dilutions of the samples were made up to 10−5 dilutions, spread on nutrient agar plates and incubated for 24 h at 37 °C. The colonies were identified as B. subtilis on the basis of morphological and biochemical characteristics, including endospore staining. Colonies were subcultured and maintained to obtain a pure culture on nutrient agar plates, which was transferred to a medium (pH 8.0) containing 1% glucose, 0.5% casein, 0.55% yeast extract, 0.2% KH2PO4, 1% Na2CO3, 0.2% MgSO4·7H2O, pH 8 and incubated at 37 °C with continuous shaking.
2.2 Substrate screening and qualitative assay of protease
Gelatin was used as the substrate in nutrient agar medium. The culture was streaked in a straight line and incubated at 37 °C. After 12 h, the plates were flooded with 15% HgCl2 solution in 20% HCl for 5 min. Substrate gelatin at 1% (w/v) was then added to nutrient agar medium, sterilized and poured into Petri plates. Wells were made with a sterile cork borer, and 100–200 μL of cell-free culture filtrate were dispensed aseptically into each well and incubated at 37 °C. After 12 h, enzyme activity was visualized as clear zones around the wells due to hydrolysis of substrates in the presence of indicator solution, and the diameter of the proteolytic zone was measured.
2.3 Shake flask fermentation with selected medium
Alkaline protease was produced in an optimized production medium of pH 8.0 containing 1% galactose, 0.5% casein, 0.55% peptone, 0.2% KH2PO4, 1% Na2CO3 and 0.2% MgSO4·7H2O; 4% inoculum was added to the production medium and incubated with continuous shaking. Shaker fermentations were carried out at 37 °C for 72 h with controlled agitation at 150–200 rpm. At the end of the fermentation period, the whole culture broth was centrifuged at 5000 rpm for 30 min to remove debris, and the supernatant was collected and used for further experiments.
2.4 Effect of incubation time on protease production
The optimized protease production medium was prepared in six 100-mL conical flasks, into which 1% B. subtilis culture broth was inoculated and kept for shaker fermentation. Every 12 h, each flask was filtered, followed by centrifugation at 5000 rpm for 30 min. The culture filtrate solution obtained was used to assay protease by qualitative and quantitative methods.
2.5 Effect of carbon and nitrogen sources on protease production
The effect of carbon and nitrogen sources for protease production was determined with simple and complex carbon sources (sucrose, maltose, xylose, galactose and starch) instead of dextrose and with peptone, ammonium nitrate, ammonium sulphate, urea and ammonium chloride as the nitrogen source instead of yeast extract. Each source was used at a concentration of 1% (w/v) and 0.55% (w/v) to replace carbon and nitrogen sources, respectively. Protease yield was determined after 72 h of incubation at 37 °C with shaking at 150–200 rpm. After production, the yield was estimated quantitatively.
2.6 Separation and purification of protease
The culture was harvested after 2 days’ growth at room temperature. The crude enzyme preparation was subjected to ammonium sulphate precipitation, and the harvested culture was filtered through Whatman no. 1 filter paper of pore size 125 mm and centrifuged (REMI C-24BL) at 5000 rpm for 30 min at 4 °C. Ammonium sulphate was added slowly to the cell-free culture filtrate at 75% saturation to precipitate the protein, with continuous shaking and a magnetic stirrer. The precipitated protein was separated by centrifugation at 5000 rpm for 30 min and was dissolved in 0.5 mmol/L phosphate buffer, pH 7, dialysed and stored. This source of crude enzyme was used for further enzyme characterization. At each step, the protease activity and total protein content were measured in an ultraviolet spectrophotometer.
2.7 Quantitative assay of protease
The protease activity in the culture filtrate of B. subtilis was assayed in a mixture containing 0.5 mL of 0.65% gelatin as the substrate and 1 mL of cell-free culture filtrate as the enzyme source. The mixture was incubated at 37 °C for 10 min, and then 5 mL of 110 mmol/L trichloroacetic acid (TCA) were added to the reaction mixture to terminate the enzyme reaction. The reaction mixture was centrifuged (REMI C-24BL) at 5000 rpm for 30 min, and the supernatant was collected. To 2 mL of supernatant, 5 mL of alkaline solution (500 mmol/L of Na2CO3) and 1 mL of 25% Folin phenol reagent (25 mL of reagent diluted with 100 mL of glass-distilled water before use) were added and the mixture was incubated at room temperature. After 30 min, the protease activity was read at 650 nm in a spectrophotometer (U-2900 UV/VIS). Simultaneous controls containing enzyme, heat-killed enzyme and substrate were maintained. One unit of protease activity was defined as the amount of enzyme required to liberate 1 μmol tyrosine/mL per min. The protein content of the culture filtrate was estimated by the dye-binding method of Bradford [Citation34].
Enzyme activity was calculated from the formula
2.8 Effect of pH on activity and stability of protease
Partially purified enzyme in a volume of 500 μL was made up to 1 mL with buffers of various pH, and 0.5 mL gelatin was added. The buffers used were 0.1 mol/L acetate buffer (pH 5, 5.4 and 5.8), 0.1 mol/L phosphate buffer (pH 6, 6.4, 6.8, 7.4 and 8.0) and 0.1 mol/L Tris buffer (pH 8.8, 8.6 and 9.0). The enzyme and substrate mixture was incubated at room temperature. After 60 min, the reaction was terminated by adding 5 mL of 110 mmol/L TCA. The enzyme and buffer mixture, without substrate, were pre-incubated for 30 min at room temperature; then, 5 mL of 0.65% gelatin were added to the suspensions, and the residual protease activity and stability were estimated quantitatively in a spectrophotometer.
2.9 Effect of temperature on activity and stability of protease
Partially purified enzyme in a volume of 500 μL was made up to 1 mL with distilled water, to which 0.5 mL of 0.65% gelatin was added. The enzyme and substrate mixture was incubated for 30 min at 40 °C, 50 °C, 60 °C, 80 °C and 100 °C, and the reaction was terminated by adding 5 mL of 110 mmol/L TCA to the suspension. Residual protease activity was measured. As a control, the enzyme and buffer mixture was incubated at the different temperatures without substrate; after 30 min, 0.5 mL of 0.65% gelatin was added, and residual protease activity was assayed.
3 Results
The production of enzymes from microorganisms is strongly influenced by the composition of the medium, temperature, pH, percentage of culture inoculated and length of incubation. B. subtilis showed higher proteolytic activity on 0.03 (w/v) gelatin than on casein or skimmed milk in nutrient agar medium (). Carbon and nitrogen sources are also important factors for the production of protease in culture medium. Of the five carbon and nitrogen sources tested, galactose and peptone resulted in the highest protease activity (236.31 and 175.083 U/mL per min, respectively), while the least enzyme activity was observed with starch and ammonium chloride ().
Fig. 2 (A) Effect of different carbon sources on protease production by Bacillus sp. (B) Effect of different nitrogen sources on protease production by Bacillus sp.
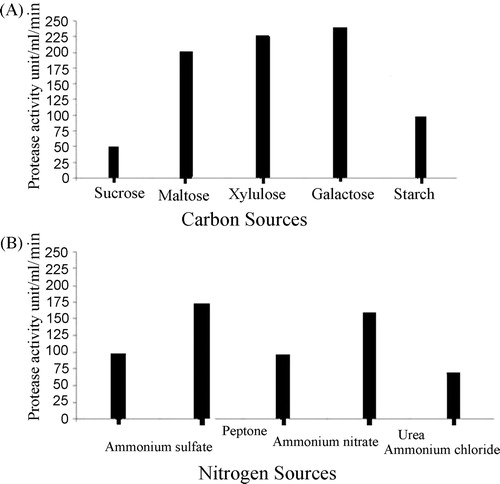
In tests to optimize the time, protease production increased gradually from 0 to 36 h, at which it was maximal, at 243.28 U/mL per min; it then decreased with time (). To determine the optimal H+ concentration for protease production, B. subtilis was cultivated over the pH range 5–9 at room temperature. The highest protease activity was found at pH 7.4 (143.73 U/mL per min; ). Residual protease activity was determined at different temperatures and was maximal at 40–50 °C, although considerable residual activity (50%) was observed even after incubation at 80 °C for 30 min (). Hence, protease obtained from B. subtilis can be considered a thermostable enzyme. To obtain proteins from the cell-free culture filtrate, they were precipitated with ammonium sulphate at 75% (w/v) saturation by adding powdered ammonium sulphate slowly with continuous shaking and a magnetic stirrer to avoid bubble formation. The protein precipitate was then dissolved and dialysed for 24 h against phosphate buffer. The yield of protein was 2.31 mg/mL, with a total activity of 475.56 U/mL per min.
4 Discussion
B. subtilis had high proteolytic activity, with a clear zone of 22 mm when gelatin was used as the matrix. Enzyme production began 6 h after cell entrapment of gelatin and increased gradually to a maximum level of 10.8 U/mL; thereafter, enzyme production decreased [Citation25].
B. subtilis grew in the pH range 6–9, with optimum protease secretion at pH 7.4 and galactose and peptone as the carbon and nitrogen source, respectively, at specific incubation times and temperature. It was observed previously that use of casein as the substrate under the standard assay conditions gave the highest activity at pH 8.0 [Citation26,Citation33]. Other experiments showed that an incubation temperature of 37 °C, pH 9.0 and glucose and sodium nitrate as the carbon and nitrogen source, respectively, resulted in the highest production of protease [Citation27,Citation28]. Protease yields vary considerably with temperature and pH [Citation30,Citation31], which may be attributed to other components of the medium and their combined influence on the metabolism of the bacterial species [Citation29,Citation32]. In the present experiment, a high yield of protease was found at 50 °C and when alternate nitrogen and carbon sources were used.
5 Conclusion
B. subtilis can be used for large-scale production of alkaline protease to meet present-day needs in the industrial sector. In the present study, a natural polymer gelatin was used with the nutrient agar medium to help in cell immobilization for maximum production of alkaline protease by strains of B. subtilis. We also determined the optimum growth parameters for cultivating the organism: maximum production of alkaline protease was observed at pH 7.4 and a temperature of 50 °C with galactose and peptone as the carbon and nitrogen sources, respectively. As alkaline protease has more applications than protease in industry, alkaline protease production from B. subtilis is recommended.
Acknowledgements
The authors are grateful to the management and staff of GITAM University, Visakhapatnam, Andhra Pradesh, India, and H.N.B. Garhwal University (Central University), Srinagar, Uttarakhand, India, for providing facilities for the research.
Notes
Peer review under responsibility of Taibah University.
References
- R.GuptaQ.K.BegP.LorenzBacterial alkaline proteases: molecular approaches and industrial applicationsAppl. Microbiol. Biotechnol.5920021532
- M.Moo-youngY.ChistiBiochemical engineering in biotechnologyPure Appl. Chem.661994117136
- J.K.SiereckaPurification and partial characterization of a neutral protease from a virulent strain of Bacillus cereusInt. J. Biochem. Cell Biol.301998579595
- Y.LinS.TanakaEthanol fermentation from biomass resources: current state and prospectsAppl. Microbiol. Biotechnol.692006627642
- K.BrijwaniH.S.OberoiP.V.VadlaniProduction of a cellulolytic enzyme system in mixed-culture solid-state fermentation of soybean hulls supplemented with wheat branProcess Biochem.452010120128
- P.M.SchenkS.R.Thomas-HallE.StephensU.C.MarxJ.H.MussgnugC.PostenO.KruseB.HankamerSecond generation biofuels: high-efficiency microalgae for biodiesel productionBioenerg. Res.120082043
- L.JohnsonSymmetry at the molecular level in biologyEur. Rev.1320057795
- A.PingoudM.FuxreiterV.PingoudW.WendeType II restriction endonucleases: structure and mechanismCell. Mol. Life Sci.622005685707
- S.A.DubalY.P.TilkariS.A.MominI.V.BorkarBiotechnological routes in flavour industriesAdv. Biotechnol.620083045
- B.S.SekhonFood nanotechnology—an overviewNanotechnol. Sci. Appl.32010115
- P.WidstenA.K.LaccaseApplications in the forest products industry: a reviewEnzyme Microb. Technol.422008293307
- L.M.ZanphorlinF.D.A.FacchiniF.VasconcelosR.C.B.SantosA.RodriguesL.D.SetteE.GomesG.O.B.RodriguezProduction, partial characterization, and immobilization in alginate beads of an alkaline protease from a new thermophilic fungus Myceliophthora sp.J. Microbiol.482000331336
- S.A.SawiH.M.LabdaneP.AbietAn editerpene from the fruits of Juniperus phoenicea L. grown in Egypt and their activities against human liver carcinomaAust. J. Med. Herbal.22008115122
- M.B.RaoA.M.TanksaleM.S.GhatgeV.V.DeshpandeMolecular and biotechnological aspects of microbial proteaseMicrobiol. Mol. Biol.621998597635
- O.A.T.AzuraL.K.AbubakarF.HamidN.S.A.RaduS.S.NazamidPhenotypic and molecular identification of a novel thermophilic Anoxybacillus species: a lipase-producing bacterium isolated from a Malaysian hotspringWorld J. Microbiol. Biotechnol.25200919811988
- P.EsakkirajG.ImmanuelS.M.SowmyaP.IyapparajA.PalavesamEvaluation of protease-producing ability of fish gut isolate Bacillus cereus for aqua feedFood Bioprocess. Technol.22009383390
- W.H.ChuOptimization of extracellular alkaline protease production from species of BacillusJ. Ind. Microbiol. Biotechnol.342007241245
- A.AnwarM.SaleemuddinAlkaline protease from Spilosoma obliqua: potential applications in bio-formulationsBiotechnol. Appl. Biochem.3120008589
- J.SjodahlA.EmmerJ.VincentJ.RoeraadeCharacterization of proteinases from Antarctic krill (Euphausia superba)Protein Expr. Purif.262002153161
- M.SchallmeyA.SinghO.P.WardDevelopments in the use of Bacillus species for industrial productionCan. J. Microbiol.502004117
- R.NijlandO.P.KuipersOptimization of protein secretion by Bacillus subtilisRecent Pat. Biotechnol.220087987
- W.LiX.ZhouP.LuBottlenecks in the expression and secretion of heterologous proteins in Bacillus subtilisRes. Microbiol.1552004605610
- L.L.FuZ.R.XuW.LiJ.B.ShuaiP.LuC.X.HuProtein secretion pathways in Bacillus subtilis: implication for optimization of heterologous protein secretionBiotechnol. Adv.252007112
- P.RathakrishnanP.NagarajanR.R.KannanOptimization of process parameters using a statistical approach for protease production by Bacillus subtilis using cassava wasteInt. J. Chem. Tech. Res.42012749760
- R.KumarR.VatsProtease production by Bacillus subtilis immobilized on different matricesN. Y. Sci. J.320102024
- J.K.YangaI.L.ShihbY.M.TzengcS.L.WangaProduction and purification of protease from a Bacillus subtilis that can deproteinize crustacean wastesEnzyme Microb. Technol.262000406413
- R.S.RaoY.D.DeshmukhP.S.BorkarC.N.KhobragadeProduction of alkaline protease from Bacillus subtilis using rice branJ. Cell Tissue Res.8200813471350
- A.S.QureshiM.A.BhuttoI.KhushkM.U.DahotOptimization of cultural conditions for protease production by Bacillus subtilis EFRL 01Afr. J. Biotechnol.10201151735181
- A.SonnleitnerBiotechnology of thermophilic bacteria—growth, products, and applicationAdv. Biochem. Eng. Biotechnol.28198369138
- V.MaghsoodiA.KazemiP.NahidS.YaghmaeiM.A.SabzevariAlkaline protease production by immobilized cells using B. licheniformisSci. Iran.202013607610
- B.K.BajajG.JamwalThermostable alkaline protease production from Bacillus pumilus D-6 by using agro-residues as substratesAdv. Enzyme Res.120133036
- P.VijayaraghavanS.LazarusS.GnanaP.VincentDe-hairing protease production by an isolated Bacillus cereus strain AT under solid-state fermentation using cow dung: biosynthesis and propertiesSaudi J. Biol. Sci.2120142734
- D.KaradagA.E.MakinenE.EfimovaJ.A.PuhakkaThermophilic biohydrogen production by an anaerobic heat treated-hot spring cultureBioresour. Technol.100200957905795
- B.StarcherA ninhydrin–based assay to quantitate the total protein content of tissue smaplesAnal. biochem.2922001125129