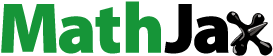
Abstract
The removal and recovery of rosaniline and brilliant green were studied using batch and dynamic processes. The maximum sorption of these dyes (∼100%) was obtained in a pH range of 6–10 with a shaking time of 2–5 min. The sorption kinetics were best described by the pseudo-second-order model (R2 = 0.993). The variation in the sorption of rosaniline and brilliant green with temperature results in average values of ΔH and ΔG (i.e., −65.7 kJ mol−1 and −8.1 kJ mol−1, respectively). The sorption capacity of iodo-polyurethane powder (Iodo-PUP) was 106.8 and 154.4 mg g−1 for rosaniline and brilliant green, respectively. The dye removal percentage and detection limit were 99.5% and 20 ng mL−1, respectively. The isotherms exhibit good correlation (R2 = 0.9985) with a zero intercept (0.0045). Successful application was achieved for environmental samples (i.e., industrial, laundry and sewage wastewater). The average recovery and RSD were 85.2% and 4.9%, respectively.
1 Introduction
Dyes are used extensively in the textile, leather, food processing, cosmetics, paper, and dyeing industries [Citation1]. Dyes become water pollutants due to their presence in the effluents of dye manufacturing industries [Citation2]. Over 105 commercially available dyes exist, and more than 7 × 105 tonnes of dye are produced annually [Citation3]. However, approximately 7 × 104 tonnes/year of dyes are discharged into waste streams by the textile industry [Citation4]. Dyes are carcinogenic and possess a serious hazard to aquatic living organisms [Citation5]. In addition, the dyes in water cause human health disorders, such as severe damage to the kidneys and central nervous system [Citation1]. In particular, basic dyes, such as triphenyl methane, are considered to be one of the more problematic classes of dyes [Citation1]. Therefore, the removal of dyes from effluents prior to mixing with natural water is very important. Adsorption is one of the most efficient methods of removing pollutants from wastewater [Citation6–Citation11]. The adsorption processes yield the best results because they can remove different types of colouring materials, especially if inexpensive adsorbents are available. Different sorbents have been used to remove dyes from wastewater (e.g., Ni0.6Fe2.4O4 nanoparticles, castor bean, lignocellulose, agricultural waste, cationic polymer, coal, carbonaceous and miswak) [Citation12–Citation22].
The polyurethane sorbent (PUF) is advantageous due to its simple preparation, low cost and high surface area [Citation23–Citation25]. PUF is a good sorbent for the removal of organic pollutants from aqueous solutions, such aromatic acids [Citation26], phenols [Citation27], herbicides [Citation28], β-lactam antibiotics [Citation29], azine and triphenyl methane dye [Citation30]. The Iodo-PUP sorbent was synthesized from PUF, which undergoes hydrolysis and diazotization by sodium nitrite followed by treatment with potassium iodide [Citation1]. The aim of this study is to develop a new, simple, cheap and rapid removal procedure for triphenyl methane basic dyes that have different molecular sizes from wastewater using the modified Iodo-PUP. Iodo-PUP is inexpensive with good stability and a high sorption capacity for the removal of basic dyes from aqueous solutions. In addition, Iodo-PUP can be recycled many times for the removal of dyes from wastewater without significantly decreasing its capacities. The removal behaviour of Iodo-PUP for dyes was studied to optimize the conditions for the optimal removal of dyes. Kinetic, thermodynamic and equilibrium data was obtained.
2 Experimental
2.1 Reagents
The rosaniline and brilliant green dyes were prepared from high purity grade reagents (BDH, Poole, England). A 1 mg mL−1 stock solution of the dyes was prepared by dissolving 0.1 g of rosaniline hydrochloride (C20H19N3 HCl, 337.86 g/mol) and brilliant green (C27H34N2O4S, 482.63 g/mol, λmax = 624.5 nm) in 100 mL of distilled water.
2.2 Apparatus
The rosaniline and brilliant green dyes were determined spectrophotometrically using a Shimadzu UV-1800 spectrophotometer (Shimadzu Corporation, Japan) at λmax 542 and 624.5 nm, respectively. The pH measurements were carried out using a pH metre from Microprocessor pH Meter (HANNA Instruments). The glass column, which contained 1.0 g of Iodo-PUP, was 50.0 cm long, 1.5 cm in diameter with a bed height of approximately 7.0 cm.
2.3 Recommended procedures
The sorption of rosaniline and brilliant green was carried out using a batch technique at 25 °C. A portion of the 0.1 g of Iodo-PUP was mixed with 25 mL of the studied dye solution (1 μg mL−1) in a shaker adjusted to the desired shaking speed. After a certain time period, the dye concentration remaining in the supernatant solution was determined spectrophotometrically.
In the dynamic experiments, 1.0 g of Iodo-PUP was packed into a column (50 cm × 1.5 cm). The bed height (L) of the Iodo-PUP column was approximately 7 cm. 25 mL of the studied dye solutions (1 μg mL−1) were passed through the Iodo-PUP column at a flow rate of 2–5 mL min−1. The stripping of the dye from the Iodo-PUP column was carried out using CH3OH. The eluates of the dyes were collected and determined spectrophotometrically.
To determine the pHpZC of Iodo-PUP, a series of flasks containing 25 mL of a solution with a pH in a range 1–13 (solution was adjusted using HCl and NaOH) was added to 0.1 g of Iodo-PUP. After 24 h, the final pH (pHf) of the solution was measured. The difference between the initial and final pH values (ΔpH = pHf − pHi) was plotted as a function of the pHi. The pHpZC was the pH at which the initial pH equals the final pH.
A 25 mL portion of each dye solution was shaken with 0.1 g of Iodo-PUP for 30 min. The pH of the dye solution was adjusted prior to equilibration with HCl or NaOH over a range of 1–14. After equilibration, the remaining dyes were determined using the recommended method.
The effect of the shaking time on the extraction efficiency of the dye was studied. 0.1 g of Iodo-PUP was added to a 25 mL of sample at the optimum pH, and automatic shaking was performed for different time intervals (1–60 min).
3 Results and discussion
3.1 Effect of pH on the sorption of dye onto Iodo-PUP
The effect of the pH on the sorption of rosaniline and brilliant green onto Iodo-PUP using the batch technique is shown in . The pH of the aqueous solution was an important parameter that controlled the uptake process. The maximum removal efficiency reached 97–100% for rosaniline and brilliant green in a pH range of 6–12 and 8–12, respectively. At a pH of less than 2.0, the uptake of the studied dyes is too small, and the uptake increased rapidly as the pH value increased. The optimum pH value for the removal of rosaniline and brilliant green from an aqueous solution was ≥8. The sorption percentage of rosaniline is greater than that of brilliant green at a pH of 4–7, which can play a role in enhancing the selectivity of the dye separation with Iodo-PUP. In addition, the sorption percentage of the studied dyes on Iodo-PUP and PUF was 98.5% and 57.5%, respectively. This result indicated that Iodo-PUP is more efficient than PUF for removing basic dyes from an aqueous solution.
3.2 Sorption kinetics
The sorption rate of rosaniline and brilliant green (0.004 mg mL−1) onto Iodo-PUP has been measured using a batch experiment at different time intervals (1–30 min). The time for 70–90% dye sorption is very fast but becomes progressively slower as the time increased. The time required for sorption equilibrium of the dyes was determined to be 2–6 min. This rapid sorption rate of dyes is due to the strong bond that is formed with the Iodo-PUP.
The diffusion mechanism was investigated using the Morris–Weber , Reichenberg [Bt = −0.4977 − ln(1 − F)] and [F = (6/R)(Dit/π)1/2], and Bangham [loglogCO/(CO − Qtm) = logkom/2.303V + αlogt] equations [Citation31–Citation33]. Qt is the amount of dye sorbed at time t. ki is the intraparticle diffusion rate constant (mmol g−1 min−1/2). Co is the initial concentration of dyes in the solution. V is the volume of the solution, and m is the mass of Iodo-PUP. The Bt value is a mathematical function of F = Qt/Qe. Di is the effective diffusion coefficient, and α and kO are constant.
A plot of Qt as a function of t1/2 should be a straight line (R2 = 0.942) that does not passing through the origin. The diffusion rate of the dyes is rapid in the initial stages and decreases with the passage of time. The average values of ki for dye sorption was 0.054 mmol g−1 min−1/2. The diffusion rate of brilliant green was greater than that of rosaniline, which indicated that the diffusion rate was independent of the size of the dye ().
Table 1 The diffusion rate for the sorption of rosaniline and brilliant green onto Iodo-PUP.
The relationship between Bt and t for the sorption of rosaniline and brilliant green was investigated (R2 = 0.983), which indicates that a partial film formed along with intraparticle diffusion. The slope of the linear plots of F as a function of t1/2 provide the numerical values of the effective diffusion coefficient (Di). The average value of Di for the dye sorption was 2.06 × 10−6 cm2 min−1 (). The film diffusion rate depended on the size of dyes.
The double logarithmic plots of the Bangham equation as a function of the time yield perfect linear plots, and the average of the correlation coefficients was 0.972 (). This result indicates that the diffusion of basic dyes into the pores of Iodo-PUP was involved in the rate-controlling step. The average value of α was calculated from the slope to be 0.88. The α values increased with as the dye size increased (). This result is in a good agreement with the results obtained from the Reichenberg model. The regression correlation coefficient (R2) exceeded 0.94 for the Morris–Weber, Reichenberg and Bangham models, suggesting that both models closely fitted the experimental data. In addition, the regression analysis results indicated that the Reichenberg model (R2 = 0.983) was able to describe the intraparticle diffusion mechanism as film diffusion.
Fig. 2 Morris–Weber, Reichenberg and Bangham diffusion models for the sorption of rosaniline and brilliant green.
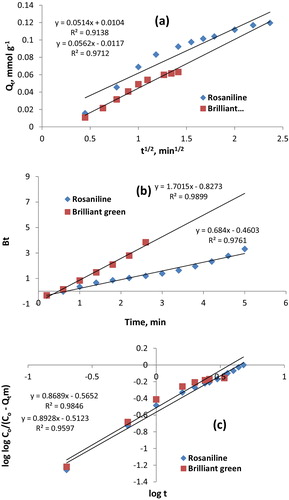
Kinetic modelling of the experimental data allows us to gain insight into the potential rate-controlling steps involved in the sorption process. Two kinetic models (i.e., pseudo-first-order [log(Qe − Qt) = (logQe) − (k1t/2.303)] and pseudo-second-order models) were tested to fit the experimental data for the sorption of rosaniline and brilliant green onto Iodo-PUP (). Qe is the amount of dye sorbed at equilibrium, k1 is the pseudo-first-order rate constant, and k2 is the pseudo-second-order rate constant.
Fig. 3 Pseudo-first-order and pseudo-second-order plots for the sorption of rosaniline and brilliant green.
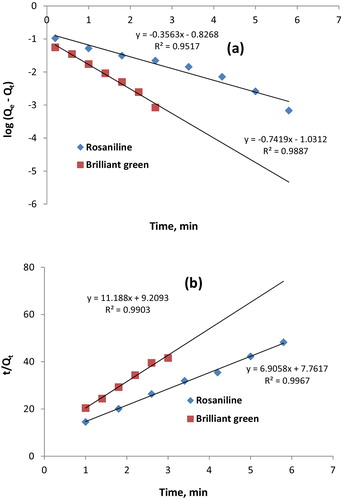
lists the kinetic parameters for the sorption of dyes using the pseudo-first-order and pseudo-second-order models. By comparing the correlation coefficients (R2), we determined that the sorption kinetics were best described by the pseudo-second-order model. The R2 values for the pseudo-second-order sorption model (0.993) were higher than those for the pseudo-first-order kinetic model (0.969). This result suggests that the pseudo-second-order adsorption mechanism is the predominant process, and the overall rate of the dye sorption process appears to be controlled by a chemisorption process. The average value of the sorption rate constant (k2) calculated from the slope of the line was 6.87 g mmol−1 min−1 (). The trend in the k2 values was in the order brilliant green > rosaniline, which indicated that the rate constant was independent of the size of the dye. The average value of the half-life (t1/2 = 1/Ck2) was 1.05 min for the sorption of the studied dyes.
Table 2 Kinetic parameter of pseudo first order and pseudo second order models.
3.3 Sorption isotherms
The effect of the initial dye concentration on the sorption of rosaniline and brilliant green onto Iodo-PUP using a batch technique was studied. The amount of sorbed dye per unit mass of Iodo-PUP (Qc) was plotted as a function of the initial dye concentration. The isotherms exhibit good correlations (R2 = 0.9985) with a zero intercept (0.0045, ). The sorption capacity initially increased with the initial concentration of dye and then plateaued. The sorption capacities of Iodo-PUP for rosaniline and brilliant green were 0.316 and 0.320 mmol g−1, respectively (106.8 and 154.4 mg g−1, respectively). The sorption capacity of Iodo-PUP was compared to that of other sorbents, which indicated that Iodo-PUP exhibited better capacity values [Citation22,Citation34–Citation37]. A regression analysis indicated that the average limit of detection (LOD = 3SDI/b, where SDI is the standard deviation of the intercept and b is the slope of the calibration curve) for the sorbed dye was 4.2 × 10−5. In addition, the average limit of quantitation (LOQ = 10SDI/b) was 1.4 × 10−4.
The data from the sorption isotherms were analyzed using Freundlich (logQc = logKF + (1/n)logCe) and Langmuir [Ce/Qc = (1/KLb) + (Ce/KL)] equations [Citation38,Citation39], where Ce is the amount of dye sorbed at equilibrium. KF, n, KL, b, ɛ, and β are constants. KF is the adsorption capacity of the Iodo-PUP sorbent, and the 1/n constant is the intensity of the adsorption.
The linear plot of log Qc as a function of log Ce (R2 = 0.964) is shown in . The Freundlich constants (1/n) are 0.37 and 0.26 for sorbed rosaniline and brilliant green, respectively (). The value of 1/n was <1, which may be due to the heterogeneous surface structure of Iodo-PUF with a non-uniform distribution of heat sorption over the surface. The values of n were 2.7 and 3.8 for the sorption of rosaniline and brilliant green, respectively, indicating that the sorption is favourable.
Table 3 Comparison between Freundlich and Langmuir isotherm parameters.
Fig. 5 Langmuir, Freundlich and Dubinin Radushkevich isotherm models for the sorption of basic dyes onto Iodo-PUP.
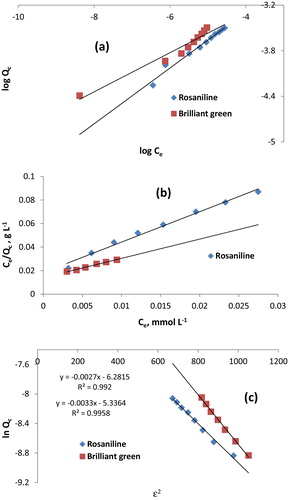
The plot of Ce/Qc as a function of Ce of the experimental data according to Langmuir model gives a linear relationship. The results in indicate that the Langmuir equation provides an accurate description of the experimental data, which was confirmed by correlation coefficient (R2 = 0.929). The dimensionless constant (RL), which was used to determine the favourability of the sorption of dyes onto Iodo-PUP, can be calculated using the following equation: RL = 1/(1 + bCO), where CO is the highest initial concentration of the dye. The RL values were determined to be 0.06 and 0.03 (0 < RL < 1) for the sorbed rosaniline and brilliant green, respectively, indicating that the sorption of these dyes was favourable.
The regression correlation coefficient (R2) exceeded 0.96 for both the Freundlich and Langmuir models, suggesting that both models closely fitted the experimental data. The Chi-square model (X2 = ∑(Qc − Qe)2/Qe) was used to compare the Freundlich and Langmuir models (). Here, Qc, which is the capacity, is calculated from the model and the experimental data of the capacity. The experimental data obtained for Iodo-PUP exhibited a better fit to the Langmuir isotherm model. This result is based on the lower X2 of 0.001 compared to those obtained using the Freundlich isotherm model (X2 = 20.0), which may suggest that Iodo-PUP has homogeneous adsorption sites.
The linear plot of ln Qc as a function of ɛ2 (Dubinin–Radushkevich isotherm [Citation24], lnQc = lnKD−R − βɛ2 and , R2 = 0.994) is shown in . KD–R is the maximum amount of dye sorbed onto Iodo-PUP, β is a constant related to the transfer energy of the dye from the bulk solution to the Iodo-PUP sorbent, and ɛ is the Polanyi potential. The β values for the sorption of rosaniline and brilliant green were −0.002 and −0.003 kJ2 mol−2, respectively. The sorption energy (ΔE) was determined to be 15.8 and 12.9 kJ mol−1 for rosaniline and brilliant green, respectively (). The ΔE value >8 kJ mol−1 corresponded to sorption processes controlled by an ion-exchange mechanism. Therefore, this study suggests that the sorption of basic dyes onto Iodo-PUP is due to an ion-exchange process. Finally, the sorption of basic dyes onto Iodo-PUP may proceed via ion-association complexes and an ion-exchange mechanism.
Table 4 Dubinin Radushkevich isotherm parameters.
3.4 Thermodynamic investigations
The effect of temperature on the sorption of rosaniline and brilliant green onto Iodo-PUP using a batch technique was studied. The results indicated that the sorption of the dyes decreased with increasing temperature. The decrease in dye sorption onto the sorbent was due to the deformation of bonds between the dye molecules and the active site of the sorbent. In addition, the solubility of the dyes in the aqueous solution increased with increasing temperature.
The thermodynamic behaviour of the sorption of the studied dyes onto Iodo-PUP was evaluated using the following equations: lnK = − ΔH/RT + ΔS/R, ΔG = ΔH − TΔS and K = Ca/Ce [Citation27], where K is the distribution coefficient for sorption, Ca is the equilibrium dye concentration on the sorbent and Ce is the equilibrium dye concentration in solution (mol L−1). The linear plots of ln K as a function of 1/T (, R2 = 0.993) yields the enthalpy (ΔH) and entropy (ΔS) values from the slope and intercept, respectively.
The average free energy (ΔG) value was −8.7 kJ mol−1, and the negative sign is due to the spontaneous nature of the sorption process (). The ΔH values for the sorption of rosaniline and brilliant green were −104.5 and −39.3 kJ mol−1, respectively. The negative ΔH value indicates an exothermic chemisorption process. The average value of ΔS was −193.4 J K−1 mol−1. The negative ΔS values indicated a decrease in the randomness at the solid-liquid interface during the sorption process.
Table 5 Thermodynamic parameters of the sorption basic dyes onto Iodo-PUP.
3.5 Preconcentration
The performance of Iodo-PUP for the preconcentration of each dye was studied. The preconcentration of rosaniline and brilliant green in different sample volumes (10–100 mL) using the batch technique was studied using 0.1 g of Iodo-PUP. The data indicate that the maximum sorption of rosaniline and brilliant green in the batch factor (V/m) ranges from 250 to 500 () (i.e., V is the sample volume of the dye and m is the mass of Iodo-PUP). The average uptake values of the studied dyes decreased with increasing sample volume (from 100% to 52%) in the batch factor, V/m ≈ 1000. This result indicates that the tested dyes can be sensitively concentrated and effectively removed from large volumes of dilute aqueous solutions using Iodo-PUP.
3.6 Effect of eluting agents and reusability of Iodo-PUP
The effect of various eluting agents, such as HCl, NaOH, CH3OH C2H5OH and CH3COCH3, on the stripping of rosaniline and brilliant green from Iodo-PUP was studied. The dyes were completely eluted from Iodo-PUP with CH3OH. However, the dyes were partially eluted with HCl, NaOH, C2H5OH and CH3COCH3. Iodo-PUP was recycled many times after regeneration using CH3OH and H2O without significantly decreasing their capacities.
3.7 Effect of sample and eluent flow rate
The uptake percentage of the studied dyes onto the Iodo-PUP column has been studied at various flow rates of the sample solution. A set of solutions containing 5 mg of rosaniline or brilliant green was passed through the Iodo-PUP column at a flow rate varying from 1 to 50 mL min−1. The average values of the optimum flow rates of the dye through the Iodo-PUP column were in the 1–19 mL min−1 range.
The elution of 5 mg of rosaniline or brilliant green from the Iodo-PUP column using CH3OH was examined at various flow rates (1–10 mL min−1). The chromatograms indicated that the dyes were completely eluted within the first 4–20 mL. The height equivalent to a theoretical plate (HETP) was calculated from the elution curves using the following equations: and (HETP = A + (B/U) + CU) [Citation40–Citation42], where VR is the volume of the eluate at the maximum peak, W is the width of the peak, L is the length of the Iodo-PUP bed, U is the flow rate and A, B, and C are constants. The average HETP and N (number of theoretical plate) values were determined to be 0.41 cm and 180, respectively, at a flow rate of 4.7 mL min−1 for the elution of the dyes. The slight increases in the HETP values were observed as the flow rate increased. However, a relatively high flow rate was applied without any appreciable loss in column performance. The ideal flow rate for the eluted dye can be calculated from the following equation:
, where B represents the longitudinal or molecular diffusion of the sample components and C is the rate of mass transfer. The average U value obtained using this equation was 4.5 mL min−1. The average standard deviation (σ) of the Iodo-PUP column efficiency, which was calculated using H = σ2/L, was 0.53.
3.8 Breakthrough capacity
To determine the sorption capacity of iodo-PF, 0.2 g of Iodo-PUP that was placed in a column was saturated with dye under optimum conditions. From the breakthrough curves shown in , the saturation of each column was achieved after passage of 18–48 mL of 1 mg/100 mL of the studied dyes. The capacities of the column for rosaniline and brilliant green were estimated to be 0.64 and 0.19 mmol g−1, respectively. The difference in the sorption capacities of these basic dyes may be due to their different sizes. In comparison to the isotherm capacities of the studied dyes, the breakthrough capacities are large, which confirms that the dynamic technique is more efficient that the batch one. In addition, the capacities of Iodo-PUP for the studied dyes are quite reasonable compared to previously reported results.
The pHpZC value of Iodo-PUP was approximately 6.9 (). At a pH lower than the pHZPC, the surface of Iodo-PUP is positively charged, and at a pH greater than pHZPC, the surface of Iodo-PUP becomes negatively charged. At the pH decreased, the nitrogen atoms of the dye become protonated, which reduces the sorption of the dye. The maximum sorption was achieved at approximately pH 7–12. Therefore, the maximum sorption of the studied dyes onto Iodo-PUP occurs in an alkaline medium due to electrostatic interactions and the formation ion-association complexes.
The electronic spectra of Iodo-PUP were recorded using a thin layer method in H2O. The UV–Vis spectra of Iodo-PUP contained three peaks at 700, 425.5 and 281 nm due to the functional groups on Iodo-PUP. These values are higher than those for the corresponding absorption bands of Iodo-PUP:rosaniline (blue shift), which were observed at 547, 388.5 and 252.5 nm. These results indicate that the formation of a [Dye]-Iodo-PUP complex. The electronic spectra of [Dye]-Iodo-PUP contained two bands in the visible region at 547 and 388.5 nm, which correspond to a charge transfer band (MLCT).
4 Applications
The accuracy of the proposed procedure for removing brilliant green and rosaniline dyes from four environmental samples, including sea water (Arabian Gulf) industrial wastewater (wastewater of El-Dammam industrial city), laundry wastewater and sewage, using Iodo-PUP was examined.
A 25 mL aliquot of a water sample was spiked with 100–200 μg of brilliant green and rosaniline. Then, the solutions were shaken for 30 min, and the concentration of the remaining dye in the supernatant solution was determined. The obtained results are summarized in . The average values for the removal percentage of brilliant green and rosaniline from the water sample were 100% and 91%, respectively. The average value of RSD% was determined to be 6.6%, which is considered relevant (less than 10%) for real samples.
Table 6 Removal of brilliant green and rosaniline from wastewater and sea water using batch technique.
The brilliant green and rosaniline dyes were removed from laundry wastewater using the column technique. A 100 mL aliquot of a water sample was spiked with 100 μg of the studied dye. Then, the solutions were passed through the Iodo-PUP column at a flow rate of 4–5 mL min−1. The average removal percentages of the dye from the laundry and sewage water samples using the Iodo-PUP column were 63.9% and 85.9%, respectively (, RSD% = 4.03). The results indicated that Iodo-PUP is a suitable sorbent for the removal of basic dyes from environmental samples.
Table 7 Removal of brilliant green and rosaniline from wastewater and sewage water using dynamic technique.
5 Conclusions
Iodo-polyurethane powder was successfully synthesized by replacing the amino group of PUF with an iodo functional group. The prepared sorbent exhibited good characteristics including fast extraction kinetics, low price, good chemical stability and reusability without loss of uptake capacity. Iodo-PUP can be potentially used as a solid-phase extraction material for the preconcentration, separation and removal of basic dyes from wastewater. The experimental data obtained for Iodo-PUP exhibited a better fit to the Langmuir isotherm model, which indicates that Iodo-PUP has homogeneous adsorption sites. The negative ΔG value was due to the spontaneous nature of the sorption process. We proposed that the sorption of basic dyes onto Iodo-PUP may proceed via ion-association complexes and an ion-exchange mechanism. Based on this study, Iodo-PUP has the ability to remove the basic dyes from wastewater.
Notes
Peer review under responsibility of Taibah University.
References
- E.A.MoawedA.B.AbulkibashM.F.El-ShahatSynthesis, characterization of iodo polyurethane foam and its application in removing of aniline blue and crystal violet from laundry wastewaterJ. Taibah Univ. Sci.920158088
- M.A.AhmadN.A.A.PuadO.S.BelloKinetic, equilibrium and thermodynamic studies of synthetic dye removal using pomegranate peel activated carbon prepared by microwave-induced KOH activationWater Resour. Ind.620141835
- C.I.PearceJ.R.LloydJ.T.GuthrieThe removal of colour from textile wastewater using whole bacterial cells: a reviewDyes Pigments582003179196
- D.ShenJ.FanW.ZhouB.GaoQ.YueQ.KangAdsorption kinetics and isotherm of anionic dyes onto organo-bentonite from single and multisolute systemsJ. Hazard. Mater.172200999107
- A.AppusamyI.JohnK.PonnusamyA.RamalingamRemoval of crystal violet dye from aqueous solution using triton X-114 surfactant via cloud point extractionEng. Sci. Technol. Int. J.172014137144
- A.SarıM.TuzenBiosorption of total chromium from aqueous solution by red algae (Ceramium virgatum): equilibrium, kinetic and thermodynamic studiesJ. Hazard. Mater.1602008349355
- A.SariD.MendilM.TuzenM.SoylakBiosorption of Cd(II) and Cr(III) from aqueous solution by moss (Hylocomium splendens) biomass: equilibrium, kinetic and thermodynamic studiesChem. Eng. J.144200819
- O.D.UluozluA.SariM.TuzenM.SoylakBiosorption of Pb(II) and Cr(III) from aqueous solution by lichen (Parmelina tiliaceae) biomassBioresour. Technol.99200829722980
- G.Z.KyzasP.I.SiafakaE.G.PavlidouK.J.ChrissafisD.N.BikiarisSynthesis and adsorption application of succinyl-grafted chitosan for the simultaneous removal of zinc and cationic dye from binary hazardous mixturesChem. Eng. J.2592015438448
- S.ZengS.DuanR.TangL.LiC.LiuD.SunMagnetically separable Ni0.6Fe2.4O4 nanoparticles as an effective adsorbent for dye removal: synthesis and study on the kinetic and thermodynamic behaviors for dye adsorptionChem. Eng. J.2582014218228
- Z.M.MagriotisM.Z.CarvalhoP.F.de SalesF.C.AlvesR.F.ResendeA.A.SaczkCastor bean (Ricinus communis L.) presscake from biodiesel production: an efficient low cost adsorbent for removal of textile dyeJ. Environ. Chem. Eng.2201417311740
- Z.M.MagriotisS.S.VieiraA.A.SaczkN.A.V.SantosN.R.StradiottoRemoval of dyes by lignocellulose adsorbents originating from biodiesel productionJ. Environ. Chem. Eng.2201421992210
- S.SadafH.N.BhattiS.NausheenS.NoreenPotential use of low-cost lignocellulosic waste for the removal of direct Violet 51 from aqueous solution: equilibrium and breakthrough studiesArch. Environ. Contam. Toxicol.662014557571
- S.NawazH.N.BhattiT.H.BokhariS.SadafRemoval of Novacron Golden Yellow dye from aqueous solutions by low-cost agricultural waste: batch and fixed bed studyChem. Ecol.3020145265
- S.NoreenH.N.BhattiS.NausheenS.M.SadafAshfaq batch and fixed bed adsorption study for the removal of Drimarine Black CL-B dye from aqueous solution using a lignocellulosic waste: a cost affective adsorbentInd. Crops Prod.502013568579
- S.SadafH.N.BhattiBatch and fixed bed column studies for the removal of Indosol Yellow BG dye by peanut huskJ. Taiwan Inst. Chem. Eng.452014541553
- S.NoreenH.N.BhattiFitting of equilibrium and kinetic data for the removal of Novacron Orange P-2R by sugarcane bagasseJ. Ind. Eng. Chem.20201416841692
- S.SadafH.N.BhattiEvaluation of peanut husk as a novel, low cost biosorbent for the removal of Indosol Orange RSN dye from aqueous solutions: batch and fixed bed studiesClean Technol. Environ. Policy162014527544
- N.M.MahmoodiU.SadeghiA.MalekiB.HayatiF.NajafiSynthesis of cationic polymeric adsorbent and dye removal isotherm, kinetic and thermodynamicJ. Ind. Eng. Chem.20201427452753
- A.HassaniL.AlidokhtA.R.KhataeeS.KaracOptimization of comparative removal of two structurally different basic dyes using coal as a low-cost and available adsorbentJ. Taiwan Inst. Chem. Eng.45201415971607
- L.A.Ramirez-MontoyaV.Hernandez-MontoyaM.A.Montes-MoranOptimizing the preparation of carbonaceous adsorbents for the selective removal of textile dyes by using Taguchi methodologyJ. Anal. Appl. Pyrolysis1092014920
- E.A.MoawedEffect of heating processes on Salvadora persica (Miswak) and its application for removal and determination of aniline blue from wastewaterJ. Taibah Univ. Sci.720132634
- E.A.MoawedI.IshaqA.Abdul-RahmanM.F.El-ShahatSynthesis, characterization of carbon polyurethane powder and its application for separation and spectrophotometric determination of platinum in pharmaceutical and ore samplesTalanta1212014113121
- E.A.MoawedM.F.El-ShahatSynthesis, characterization of low density polyhydroxy polyurethane foam and its application for separation and determination of gold in water and ores samplesAnal. Chim. Acta7882013200207
- E.A.MoawedA.B.FaragM.F.El-ShahatSeparation and determination of some trivalent metal ions using rhodamine B grafted polyurethane foamJ. Saudi Chem. Soc.1720134752
- P.FongA.ChowExtraction of aromatic acid and phenols by polyurethane foamTalanta391992497503
- K.RzeszutekA.ChowExtraction of phenols using polyurethane membraneTalanta461998507519
- E.A.MoawedM.F.El-ShahatExtraction of triazine herbicides by polyhydroxyl-polyurethane foam in environmental samplesJ. Chromatogr. Sci.5220141218
- S.M.Abdel AzeemH.M.KussM.F.El-ShahatCationic polyelectrolyte copolymer modified polyurethane foam for flow injection preconcentration and separation of trace amounts of β-lactam antibioticsTalanta972012513520
- E.A.MoawedY.AlqarniDetermination of azine and triphenyl methane dye in wastewater using polyurethane foam functionalized with tannic acidSample Prep.120131827
- R.A.AnayurtA.SariM.TuzenEquilibrium, thermodynamic and kinetic studies on biosorption of Pb(II) and Cd(II) from aqueous solution by macrofungus (Lactarius scrobiculatus) biomassChem. Eng. J.1512009255261
- A.SarıM.TuzenKinetic and equilibrium studies of biosorption of Pb(II) and Cd(II) from aqueous solution by macrofungus (Amanita rubescens) biomassJ. Hazard. Mater.164200910041011
- X.YangB.Al-DuriKinetic modeling of liquid-phase adsorption of reactive dyes on activated carbonJ. Colloid Interface Sci.28720052534
- M.A.K.M.HanafiahW.S.W.NgahS.H.ZolkaflyL.C.TeongZ.A.Abdul-MajidAcid Blue 25 adsorption on base treated Shorea dasyphylla sawdust: kinetic, isotherm, thermodynamic and spectroscopic analysisJ. Environ. Sci.2422012261268
- E.A.AbdullahA.AbdullahZ.ZainalM.Z.HusseinT.K.BanTiO2/Ag modified penta-bismuth hepta-oxide nitrate and its adsorption performance for azo dye removalJ. Environ. Sci.2410201218761884
- V.K.GuptaI.AliV.K.SainiAdsorption studies on the removal of Vertigo Blue 49 and Orange DNA13 from aqueous solutions using carbon slurry developed from a waste materialJ. Colloid Interface Sci.31520078793
- V.K.GuptaB.GuptaA.RastogiS.AgarwalA.NayakA comparative investigation on adsorption performances of mesoporous activated carbon prepared from waste rubber tire and activated carbon for a hazardous azo dye—Acid Blue 113J. Hazard. Mater.1862011891901
- D.RanjanM.TalatS.H.HasanBiosorption of arsenic from aqueous solution using agricultural residue ‘rice polish’J. Hazard. Mater.166200910501059
- O.AksakalH.UcunEquilibrium, kinetic and thermodynamic studies of the biosorption of textile dye (Reactive Red 195) onto Pinus sylvestris L.J. Hazard. Mater.1812010666672
- E.A.MoawedPreparation of novel ion exchange polyurethane foam and its application for separation and determination of palladium in environmental samplesAnal. Chim. Acta8502006263270
- M.F.El-ShahatE.A.MoawedA.B.FaragChemical enrichment and separation of uranyl ions in aqueous media using novel polyurethane foam chemically grafted with different basic dyestuff sorbentsTalanta712007236241
- E.A.MoawedN.BurhamM.F.El-ShahatSelective separation and determination of copper and gold in gold alloy using ion exchange polyurethane foamJ. Liq. Chromatogr. Relat. Technol.30200719031914