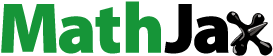
Abstract
The current study focused on the equilibrium, kinetics, and thermodynamics of Basic Fuchsin dye adsorption from aqueous solution using mussel shells as an adsorbent. Optimum adsorption conditions were identified by varying the solution pH, adsorbent dose, initial dye concentration, and contact time. Equilibrium data were fitted by the Langmuir, Freundlich and Dubinin–Radushkevich isotherm models, and a pseudo-second-order model best described the kinetics. Thermodynamic data showed that Basic Fuchsin dye adsorption onto mussel shells was a feasible, spontaneous and endothermic process. Statistical analysis was performed using the Chi-square (χ2) and mean square error (MSE) test methods to evaluate the best fit of the model to the experimental data. The adsorption of Basic Fuchsin by calcined mussel shells indicates their potential application as an adsorbent for the removal of dyes from aqueous solutions.
1 Introduction
Adsorption is one of the most common methods for the removal dyes from wastewaters. The environment is contaminated by many hazardous chemical species, especially with chemical dyes. Environmental protection requires conscientiousness regarding the use of dyes with respect to regulations and the treatment of effluent discharge because many industries use chemical dyes to treat their finished products. Chemical dyes reduce the light penetration in water, interfering with photosynthesis, and most of them contain suspected carcinogens [Citation1,Citation2]. Therefore, it is necessary to reduce or eliminate these life-threatening compounds from wastewater before it is discharged.
Basic Fuchsin dye belongs to the triarylmethane class dyes, which is inflammable in nature and possesses anaesthetic, bactericidal, and fungicidal properties. It is widely used as a colouring agent for textile and leather materials and in the staining of collagen, muscle, mitochondria, and tubercle bacillus. Due to its properties of poor biodegradation, toxicity, carcinogenicity and unsightliness, the removal of Basic Fuchsin from wastewater systems is of great concern and should be tested and implemented promptly.
The literature is rich with techniques and procedures for removing chemical dyes from aqueous media. Various authors have evaluated supplies of animal or vegetable origin [Citation3–Citation7] for the decontamination of aqueous media by chemical dyes.
In recent years, developing economical adsorbents to treat dyes in wastewater has attracted great interest. The application of inexpensive adsorbents for dye removal has been reviewed, and many non-conventional, low-cost adsorbents have been reported. In our ongoing research programme, we have focused on describing new alternative methods for the best removal of chemical dyes from aqueous media using low-cost and efficient adsorbents.
In this study, the removal of Basic Fuchsin dye from aqueous solutions onto calcined mussel shells was investigated. The effects of different parameters such as pH, contact time, adsorbent dose, initial dye concentration and temperature were investigated. The removal rate kinetics, thermodynamics and isotherms for Basic Fuchsin adsorption onto calcined mussel shells were also studied, and the characterization of calcined mussel shells as an adsorbent is presented.
2 Materials and methods
The mussel shells were collected and washed with tap water followed by distilled water, then dried at 378 K for 12 h. Mussel shells were powdered to small grains and then calcined at 1173 K for 2 h. The obtained residue was washed with distilled water and dried at 353 K for 24 h. The residue was finely chopped and ground into small particles of sizes in the range of 75–100 μm, milled in an agate mortar, washed with distilled water, dried overnight at 378 K, and then calcined at a heating rate of 2 K/min to 673 K and maintained at this temperature for 4 h. The resulting calcined mussel shell material (CMS) was stored in a glass bottle for further use.
The CMS adsorbent was characterized using elemental analysis, FT-IR and XRD. FT-IR spectra were obtained using an ATI Mattson Genesis series FTIR™ UNICAM instrument. XRD of CMS adsorbent was conducted using an X pert Pro X-ray diffractometer with a copper anticathode λ(Cu) = 1.5418 Å. The zero point charge pH of the CMS adsorbent was determined using the pH drift method as described in the reference [Citation8].
The chemical structure of Basic Fuchsin treated as cationic dye is shown in . Batch adsorption experiments were performed by shaking an appropriate weight of CMS adsorbent with 100 mL aqueous solution of Basic Fuchsin of known concentration in a series of 250 mL conical flasks placed in a temperature controlled shaking water bath at different concentrations (between 50 and 200 mg L−1), pH values (between 2 and 12), temperatures (between 298 and 428 K) and adsorbent doses (between 100 mg and 500 mg) at a constant shaking rate of 350 rpm. After the desired contact time, samples were withdrawn from the mixture using a micropipette and centrifuged for 5 min at 5000 rpm. After centrifugation, supernatants were analysed to determine the final concentration of Basic Fuchsin using a UV–vis spectrophotometer at a wavelength of 544 nm. The amount of equilibrium adsorption qe (mg g−1) was calculated using the formula(1)
(1) where Ce (mg L−1) is the concentration of Basic Fuchsin aqueous solution at the equilibrium state, C0 (mg L−1) is the initial concentration of Basic Fuchsin in aqueous solution, V is the volume of the solution (L) used in the experiments, and W is the weight of the CMS adsorbent (g). The Basic Fuchsin removal percentage was calculated from the following expression:
(2)
(2) where C0 is the initial Basic Fuchsin concentration (mg L−1) and Ce is the concentration of Basic Fuchsin (mg L−1) at equilibrium.
For statistical analysis, the tests were performed in duplicate to ensure the reliability and reproducibility of the results obtained, and the data were reported as the mean ± SD. The model parameters and constants were analysed by linear regression using Excel 2010. In addition to the correlation coefficient (r2), the Chi-square (χ2), the mean square error (MSE) and the validation by normalized standard deviation Δq (%) test methods were also used to evaluate the best fit of the model to the experimental data using Eqs. (3), Equation(4)(3)
(3) and Equation(5)
(4)
(4) , respectively.
(3)
(3)
(4)
(4)
(5)
(5) where n is the number of data points, qe,exp is the observation from the experiment, and qe,cal is the calculation from the models. Smaller values correspond to better curve fitting.
3 Results and discussion
3.1 Characterization
The results of elemental analysis show that the CMS adsorbent contains significant quantities of Ca (60.24%) and Si (3.57%) and lower amounts of other species such as Mg (0.90%), Al (0.41%), P (0.20%) and Sr (0.11%).
The analysis by XRD depicted in showed the presence of calcite and portlandite, syn. The FT-IR analysis shows functional groups existing in the CMS adsorbent (). The bands at 3643 cm−1 are assigned to hydroxy group stretching modes, and the stretching and folding of the carbonate group are assigned to the bands at 1437 cm−1 and 874 cm−1. The SEM image shown in shows that the CMS adsorbent particles have irregular forms and different sizes. We see also that the surface morphology is not homogenous, with the existence of some pores.
3.2 Effect of adsorbent dose
The effect of adsorbent dose on the removal % of Basic Fuchsin dye from aqueous solutions onto CMS adsorbent was investigated by contacting 100 mL of 60 mg L−1 dye solution at room temperature (20 °C) for 240 min at a constant stirring speed of 350 rpm. Different amounts of CMS (100–500 mg) were tested for this study. depicts the variation of removal % versus adsorbent dose. In light of these results, we note that increasing amounts of CMS adsorbent increase the removal % of Basic Fuchsin. This behaviour is related to the increased number of sites available on the CMS adsorbent for dye adsorption. This phenomenon was already described in our previous works on dye removal with calcined bones as the adsorbent [Citation9]. For the following experiments, we used 500 mg of CMS per 100 mL of Basic Fuchsin solution.
3.3 Effect of pH
The pH values of the solution are an important parameter during the adsorption processes, and the initial pH value of the solution has greater influence than the final pH [Citation10,Citation11]. In this case, shows the variation of removal % of Basic Fuchsin from aqueous solutions versus pH from 2 to 12. It is very important to determine the pH of the zero point charge of the CMS, which is equal to 9.2, as depicted in . For pH values higher than 9.2, the surface of the CMS adsorbent becomes negatively charged, while it is in the opposite state for pH < 9.2. shows that the removal % of Basic Fuchsin onto CMS is highest for pHZPC above 9.2, which indicates that the negative form of CMS is responsible for adsorption in this range. For acidic pH, increasing the value increases the removal efficiency of Basic Fuchsin. The lowest removal % at acidic pH is due to the repulsive electrostatic forces between the positive charged surface of CMS and the cationic dye. The removal % of Basic Fuchsin is maximal for pHZPC, at 90%.
3.4 Effect of initial dye concentration and contact time
shows the effect of the Basic Fuchsin solution concentration on adsorption onto the CMS adsorbent. In these experiments, the amount of CMS was constant (500 mg of CMS in 100 mL of dye solution), while the dye concentration was varied from 50 to 200 mg L−1 with different time intervals. By plotting the removal % versus dye concentration and contact time, it can be seen that the removal % of the dye increases with increasing concentration and contact time. The maximum adsorption of Basic Fuchsin is observed at 60 min for all dye concentrations. Thus, there is almost no further increase in the adsorption, and the equilibrium contact time is fixed. At this time, removal % increases from 72.45% to 91.2% when the concentration of the dye is changed from 50 mg L−1 to 200 mg L−1.
Fig. 8 Effect of initial dye concentration on the removal % of Basic Fuchsin, adsorbent dose 500 mg, temperature 25 °C.
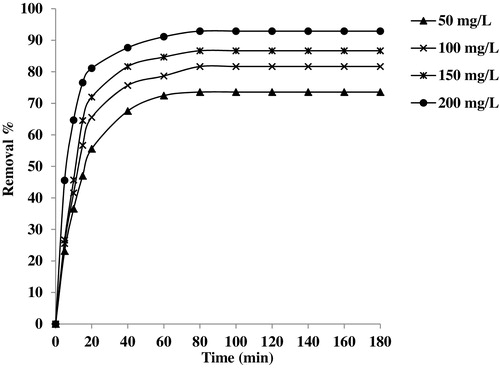
At higher concentration, the ratio of the initial number of Basic Fuchsin molecules to the available surface area is high, and the fractional adsorption subsequently becomes dependent on the initial concentration. However, at lower concentration, the available sites of adsorption are fewer, and hence the dye removal depends upon concentration. In light of these results, it appears that for the first 40 min, the adsorption uptake is rapid, after which it proceeds at a slower adsorption rate and finally attains saturation at 60 min. The obtained removal curves were single, smooth and continuous, indicating monolayer coverage of the dye on the surface of the CMS adsorbent.
3.5 Adsorption kinetics
The adsorption kinetic experimental data were fitted with the following models. The pseudo-first-order model is expressed by the following linearized form [Citation12]:(6)
(6) where qe (mg g−1) and qt (mg g−1) are the amount of adsorbed dye per gram of CMS adsorbent at equilibrium and at time t, respectively, and k1 (min−1) is the pseudo-first-order rate constant.
Hence, a linear trace is expected between the two parameters, log (qe − qt) and t, if the adsorption follows first-order kinetics. The values of k1 and qe can be determined from the slope and intercept.
The pseudo-second-order model proposed by Ho and McKay [Citation13] is based on the assumption that the adsorption follows second-order chemisorption. The linearized form of the pseudo-second-order model is as follows:(7)
(7) where k2 is the pseudo-second-order rate constant (g mg−1 min−1).
The experimental and calculated (q) values on the kinetic fitting curves can be compared to establish the adapted kinetics model. In addition, the correlation coefficients (r2) obtained were used to validate the best kinetics model and describe the adsorption kinetics.
The parameters of the pseudo-first-order and pseudo-second-order kinetic models were estimated by linear regression. The resulting data and the correlation coefficients (r2) are given in . From these results, the correlation coefficients for the pseudo-first-order model of Basic Fuchsin adsorption were low, and the values of χ2 and MSE were high. The theoretical qe did not give acceptable values compared to the experimental ones.
Table 1 Kinetic parameters for the adsorption of Basic Fuchsin onto CMS adsorbent.
A plot of t/qt and t should give a linear relationship if the adsorption follows the pseudo-second-order model. The qe and k2 can be calculated from the slope and intercept of the plot obtained in . All the determined model parameters and constants with the statistical analysis values are given in . The calculated values for the pseudo-first-order model are not well fitted with the experimental data, based on the low correlation coefficient r2 and the high values of χ2, MSE and Δq (%). Thus, the adsorption of Basic Fuchsin onto CMS does not follow the pseudo-first-order model. In contrast to the first model, we found a high correlation coefficient r2 (very close to unity) as well as small χ2 and MSE values for the pseudo-second-order model, indicating that the adsorption process of Basic Fuchsin onto CMS obeys pseudo-second-order model kinetics at all initial dye concentrations. The values of Δq (%) are still high, but less so. The rapid uptake of Basic Fuchsin indicates that the rate-determining step could be chemisorption, as described by many researchers [Citation14,Citation15].
The intraparticle diffusion model indicates that the rate-limiting step is the transport of the solute from the bulk of solution into adsorbent pores through an intraparticle process [Citation16]. The equation is as follows:(8)
(8) where kid is the intraparticle diffusion rate constant (mg g−1 min1/2), and Ci is the thickness of the boundary layer.
It should be noted that the intraparticle diffusion model also demonstrated a high correlation coefficient r2 value as well as small χ2 and MSE values, indicating that pore diffusion also affects the rate of Basic Fuchsin adsorption. However, the y-intercept Ci is not equal to zero, which implies that this mechanism does not solely limit the overall adsorption process. The rate-limiting step may be a complex combination of chemisorption and intraparticle transport [Citation17].
3.6 Adsorption isotherms
The adsorption isotherm is a graphical representation of the relationship between the amount adsorbed by a unit weight of adsorbent and the amount of adsorbate remaining in the test medium at constant temperature under equilibrium conditions. The isotherm models used to analyse the experimental equilibrium data are as follows:
• | Langmuir isotherm: The Langmuir isotherm [Citation18] assumes that monolayer adsorption occur at bindings sites with homogenous energy levels, with no interactions between adsorbed molecules and no transmigration of adsorbed molecules on the adsorption surface. The linear Langmuir equation is given as Eq. Equation(9) |
• | Dubinin–Radushkevich isotherm: The Dubinin–Radushkevich (D–R) isotherm model [Citation19] focuses on the heterogeneity of the surface energies and has the following expression: |
• | Freundlich isotherm: The Freundlich model [Citation21] is an empirical equation based on sorption on a heterogeneous surface or surface supporting sites of varied affinities. The logarithmic from of Freundlich is given by the following equation: |
In this investigation, the Freundlich, the Langmuir, and the Dubinin–Radushkevich (D–R) models were used to describe the equilibrium data acquired at different temperatures. The results are shown in .
Table 2 Isotherms parameters for the adsorption of Basic Fuchsin onto CMS adsorbent.
The Langmuir isotherm constants KL and qm were calculated from the slope and intercept of the plot between Ce/qe and Ce. The isotherm showed a good fit to the experimental data with high correlation coefficients at all temperatures, as shown in . The maximum dye adsorption capacity by CMS was found to be 141.65 mg g−1 at 298 K. The values of KL decreased with increasing temperature, indicating that increasing temperature induced a lower maximum adsorption capacity.
To distinguish between physical and chemical adsorption on the heterogeneous surfaces, the equilibrium data were tested with the D–R isotherm model. The plots between Ln(qe) and ɛ2 gave straight lines at all temperatures; the values of constants qm and β thus obtained are given in . The estimated values of E for this study were found in the range expected for chemical adsorption, as shown in . Thus, the adsorption of Basic Fuchsin on the surface of CMS is chemical in nature.
Table 3 Thermodynamic parameters for the adsorption of Basic Fuchsin onto CMS.
The Freundlich constants Kf and 1/n were calculated from the intercept and slope of the straight line of the plot log(qe) versus log(Ce). shows that the adsorption capacity Kf decreased with increasing temperature. The magnitude of n gives a measure of the favourability of adsorption. Values of n between 1 and 10 (1/n less than 1) represent favourable adsorption. For this study, the value of n presented the same trend, indicating a favourable adsorption.
By comparing the values of r2, χ2 and MSE for the three isotherms listed in , it could be concluded that the adsorption of Basic Fuchsin onto CMS was best fitted to the Langmuir isotherm equation in the temperature range studied. The fit of the adsorption data to the Langmuir isotherm implied that the binding energy on the whole surface of the adsorbent was uniform. It also indicated that the adsorbed dye molecules did not interact or compete with each other and were adsorbed by forming a monolayer.
3.7 Adsorption thermodynamic
The spontaneity of the adsorption process is determined by the crucial thermodynamic parameters. The standard Gibbs free energy changes (ΔG°), standard enthalpy changes (ΔH°), and standard entropy changes (ΔS°) can be calculated from the following equations:(15)
(15)
(16)
(16) where ΔG° is the free energy change (kJ mol−1), R is the universal gas constant (8.314 J mol−1 K−1), K0 is the thermodynamic equilibrium constant, and T is the absolute temperature (K). Values of K0 may be calculated from the relation Ln(qe/Ce) versus qe at different temperatures, extrapolating to zero.
The thermodynamic parameters are listed in . The negative ΔG° values (−1.444 kJ mol−1 to −2.217 kJ mol−1) indicate the spontaneity and feasibility of the adsorption process of Basic Fuchsin onto CMS. The adsorption is chemical in nature, indicated by the increase in ΔG° values when the temperature was raised from 298 to 328 K. The same results are obtained in similar studies [Citation22,Citation23]. ΔH° and ΔS° were then estimated from the slope and intercept of the plot of Ln K0 versus 1/T based on Eq. Equation(15)(15)
(15) . The data are presented in . The positive value of ΔH° (5.247 kJ mol−1) suggests the endothermic nature of the adsorption process, while the positive ΔS° (22.45 J mol−1 K−1) value suggests the increase in adsorbate concentration in the solid–liquid interface, thereby indicating the decrease in adsorbate concentration onto the solid phase. It also confirms the increased randomness at the solid–liquid interface during adsorption. This result is a normal consequence of the chemical adsorption phenomenon, which occurs through electrostatic interactions.
3.8 Comparison of the adsorption capacities of certain adsorbents for the removal of dyes from aqueous media
depicts the values of adsorption capacities of various marine-origin adsorbents. CMS can be classified as a good adsorbent compared with other examples, with an adsorption capacity of 141.65 mg g−1.
Table 4 Comparison of the adsorption capacities of some adsorbents for removal dyes from aqueous media.
3.9 Reusability of CMS adsorbent
The stability and regeneration ability of the adsorbent during the adsorption process are essential to its practical application. Interestingly, the CMS adsorbent could be regenerated by simple calcination at 500 °C in air for 60 min. The calcination of the adsorbent could retain the high removal efficiency during five successive cycles. To achieve this effect, we examined studied the variation of adsorption capacity versus cycle number. As depicted in , the adsorption capacities decrease for each new cycle after regeneration. The original adsorption capacity of CMS for BB41 is 167.68 mg g−1. After five cycles, the adsorption capacity of CMS for BB41 drops to 10.12 mg g−1. Therefore, CMS shows excellent adsorption performance and regeneration, and its use can be extended to environmental applications for wastewater treatment.
4 Conclusion
The ability of calcined mussel shells as an adsorbent to remove Basic Fuchsin from aqueous solutions was investigated. Operational parameters such as pH, initial dye concentration, adsorbent dose, contact time, and temperature were found to affect the adsorption efficiency of calcined mussel shells. The kinetic data show that the adsorption process follows a pseudo-second-order model, indicating chemisorption. The same result was found by the D–R isotherm model, and the adsorption data present the best fit to the Langmuir model, suggesting that adsorption occurs by the formation of a monolayer. The thermodynamic parameters show that adsorption is spontaneous and endothermic in nature and increased randomness at the solid–liquid interface, overall showing the feasibility of the process. This study showed that calcined mussel shells could be used as a good and inexpensive adsorbent for dye effluent treatment.
Notes
Peer review under responsibility of Taibah University
References
- D.BrownEffects of colorants in the aquatic environmentEcotoxicol. Environ. Saf.1321987139147
- M.La FarreS.PerezL.KantianiD.BarceloFate and toxicity of emerging pollutants, their metabolites and transformation products in the aquatic environmentTrends Anal. Chem.2720089911007
- S.NoreenH.N.BhattiS.NausheenS.SadafM.AshfaqBatch and fixed bed adsorption study for the removal of Drimarine Black CL-B dye from aqueous solution using a lignocellulosic waste: a cost affective adsorbentInd. Crops Prod.502013568579
- G.Z.KyzasK.A.MatisNano adsorbents for pollutants removal: a reviewJ. Mol. Liq.2032015159168
- O.DumanS.TunçT.G.PolatAdsorptive removal of triarylmethane dye (Basic Red 9) from aqueous solution by sepiolite as effective and low-cost adsorbentMicroporous Mesoporous Mater.2102015176184
- M.RafatullahO.SulaimanR.HashimA.AhmadAdsorption of methylene blue on low-cost adsorbents: a reviewJ. Hazard. Mater.1771-320107080
- B.H.HameedA.T.DinA.L.AhmadAdsorption of methylene blue onto bamboo-based activated carbon: kinetics and equilibrium studiesJ. Hazard. Mater.14132007819825
- M.GhaediS.HajatiB.BarazeshF.KarimiG.GhezelbashSaccharomyces cerevisiae for the biosorption of basic dyes from binary component systems and the high order derivative spectrophotometric method for simultaneous analysis of Brilliant green and Methylene blueJ. Ind. Eng. Chem.192013227233
- M.El HaddadR.SlimaniR.MamouniR.LaamariS.RafqahS.LazarEvaluation of potential capability of calcined bones on the biosorption removal efficiency of safranin as cationic dye from aqueous solutionsJ. Taiwan Inst. Chem. Eng.4420131318
- F.KooliL.YanR.Al-FazeA.Al-SehimiRemoval enhancement of basic blue 41 by brick waste from an aqueous solutionArab. J. Chem.82015333342
- M.El HaddadR.MamouniN.SaffajS.LazarRemoval of a cationic dye – Basic Red 12 – from aqueous solution by adsorption onto animal bone mealJ. Assoc. Arab Univ. Basc Appl. Sci.1220124854
- S.LagergrenAbout the theory of so-called adsorption of soluble substanceK. Seven Vet. Akad. Handl.241898139
- Y.S.HoG.McKayPseudo-second order model for sorption processesProcedia Biochem.3451999451465
- K.S.LowC.K.LeeK.K.TanBiosorption of basic dyes by water hyacinth rootsBioresour. Technol.5219957983
- X.J.XiongX.J.MengT.L.ZhengBiosorption of C.I. Direct Blue 199 from aqueous solution by nonviable Aspergillus nigerJ. Hazard. Mater.1752010241246
- B.YaseminT.ZekiRemoval of heavy metals from aqueous solution by sawdust adsorptionJ. Environ. Sci.192007160167
- G.CunhaL.C.RomaoM.C.SantosB.R.AraujoS.NavickieneV.L.PaduaAdsorption of trihalomethanes by humin: batch and fixed bed column studiesBioresour. Technol.101201033453350
- I.LangmuirThe constitution and fundamental properties of solids and liquidsJ. Am. Soc.3811191622212295
- M.M.DubininL.V.RadushkevichThe equation of the characteristic curve of the activated charcoalUSSR Phys. Chem. Sect.551947331337
- S.KunduA.K.GuptaArsenic adsorption onto iron oxide-coated cement (IOCC): regression analysis of equilibrium data with several isotherm models and their optimizationChem. Eng. J.122200693106
- H.M.F.FreundlichOver the adsorption in solutionJ. Phys. Chem.57A1906385470
- S.KaurS.RaniR.K.MahajanM.AsifV.K.GuptaSynthesis and adsorption properties of mesoporous material for the removal of dye safranin: kinetics, equilibrium, and thermodynamicsJ. Ind. Eng. Chem.2220151927
- N.F.CardosoE.C.LimaB.RoyerM.V.BachG.L.DottoL.A.A.PintoT.CalveteComparison of Spirulina platensis microalgae and commercial activated carbon as adsorbents for the removal of reactive Red 120 dye from aqueous effluentsJ. Hazard. Mater.241–2422012146153
- N.A.OladojaI.O.RajiS.E.OlaseniT.D.OnimisiIn situ hybridization of waste dyes into growing particles of calcium derivatives synthesized from a Gastropod shell (Achatina Achatina)Chem. Eng. J.1712011941950
- A.EsmaeliM.JokarM.KoushaE.DaneshvarH.ZiloueiK.KarimiAcidic dye wastewater treatment onto a marine macroalga, Nizamuddina zanardini (Phylum: Ochrophyta)Chem. Eng. J.2172013329336
- Y.KhambhatyK.ModyS.BashaEfficient removal of Brilliant Blue G (BBG) from aqueous solutions by marine Aspergillus wentii: kinetics, equilibrium and process designEcol. Eng.4120127483
- B.XinY.ZhangC.LiuS.ChenB.WuComparison of specific adsorption capacity of different forms of fungal pellets for removal of Acid Brilliant Red B from aqueous solution and mechanisms explorationProcess Biochem.47201211971201