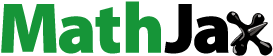
Abstract
The gram-positive bacterium Rhodococcus equi was isolated from fertile soil, and mineral salt media (MM) and trace elements were used to provide the necessary elements for its growth and PHB production in addition to using crude palm kernel oil (CPKO) 1% as the carbon source. Gas chromatography (GC) demonstrated that the composition of the recovered biopolymer was homopolymer polyhydroxybutyrate (PHB). The strain of the present study has a dry biomass of 1.43 (g/l) with 38% PHB, as determined by GC. The recovered PHB was characterized by NMR to study the chemical structure. In addition, DSC and TGA were used to study the thermal properties of the recovered polymer, where the melting temperature (Tm) was 173 °C, the glass transition temperature (Tg) was 2.79 °C, and the decomposition temperature (Td) was 276 °C. Gel permeation chromatography (GPC) was used to study the molecular mass of the recovered PHB in addition to comparing the results with other studies using different bacteria and substrates, where the molecular weight was 642 kDa, to enable its usage in many applications. The present study demonstrated the use of an inexpensive substrate for PHB production, i.e., using gram-positive bacteria to produce PHB polymer with characterization.
1 Introduction
Polyhydroxyalkanoates (PHAs) are a class of natural, biodegradable and environmentally friendly polymers that are produced from biorenewable sources [Citation1]. PHAs are storage compounds that are naturally accumulated in the bacterial cytoplasm for carbon and energy under limited nutrient conditions [Citation2]. Approximately 150 types of monomers have been distinguished as PHA constituents, which are delivered via different metabolic pathways using different carbon sources [Citation3]. Generally, PHAs are divided into three groups: short-chain-length PHA (SCL-PHA) has 3–5 carbon atoms such as 3-hydroxybutyrate (4C) and/or 3-hydroxyvalerate (5C), medium-chain-length PHA (MCL-PHA) has 6–14 carbon atoms such as 3-hydroxyhexanoate (6C), 3-hydroxyclanoate (8C), 3-hydroxydecanoate (10C), 3-hydroxydodecunoate (12C) and 3-hydroxytetradecanoate (14C), and long-chain-length (LCL-PHA) has 15 or more carbon atoms [Citation4]. PHB is the most widely recognized PHA and was first found in microbes in 1925 by Lemoigne [Citation5]. PHB is a linear polyester of d(−)-3-hydroxybutyric acid, which is aggregated in intracellular granules by various gram-positive and gram-negative bacteria when the concentrations of nutrients, such as nitrogen, phosphorous or oxygen limit growth [Citation6,Citation7]. At least 75 different genera of bacteria have been known to accumulate PHB [Citation8], such as Ralstonia eutropha [Citation9], Pseudomonas putida [Citation10] and Bacillus subtilis [Citation11]. The limited studies about the production of PHA from Rhodococcus sp. by Hori et al. [Citation12] identified the ability of Rhodococcus aetherivorans IAR1 to produce PHBV from toluene without the supply of a secondary carbon source as the precursor. The indicated presence of PHA synthase genes in other Rhodococcus species, such as R. aetherivorans I24, which has two new PHA synthase genes, confirms the integration to R. eutropha [Citation13] and Rhodococcus opacus PD630, which was indicated with PHA synthase in the genome sequence [Citation14].
Plant oils, such as soybean oil, palm oil and corn oil, are attractive carbon sources for PHA production because they are generally less expensive than most sugar. More cell biomass and PHA production accumulate in the plant oil culture (0.6–0.8 g of PHA per g of oil) because they contain higher carbon content per weight than sugar [Citation15]. Two separate types of oils can be extracted from the oil palm fruit: crude palm oil (CPO) from the mesocarp and crude palm kernel oil (CPKO) from the kernel [Citation16]. The molecular weight of PHB differs according to the organism, growth condition and extraction method, and it can vary from 50,000 to over a million. Because of its important properties of thermoplasticity and biodegradability, this polymer has a considerable commercial interest [Citation7]. PHB is a highly crystalline thermoplastic polymer with a relative melting temperature of 170–180 °C and a glass transition temperature of 0–5 °C [Citation17]. The thermal properties of PHB can be improved by grafting suitable monomers onto PHB to increase the thermal stability of PHB without the conventional stabilizers or antioxidants, which are used to stabilize commodity thermoplastics [Citation18]. The thermal stability of PHB can be improved by grafting maleic acid (MA) to PHB using different methods, which significantly improves the crystallization and degradation behaviors of PHB [Citation19]. Another study uses a pomac extract (EP) to improve the thermal stability and mechanical properties of PHB [Citation20]. Many other reports are related to this field. This work studied the chemical structure of PHB and thermal properties by R. equi, whereas there are limited reports for its polymer synthesis using a potential substrate CPKO.
2 Materials and methods
2.1 Bacterial strain and culture media:
The bacterial strain in our study was R. equi, which was isolated from fertile soil sampled at Gunung Lang, Perak, Malaysia. The mineral salt medium (MM) and trace elements were used as described in [Citation21]. The MM was contained (g/l): 2.80 KH2PO4, 3.32 Na2HPO4, 0.25 MgSO4·7H2O, 0.50 NH4Cl, and CPKO (1%) as a carbon source for bacteria with 1 ml of trace element solution. The trace element solution contains (g/l): 20 FeCl3O·6H2O, 10 CaCl2·H2O, 0.03 CuSO4·5H2O, 0.05 MnCl2·4H2O and 0.10 g ZnSO4·7H2O. The trace element solution was sterilized by filtration; the MgSO4·H2O solution and CPKO were sterilized by autoclave and separately aseptically added to the culture medium (MM).
2.2 Production of PHB
PHB production was performed using one-stage cultivation; the bacterial cells were first grown in the tryptone soy broth to enrich and activate the cells. This step is referred as the inoculum preparation. Next, 50 ml of the MM in 250 ml flasks were inoculated with 3% of inoculums. This flask incubated in the shaker incubator for 48 h at 30 °C and 200 RPM. The cells were harvested and washed with hexane and subsequently distilled water. Next, they were frozen for 24 h before they were freeze-dried at −40 °C.
2.3 Determination of PHB content using gas chromatography (GC)
The PHB content was determined using GC-2010 (Shimadzu, Japan). Approximately 15–25 mg of freeze-dried cells were methanolysed by adding 2 ml of methanolysis solution, which was prepared as described by Kamilah et al. [Citation22], and 2 ml of chloroform. This mixture was subsequently heated at 100 °C for 140 min with gentle shaking every half hour. The reaction was allowed to cool. Then, 1 ml water was added; the mixture was vortexed for 1 min and subsequently allowed to separate to form two layers. The lower chloroform layer was recovered into a new tube, which contained anhydrous sodium sulfate to remove the moisture. Then, 0.5 ml of chloroform solution was mixed with 0.5 ml of caprylate methyl ester in a GC tube. The samples were analysed using GC, which was equipped with a fused silica column, and nitrogen was used as a gas carrier [Citation23]. The injection volume of converted hydroxyacyl methyl ester was 2.0 μL with an injector temperature of 270 °C.
2.4 Recovering of PHB
PHB polymer recovery was performed according to [Citation24]. The accumulated PHB granules in the cells were extracted by stirring lyophilised cells for 5 days in chloroform with a ratio of cells to chloroform of 1 g:100 ml. The digested cells were filtered using Whatman ® No. 1 filter paper to remove the cell debris and collect the chloroform extract. After filtration, the solution was concentrated to approximately 20 ml using an Eyela N-1000 rotary evaporator (Eyela, Japan). The concentrated polymer solution was precipitated by drop-wise addition into vigorously stirred chilled methanol to form a white material as a precipitate. The precipitated and purified polymer was later collected, air-dried and used for further characterization work.
2.5 Characterization PHB
2.5.1 Nuclear magnetic resonance (NMR)
The 1H NMR and 13C NMR analyses were performed using a Bruker Advance 500 NMR (Bruker Biospin Corporation, USA) spectrometer, which operated at 500 MHz, 25 °C. The polymer sample was dissolved in deuterated chloroform (CDCl3) (Sigma–Aldrich, USA) at a concentration of 25 mg/ml using tetramethysilane (Me4Si) as an internal chemical shift reference.
2.5.2 Differential scanning calorimetry analysis (DSC)
The thermal properties were measured using instrument Pyris 1 (Perkin Elmer, USA), which was equipped with cooling accessory. The instrument was equilibrated at room temperature prior to cooling at −40 °C at a cooling rate of −5 °C/min. Then, 5 mg of the polymer was encapsulated in an aluminium pan and maintained at approximately −40 °C for 1 min with subsequent heating to 200 °C at a heating rate of 5 °C/min. The second cooling and heating was similarly performed, except the heating began from −40 to 200 °C. The glass transition temperature (Tg) and melting temperature (Tm) were determined from the DSC thermogram.
2.5.3 Thermogravimetric analysis (TGA)
The thermal stability of the polymer was determined using instrument STA 6000 (Perkin Elmer, USA). Approximately 10 mg of the polymer sample was loaded into an aluminium pan and heated from 30 to 920 °C at a heating rate of 10 °C/min under nitrogen atmosphere. This analysis was used to measure the decomposition temperature (Td), which is defined as a temperature for 5% weight loss of the polymer sample.
2.5.4 Gel permeation chromatography analysis (GPC)
The molecular weights of the extracted and purified polymer were determined using an Agilent 1200 gel permeation chromatography (GPC) system, which was connected to a refractive index detector with Shodex K-806 (Agilent, USA) columns. The polymers were dissolved in HPLC-grade chloroform at a concentration of 1 mg/ml and filtered through 0.45 μm PTFE membrane. Chloroform was used as the eluent with a flow rate of 0.8 ml/min at 40 °C. Universal calibration was generated using a narrow polydispersity polystyrene standard (Agilent, USA).
3 Results and discussion
3.1 PHB production by R. equi
The production of PHB polymer was performed using a shaking flask with CPKO (1%) as the carbon source, which is considered the potential carbon feedstock for high cell density and PHA production that contains unsaturated fatty acids [Citation25]. The bottles with the harvested cells were weighed after lyophilisation to measure the CDW. The CDW was calculated as follows:
The strain yielded 1.43 (g/l) in dry biomass, which comprised of up to 38% of PHB as evaluated by GC. This result was close to the result for Rhodococcus Rubber, where fructose was used as the carbon source [Citation26]. CPKO has been reported in another study as a potential carbon source for P(3HB-co-3HHx) copolymer by recombinant Cupriavidus necator [Citation27]. In addition, Kek et al. [Citation28] recorded the ability of C. necator H16 and its mutant PHB–4 to synthesize PHB from CPKO.
3.2 Chemical structure
The pure extracted PHB polymer, which appeared whitish as in , was used to study the chemical structure using NMR analysis to determine the quality of the PHB structural composition. The obtained 1H NMR spectra from the PHB sample that was produced from R. equi in the presence of CPKO 1% as the carbon source are shown in . Both observed peaks in the spectra correspond to different types of carbon atoms in the PHB structure. The spectrum shows a doublet at 1.30 ppm, which is attributed to the methyl group that is coupled to one proton. The doublet of quadruplet at 2.57 ppm is attributed to the methylene group adjacent to an asymmetric carbon atom, which bears a single atom. The multiplet at 5.27 ppm is characteristic of a methylene group. Two other signals are observed: a broad one at 1.30 ppm because of water and another at 7.28 ppm, which is attributed to the solvent, i.e., chloroform. Identical observations were reported in Refs. [Citation29,Citation30].
The 13C NMR analysis also confirmed the structure of the extracted PHB polymer by R. equi (). The four peaks were assigned to the methyl (CH3) at 19.65 ppm, methylene (CH2) at 40.66 ppm, methane (CH) at 67.77 ppm and carbonyl (C=O) at 169.162 ppm. This chemical shift signals of the 13C NMR spectrum of recoverd PHB from R. equi was identical to the results of the commercial PHB [Citation31] as shown in .
Table 1 Chemical shift signals obtained from the 13C NMR spectra for the PHB sample, which are similar to the reported result of commercial PHB in [Citation31].
3.3 Thermal properties
and show the thermal properties for PHB, which were investigated using DSC and TGA. The results are listed in . The melting temperature (Tm) of the recovered PHB using CPKO as the carbon source is 173 °C, which is consistent with the publications [Citation32,Citation33]. The glass transition temperature (Tg), which is 2.79 °C, is in the mentioned range in a previous study [Citation34], The decomposition temperature (Td) is approximately to 276 °C. These values are consistent with the results of PHB produced by Bacillus sphaericus NII 0838 [Citation35], which is mainly associated with the ester cleavage of PHB component by the β-elimination reaction [Citation36]. The thermal properties of PHB in the present study are consistent with the results of PHB produced from C. necator cells when cooking oil is used as the carbon source [Citation37].
Fig. 4 Melting temperature (Tm) and glass transition temperature (Tg) of PHB produced from R. equi using CPKO 1% as the carbon source.
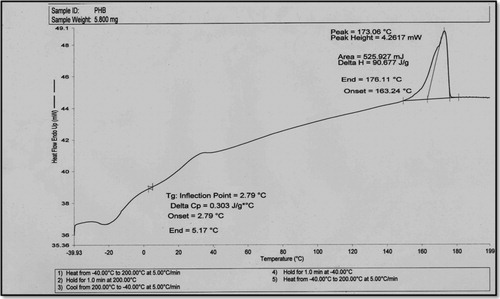
Fig. 5 Decomposition temperature (Td) of PHB produced from R. equi using CPKO 1% as the carbon source.
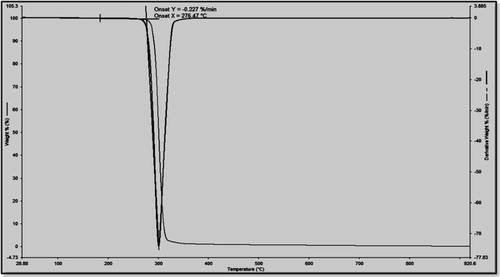
Table 2 Thermal properties of PHB produced from R. equi with CPKO 1% as the carbon source.
3.4 Molecular mass
Gel permeation chromatography (GPC) was used to estimate the molecular mass of the PHB sample because the molecular mass is an important factor to determine the physical properties of polymers [Citation38]. GPC was used to determine the molecular weight (), weight number average mass (
) and polydispersity (
) of polymer. The molecular mass, weight number average mass, and polydispersity of PHB recovered from R. equi cells are 642 kDa, 373 kDa, and 1.72, respectively. The GPC results for PHB polymer of R. equi are listed in in comparison with the results of other studies. The molecular mass of PHB in appears adversely affected by microbes, carbon sources, extraction methods and environmental conditions. This result is similar to the results of PHB polymer from marine Bacillus subtilis MSBN17 [Citation11], and the molecular weight is less than 10 × 105 Da, which qualifies it for commercial applications [Citation6]. Another study reported that the molecular weight of PHB polymer was affected by PHA synthase, and the high activity of PHA synthase decreased the molecular weight of the biopolymer [Citation39].
Table 3 Molecular weight of the recovered PHB in comparison with the results of other reports: weight average (), number average (
) and polydispersity (
) as determined using GPC.
4 Conclusions
This study has successfully revealed the ability of R. equi to produce PHB, which is considered a substitute for petroleum-based plastics in numerous applications. The substrate is an important factor in the production of microbial PHB in addition to other growth conditions, which involve the contents of medium and trace elements to add to the medium. CPKO was used as a potential substrate for the PHB biosynthesis because of its rich carbon content. This work demonstrated the ability of R. equi for PHB production, evaluated its content using GC, and characterized the extracted polymer by studying the chemical structure and thermal properties of this homopolymer PHB in comparison with the results of other studies. The obtained PHB in this study has a molecular weight with a polydispersity value, which can be used as a substitute for synthetic polymers. Thus, it can be used in commercial applications.
Acknowledgement
The authors gratefully acknowledge the Al-Nahrain University and the Ecobiomaterial Lab, Biological School, University of Science, Malaysia.
Notes
Peer review under responsibility of Taibah University.
References
- C.S.K.ReddyR.GhaiR.KaliaPolyhydroxyalkanoates: an overviewBioresour. Technol.782003137146
- S.J.ParkM.K.KimS.Y.LeeS.C.LimAdvanced bacterial polyhydroxyalkanoates: towards a versatile and sustainable platform for unnatural tailor-made polyestersBiotechnol. Adv.30201211961206
- M.ZinnR.HanyTailored material properties of polyhydroxyalkanoates through biosynthesis and chemical modificationAdv. Eng. Mater.72005408411
- J.LuR.C.TappelC.T.NomuraMini-review: biosynthesis of poly(hydroxyalkanoates)Polym. Rev.492009226248
- E.A.DawesP.J.SeniorThe role and regulation of energy reserve polymer in microorganismsAdv. Microb. Physiol.101973135265
- Y.DioMicrobial Polyester1990VCHNew York
- E.A.DawesPolyhydroxybutyrate: an intriguing biopolymerBiosci. Rep.861988537547
- U.AyselN.SahinY.BeyatleAccumulation of poly-β-hydroxybutyrate in streptomyces species during growth with different nitrogen sourcesTurk. J. Biol.262002171174
- P.ChakrabortyW.GibbonsK.MuthukmarappanlConversion of volatile fatty acids into polyhydroxyalkanoates by Ralstonia eutrophaJ. Appl. Microbiol.106200919962005
- I.P.CastroD.BingerA.RodriguesIn-silico-driven metabolic engineering of Pseudomonas putida for enhanced production of poly-hydroxyalkanoatesMetab. Eng.152013113123
- G.SathiyanarayananG.SaibabaG.S.KiranJ.SelvinA statistical approach for optimization of polyhydroxybutyrate by marine Bacillus subtilis MSBN17Int. J. Biol. Macromol.592013170177
- K.HoriM.AbeH.UnnoProduction of triacylglycerol and poly(3-hydroxybutyrate-co-3-hydroxyvalerata) by the toluene-degrading bacterium Rhodococcus aetherivorans IAR1J. Biosci. Bioeng.10842009319324
- C.F.BuddeS.L.RiedelL.B.WillisC.RhaA.J.SinskeyProduction of poly(3-hydroxybutyrate-co-3-hydroxyhexanoate) from plant oil by engineered Ralstonia eutropha strainsAppl. Environ. Microbiol.779201128472854
- J.W.HolderJ.C.UlrichA.C.DeBonoP.A.GodfreyC.A.DesjardinsJ.ZuckerQ.ZengA.L.B.LeachI.GhivirigaC.DancelT.AbeelComparative and functional genomics of Rhodococcus opacus PD630 for biofuels developmentPLoS Genet.792011118
- M.AkiyamaT.TsugeY.DoiEnvironmental life cycle comparison of polyhydroxyalkanoates produced from renewable carbon resources by bacterial fermentationPolym. Degrad. Stab.802003183194
- Y.BasinonPalm oil production through sustainable plantationsEur. J. Lipid Sci. Technol.10942007289295
- C.S.HaW.J.ChoMiscibility, properties and biodegradability of microbial polyester containing blendsProg. Polym. Sci.272002759809
- M.Yamaguchik.ArakawaEffect of thermal degradation on rheological properties for poly(3-hydroxybutyrate)Eur. Polym. J.427200614791486
- S.G.HongC.H.LinImprovement of thermal properties of polyhydroxybutyrate by grafting chemicalsE-Polymers1012010509522
- P.PersicoV.AbrogiA.BaroniEnhancement of poly(hydroxybutyrate) thermal and processing stability using a bio-waste derived additiveInt. J. Biol. Macromol.51201211511158
- Y.DoiS.KitamuraH.AbeMicrobial synthesis and characterization of poly(3-hydroxybutyrate-co-3-hydroxyhexanoate)Macromolecules28199548224828
- H.KamilahT.TsugeT.A.YangK.SudeshWaste cooking oil as substrate for biosynthesis of poly(3-hydroxybutyrate) and poly(3-hydroxybutyrate-co-3-hydroxyhexanoate): turning waste into a value-added productMalays. J. Microbiol.9120135159
- G.BrauneggB.SonnleitnerR.M.LaffertyA rapid gas chromatographic method for the determination of poly-β-hydroxybutyric acid in microbial biomassEur. J. Appl. Microbiol. Biotechnol.619782937
- K.SudeshL.L.FewM.A.Mohd NoorM.I.Abdul MajidM.R.SamianN.NajimudinBiosynthesis and characterization of polyhydroxyalkanoate blends accumulated by Pseudomonas sp USM 4-55J. Biosci.1520041528
- K.SudeshPolyhydroxyalkanoates from Palm Oil: Biodegradable Plastics2013 Springer, Germany
- U.PieperA.SteinbiichelIdentification, cloning and sequence analysis of the poly(3-hydroxyalkanoic acid) synthase gene of Gram-positive bacterium Rhodococcus rubberFEMS Microbiol. Lett.9619927380
- Y.M.WongC.J.BrighamC.RhaA.J.SinskeyBiosynthesis and characterization of polyhydroxyalkanoate containing high 3-hydroxyhexanoate monomer fraction from crude palm kernel oil by recombinant Cupriavidus necatorBioresour. Technol.1122012320327
- Y.K.KekC.W.ChangA.A.AmirulK.SudeshHeterologous expression of Cupriavidus sp USMAA2-4 PHA synthase gene in PHB–4 mutant for the production of poly(3-hydroxybutyrate) and its copolymersWorld J. Microbiol. Biotechnol.26201015951603
- K.M.BonthroneJ.ClaussD.M.HorowitzB.K.HunterJ.K.M.SandersThe biological and physical chemistry of polyhydroxyalkanoates as seen by NMR spectroscopyFEMS Microbiol. Rev.1031992269278
- S.JanC.RoblotJ.CourtoisB.CourtoisJ.N.BarbotinJ.P.Shguin1H NMR spectroscopic determination of poly 3-hydroxybutyrate extracted from microbial biomassEnzyme Microbiol. Technol.181996195201
- C.O.FabianeL.D.MarcosR.C.LedaM.G.F.DeniseCharacterization of poly(3hydroxybutyrate) produced by Cupriavidis necator in solid state fermentationBioresour. Technol.982007633638
- M.ErcegT.KovaCiCI.KlariCThermal degradation of poly(3hydroxybutyrate) plasticized with acetyl tributyl citratePolym. Degrad. Stab.902005313318
- D.MarjadiN.DharaiyaRecovery and characterization of poly(3-hydroxybutyric acid) synthesized in Staphylococcus epidermidisAfr. J. Environ. Sci. Technol.862014319329
- M.ScandolaM.PizzoliG.CeccoruiliA.CesaroS.PaolettiL.NavariniViscoelastic and thermal properties of bacterial poly(d-(−)-β-hydroxybutyrate)Int. J. Biol. Macromol.101988373377
- R.SindhuB.AmmuP.BinodS.K.DeepthiK.B.RamachandranC.R.SoccolA.PandeyProduction and characterization of poly-3-hydroxybutyrate from crude glycerol by Bacillus sphaericus NII 0838 and improving its thermal properties by blending with other polymersBraz. Arch. Biol. Technol.5442011783794
- J.Y.L.ChoiJ.K.LeeY.YouW.H.ParkEpoxidized polybutadiene as a thermal stabilizer for poly(3-hydroxybutyrate). II. Thermal stabilization of poly(3-hydroxybutyrate) by epoxidized polybutadieneFibers Polym.42003195198
- L.MartinoM.V.CruzA.ScomaF.FreitasL.BertinM.ScandolaM.A.M.ReisRecovery of amorphous polyhydroxybutyrate granules from Cupriavidus necator cells grown on used cooking oilInt. J. Biol. Macromol.712014117123
- A.J.AndersonE.A.DawesOccurence, metabolic roles and industrial uses of bacterial polyhydroxybutyratesAppl. Environ. Microbiol.541990450472
- B.H.A.RehmPolyester synthases: natural catalysts for plasticsBiochem. J.37620031533
- S.ChaijamrusN.UdpuayProduction and characterization of polyhydroxybutyrate from molasses and corn steep liquor produced by Bacillus megaterium ATCC 6748Agric. Eng. Int.102008112
- S.O.KulkarniP.P.KanekarJ.P.JogS.S.SarnaikS.S.NilegaonkarProduction of copolymer, poly(hydroxybutyrate-co-hydroxyvalerate) by Halomonas campisalis MCM B-1027 using agro-wastesInt. J. Biol. Macromol.722015784789